 Creative Education 2012. Vol.3, No.5, 619-631 Published Online September 2012 in SciRes (http://www.SciRP.org/journal/ce) http://dx.doi.org/10.4236/ce.2012.35091 Copyright © 2012 SciRes. 619 Addressing Misconceptions about the Particulate Nature of Matter among Secondary-School and High-School Students in the Republic of Macedonia Marina I. Stojanovska1, Bojan T. Šoptrajanov2, Vladimir M. Petruševski1 1Institute of Chemistry, Faculty of Natural Sciences and Mathematics, Ss. Cyril & Methodius University, Skopje, Macedonia 2Macedonian Academy of Sciences and Arts, Skopje, Macedonia Email: marinam@pmf.ukim.mk Received July 3rd, 2012; revised August 10th, 2012; accepted August 20th, 2012 A study was conducted to identify concepts about the particulate nature of matter among secondary- and high-school students (N = 187) and to address some misconceptions regarding this topic, especially the misunderstandings related to the vague ideas of the relationship between the macro and micro world. Data were collected using both quantitative (six-item multiple-choice instrument in a pre-test-post-test design) and qualitative (semi-structured focus group interviews) methods. Paired-samples t-test analysis showed that students experienced significantly higher results in the post-test when compared to the pre-test, thus confirming the efficiency of the intervention program in facilitating the understanding of some basic ele- ments of the theory and practice concerning the particulate nature of matter (widely known as particle theory concepts, a term which will be used in this paper as well) among students of different levels of study. The findings revealed seven misconceptions prevalent by more than 20% of students and some ad- ditional ones emerged from the in-depth focus group discussions. The analysis of the content of textbooks indicated that some erroneous chemical concepts might have been formed as a result of the teaching of chemistry and that of physics, as well. The use of animations and molecular models had a positive effect on students and pointed to the need of introducing, in the chemistry teaching, the new material more visu- ally. Keywords: Focus Group Interviews; Intervention Program; Misconceptions; Particle Theory Concepts; Particulate Nature of Matter; Secondary- and High-School Chemistry Education Introduction Chemistry is a subject based on concepts, many of which are abstract and are therefore hard to grasp and learn especially when the students are put in a position to believe without seeing. On the other hand, students are basically familiar with a num- ber of relevant concepts as a result of their previous learning (Roschelle, 1995). The potentially present preconceptions about the world itself can be reflected in the chemistry lessons and can sometimes grow into misconceptions. It has been known that the misconceptions are powerful, ex- tremely persistent and hard to change, creating obstacles to further learning (Canpolat, 2006; Pabuçcu & Geban, 2006). Many misconceptions concerning various chemistry (and sci- ence in general) topics have been documented (Horton, 2007; Kind, 2004). Also, many studies in the field of science miscon- ceptions and difficulties in learning and understanding chemical concepts have been reported (Chiu, 2005; Cliff, 2009; Çalýk et al., 2005; Kariper, 2011; Levy Nahum et al., 2004; Morgіl & Yörük, 2006; Taber, 2011; Wenning, 2008). In some of them, students’ drawings or pictorial representations within the test item play an important role in inspecting their thinking (Mul- ford & Robinson, 2002; Nyachwaya et al., 2011; Onwu & Randal, 2006). The misconceptions related to the particulate nature of matter are perhaps some of the most investigated ones and are widely reported in literature (Badrian et al., 2011; Banda et al., 2011; Bindernagel & Eilks, 2009; de Vos & Ver- donk, 1996; Bridle & Yezierski, 2011; Gabel & Samuel, 1987; Margel et al., 2004; Özmen & Kenan, 2007; Skamp, 2009; Yezierski & Birk, 2006). Many misconceptions are due to the fact that students do not distinguish between macroscopic and microscopic explanations (Bucat, 2004; Chandrasegaran et al., 2007; Meijer, 2011). According to Johnstone (2000), the chemical knowledge is acquired at three levels: a) the macro and tangible (what can be seen, touched and smelt); b) the sub-micro (atoms, molecules, ions and structures); and c) the representational (symbols, for- mulae, equations, mathematical manipulation, graphs etc.) and the links between these levels should be explicitly taught. None of the above aspects is superior to another; on the contrary, they complement each other. Aware of the conflict between the in- tuitive, continuous model and the “scientific”, particulate one, Ben-Zvi et al. (1986) pose the question: “Is an atom of copper malleable?” In their study, involving students from all levels, Treagust et al. (2011) display the widely-held confusion be- tween the macroscopic and sub-microscopic properties of mat- ter held by the students. Namely, the authors highlight the ideas of students that particles (sub-microscopic representation) themselves are compressed and pushed closer together instead of the gas being compressed (macroscopic representation). Introducing all three levels to students simultaneously leads to an “overload of their working memory space” (Sirhan, 2007)
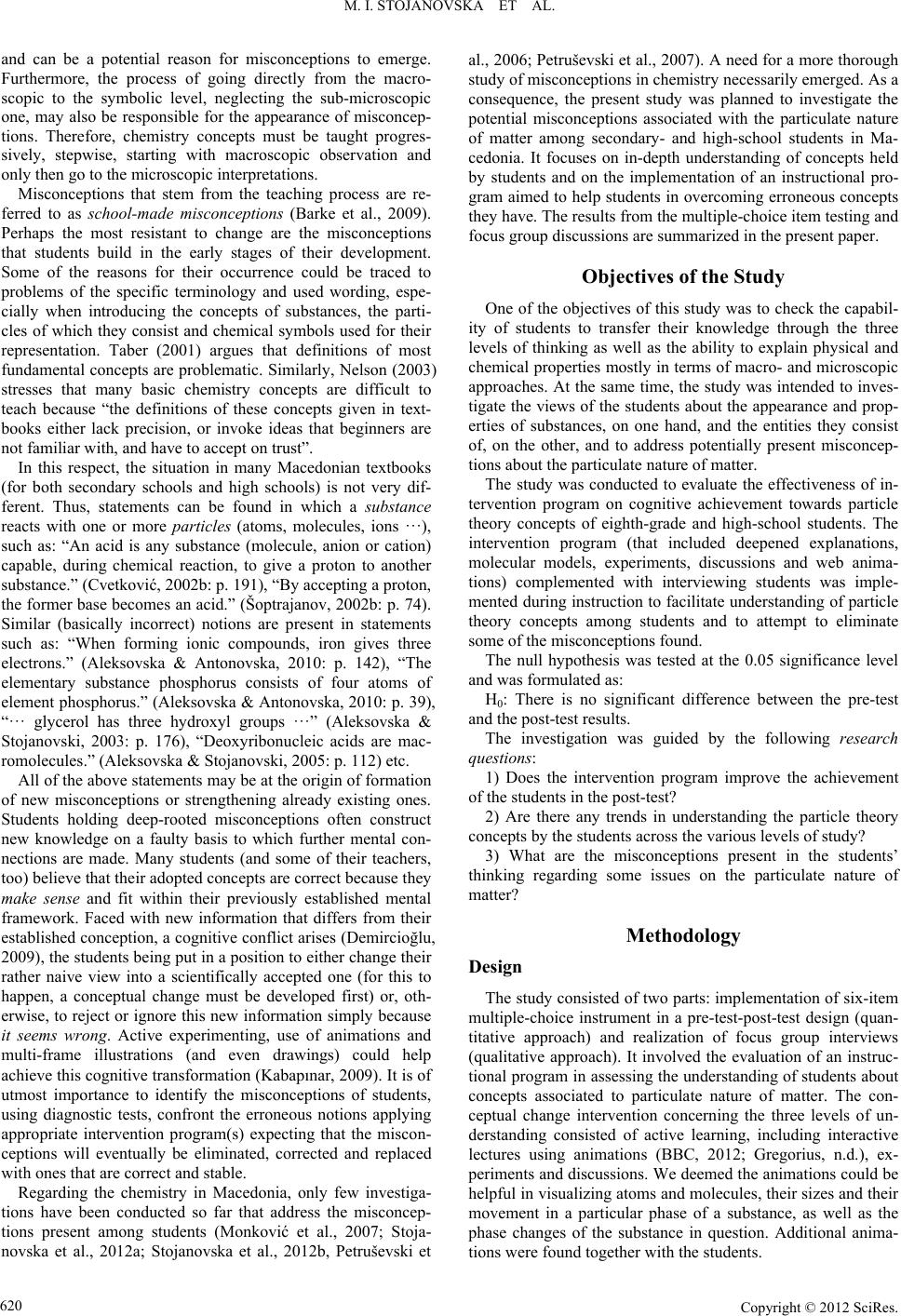 M. I. STOJANOVSKA ET AL. and can be a potential reason for misconceptions to emerge. Furthermore, the process of going directly from the macro- scopic to the symbolic level, neglecting the sub-microscopic one, may also be responsible for the appearance of misconcep- tions. Therefore, chemistry concepts must be taught progres- sively, stepwise, starting with macroscopic observation and only then go to the microscopic interpretations. Misconceptions that stem from the teaching process are re- ferred to as school-made misconceptions (Barke et al., 2009). Perhaps the most resistant to change are the misconceptions that students build in the early stages of their development. Some of the reasons for their occurrence could be traced to problems of the specific terminology and used wording, espe- cially when introducing the concepts of substances, the parti- cles of which they consist and chemical symbols used for their representation. Taber (2001) argues that definitions of most fundamental concepts are problematic. Similarly, Nelson (2003) stresses that many basic chemistry concepts are difficult to teach because “the definitions of these concepts given in text- books either lack precision, or invoke ideas that beginners are not familiar with, and have to accept on trust”. In this respect, the situation in many Macedonian textbooks (for both secondary schools and high schools) is not very dif- ferent. Thus, statements can be found in which a substance reacts with one or more particles (atoms, molecules, ions ···), such as: “An acid is any substance (molecule, anion or cation) capable, during chemical reaction, to give a proton to another substance.” (Cvetković, 2002b: p. 191), “By accepting a proton, the former base becomes an acid.” (Šoptrajanov, 2002b: p. 74). Similar (basically incorrect) notions are present in statements such as: “When forming ionic compounds, iron gives three electrons.” (Aleksovska & Antonovska, 2010: p. 142), “The elementary substance phosphorus consists of four atoms of element phosphorus.” (Aleksovska & Antonovska, 2010: p. 39), “··· glycerol has three hydroxyl groups ···” (Aleksovska & Stojanovski, 2003: p. 176), “Deoxyribonucleic acids are mac- romolecules.” (Aleksovska & Stojanovski, 2005: p. 112) etc. All of the above statements may be at the origin of formation of new misconceptions or strengthening already existing ones. Students holding deep-rooted misconceptions often construct new knowledge on a faulty basis to which further mental con- nections are made. Many students (and some of their teachers, too) believe that their adopted concepts are correct because they make sense and fit within their previously established mental framework. Faced with new information that differs from their established conception, a cognitive conflict arises (Demircioğlu, 2009), the students being put in a position to either change their rather naive view into a scientifically accepted one (for this to happen, a conceptual change must be developed first) or, oth- erwise, to reject or ignore this new information simply because it seems wrong. Active experimenting, use of animations and multi-frame illustrations (and even drawings) could help achieve this cognitive transformation (Kabapınar, 2009). It is of utmost importance to identify the misconceptions of students, using diagnostic tests, confront the erroneous notions applying appropriate intervention program(s) expecting that the miscon- ceptions will eventually be eliminated, corrected and replaced with ones that are correct and stable. Regarding the chemistry in Macedonia, only few investiga- tions have been conducted so far that address the misconcep- tions present among students (Monković et al., 2007; Stoja- novska et al., 2012a; Stojanovska et al., 2012b, Petruševski et al., 2006; Petruševski et al., 2007). A need for a more thorough study of misconceptions in chemistry necessarily emerged. As a consequence, the present study was planned to investigate the potential misconceptions associated with the particulate nature of matter among secondary- and high-school students in Ma- cedonia. It focuses on in-depth understanding of concepts held by students and on the implementation of an instructional pro- gram aimed to help students in overcoming erroneous concepts they have. The results from the multiple-choice item testing and focus group discussions are summarized in the present paper. Objectives of the Study One of the objectives of this study was to check the capabil- ity of students to transfer their knowledge through the three levels of thinking as well as the ability to explain physical and chemical properties mostly in terms of macro- and microscopic approaches. At the same time, the study was intended to inves- tigate the views of the students about the appearance and prop- erties of substances, on one hand, and the entities they consist of, on the other, and to address potentially present misconcep- tions about the particulate nature of matter. The study was conducted to evaluate the effectiveness of in- tervention program on cognitive achievement towards particle theory concepts of eighth-grade and high-school students. The intervention program (that included deepened explanations, molecular models, experiments, discussions and web anima- tions) complemented with interviewing students was imple- mented during instruction to facilitate understanding of particle theory concepts among students and to attempt to eliminate some of the misconceptions found. The null hypothesis was tested at the 0.05 significance level and was formulated as: H0: There is no significant difference between the pre-test and the post-test results. The investigation was guided by the following research questions: 1) Does the intervention program improve the achievement of the students in the post-test? 2) Are there any trends in understanding the particle theory concepts by the students across the various levels of study? 3) What are the misconceptions present in the students’ thinking regarding some issues on the particulate nature of matter? Methodology Design The study consisted of two parts: implementation of six-item multiple-choice instrument in a pre-test-post-test design (quan- titative approach) and realization of focus group interviews (qualitative approach). It involved the evaluation of an instruc- tional program in assessing the understanding of students about concepts associated to particulate nature of matter. The con- ceptual change intervention concerning the three levels of un- derstanding consisted of active learning, including interactive lectures using animations (BBC, 2012; Gregorius, n.d.), ex- periments and discussions. We deemed the animations could be helpful in visualizing atoms and molecules, their sizes and their movement in a particular phase of a substance, as well as the phase changes of the substance in question. Additional anima- tions were found together with the students. Copyright © 2012 SciRes. 620
 M. I. STOJANOVSKA ET AL. Copyright © 2012 SciRes. 621 The experiments carried out in front of (or together with) the students followed by discussions were: a) Freezing of water in a plastic cup and discussing phase changes from the macro and micro points of view; b) Burning of ethanol and discussing the fate of the C, H and O atoms and; c) Dissolving anhydrous copper(II) sulfate in water, evapo- rating the solvent and comparing the chemical nature of the substance(s) before and after the change. The developmental stages of the investigation are briefly stated as follows: 1) Administration of the pre-test; 2) Analysis of the pre-test data using the software package PASW 18.0; 3) Recording misconceptions and identifying students hold- ing those misconceptions; 4) Conducting focus group interviews; 5) Preparing transcripts and analyzing each interview; 6) Implementation of instruction program; 7) Administration of the post-test (two weeks after finishing the stage 6); 8) Analysis of the post-test data using the software package PASW 18.0. Sample The instrument was administered to a total of 187 sample students both from a secondary school (N = 30) and from sev- eral high schools (N = 157) in the 2011/12 school year. Further, in order to get an insight into the particle theory concepts among students of different age and the related misconceptions, four sub-samples were formed according to the level of study in which students were enrolled. Regarding the sampling, it is mentioned (Hoque et al., 2011) that the minimum number of subjects for experimental research is 30. Thus, the number of students involved in this study was sufficient for further analy- sis. A total of 77 participants were purposively selected from the sample students to take part in the group discussions. Accord- ing to their earlier performance in chemistry, students were categorized into two sub-groups (high achievers and low achievers). Details concerning the participants involved in the study are given in Table 1. During the focus group interviews, mutual respect and con- fidentiality was observed. The participants (students) were carefully explained that the test results and interview discus- sions would be used for research purposes only and that all information would be kept confidential. For that reason, each student was assigned a code (S1, S2 etc.) in the data analysis process. Teachers approved the testing and interviewing stu- dents. Students who agreed to participate in focus group dis- cussions had the right to withdraw at any given moment and were guaranteed that the research will not affect their grade. Data Collection A combination of quantitative and qualitative data collection techniques was used and two kinds of instruments were imple- mented: multiple-choice concept tests and focus group inter- views. The usage of both kinds of methods led to an improve- ment of the validity of results by means of triangulation (Hus- sein, 2009; Jick, 1979). The advantages of the written test are that it is easily administered to a wider population and that it allows calculating the percentages of correct and incorrect an- swers (Schmidt et al., 2003), thus enabling to draw conclusions about the most prevalent choices that can indicate existence of a misconception. Nevertheless, in many cases students who hold misconceptions were able to give correct answers to open- ended questions, especially to those that do not seek higher mental activation. Therefore, it is advisable to use misconcep- tions as distractors in a multiple-choice test (Herrmann Abell & DeBoer, 2011) and, in that way, to provide students with plau- sible answer choices to select from, so they are less likely to simply guess the correct answer. Furthermore, interviews are an appropriate technique when looking for in-depth explanations. Interviews, both individual (Singh, 2008; Sözbilir et al., 2010) and in a group (Eybe & Schmidt, 2004; Muzaffar et al., 2011; Nair & Ngang, 2012; Osborne & Collins, 2001) have been successfully used as data collection instruments in educational studies, the focus groups providing a possibility for interaction and exchanging ideas among participants. Concept test: To identify notions that are present and to get insight into the misconceptions regarding the particulate nature of matter, a concept test was given both before and after group discussions and intervention program (these are referred to as pre-test and post-test respectively). The test was distributed to secondary- and high-school students (Appendix 1). It was conducted under normal class conditions and was completed within 40 minutes. It consisted of 6 items written in a multi- ple-choice format. No open-ended questions were provided for testing because students (a part of them) were later interviewed in a group, although occasionally an option existed to write down their answers if the participants thought this would be more appropriate. The test items were developed by the authors. Some of the misconceptions known in the literature were added as distractors (Horton, 2007; Kind, 2004; Anonimous, 2003; Yezierski & Birk, 2006). Focus group interviews: In this study, thirteen semi-struc- Table 1. Information on participants involved in the study. Number of participants Number of focus groups Level of study N (%) High-achievers Low-achievers Secondary school VIII Grade 30 (16) 2 1 II Year 57 (31) 1 1 III Year 51 (27) 2 2 IV Year 49 (26) 2 2 High school Total 187 (100) 13
 M. I. STOJANOVSKA ET AL. tured in-depth focus group interviews were conducted: three with secondary-school and ten with high-school students. The number of students in each group varied between 5 and 7 and the time needed for completing an interview was in the range of 40 to 90 minutes. The interviews took place in an empty class- room (or a laboratory) to enable uninterrupted conversation. They were audio-taped and transcripts were made for each focus group discussion. The interviews were carried out according to the design pro- posed by Kvale (1996). They consisted of three phases: the briefing phase, the main phase and the debriefing phase. In the main phase students were asked questions (in an open-ended format) according to an interview guide prepared beforehand. Details about the interview protocol are given in the Appendix 2. The misconceptions identified from the tests were used as a starting point in the preparation of the interview guide and conducting the interviews themselves. More questions emerged during discussions depending on the answers of the partici- pants. Data analysis In the concept test, each correct response was scored 1 point and each incorrect one - 0 points, thus making the maximum possible score on the test 6 points. Next, responses were ana- lyzed using the Predictive Analytics Software PASW 18.0 and were subjected to mean, standard deviations and significance testing. In order to determine whether significance differences were present between the pre- and post-test, a pared-sample t-test was run. Besides calculating the percentage of correct answers, the number of wrong ones that were present in the answers by ap- proximately 20% or above was considered. Namely, according to Dhindsa & Treagust (2009), the incorrect responses (distrac- tors) by more than 20% of the students indicate the presence of misconception of the tested concepts. The above-mentioned authors also proposed a criterion for satisfactory understanding the tested concept in a four-distractors-item, according to which it is necessary that at least 75% of the students give correct answers. In the present study, students preferred one or two distractors for certain items. The misconceptions that were identified in the answers of over 20% of the students are reported in the Results section. The focus group interviews were not subject to a de- tailed analysis and are used as representative for misconcep- tions found in the written responses of the students. All inter- views were conducted in Macedonian, so the excerpts quoted in this paper were translated into English. Results The first research question was related to the effectiveness of the intervention program on the improvement of the students’ achievement in the post-test. The comparison of the mean scores of pre- and post-tests showed that an improvement in cognitive achievement was evident (Figure 1), the positive results being found for all sub-samples in the study. The results of the paired-samples t-test analysis showed that that students experienced significantly higher results in the post-test when compared to the pre-test in each of the four sub-samples and in the total sample (Table 2) which indicates that the intervention program was efficient in improving the students’ knowledge and understanding of particle theory concepts. Thus, the null hypothesis was rejected. In addition, the effect size (Cohen’s d) was calculated as a measure of strength of the difference between the pre- and post-test mean scores. The effect size values in Table 2 are high (d > 0.8) implying relatively large mean differences for each sub-sample and for the total sample, as well. With respect to the second research question for the trends in understanding of particle theory concepts by students across the various levels of study, one can notice that the t-values increase from eighth-grade to the fourth year high-school students’ sam- ples; the second value disagrees with this trend by being higher (cf. Table 2). As a rough estimate of the concept-grasping by the students, the percentage of correct answers (both in the pre-test and the post-test) for all test items are given in the Table 3. The data summarized in this table show that in all cases but one (for eighth-grade students), an improvement in understanding can be observed. Students had problems solving items 1, 4 and 6 and understanding the concepts involved, but were more suc- cessful at answering the other items in the pre-test. Figure 1. Comparison of the pre-test—post-test mean scores. Table 2. Paired-samples t-test analysis results comparing pre-test and post-test score. Pre-test Post-test Level of study N Mean SD Mean SD t Cohen’s d VIII 30 1.38 0.84 2.95 0.63 9.88* 2.11 II 57 1.29 0.97 4.32 1.44 14.60* 2.47 III 51 2.27 1.47 4.06 1.19 10.43* 1.34 IV 49 2.1 1.25 3.76 1.05 11.00* 1.44 Total 187 1.78 1.25 3.88 1.25 20.58* 1.68 *p < 0.01. Copyright © 2012 SciRes. 622
 M. I. STOJANOVSKA ET AL. As for the third research question, some distractors were fa- voured for certain items in the test and thus seven misconcep- tions were identified in the answers given by students. Several more misconceptions emerged on analyzing the interview tran- scripts. The misconceptions found in the written responses are listed here and are discussed in more detail in the next section. Percentages of students holding the particular misconceptions were also calculated (according the level of study, as well as the total sample percentage) and are summarized in the Table 4. No strict regularity in their appearance in different sub-sample students can be detected from the percentages of the prevalent misconception represented in the table. Misconceptions (M) identified through the written responses of the students were: M1: Butadiene reacts with two bromines. M2: Butadiene reacts with one molecule of bromine. M3: Copper(II) sulfate from aqueous solution crystallizes as a solid substance composed of copper(II) sulfate molecules. M4: In a process of ice melting, the volume of the system (mixture of ice and liquid water) increases. M5: Particles change from solid to liquid ones. M6: When ethanol is ignited, carbon, hydrogen and oxygen atoms (of which ethanol is composed), will ignite too and will burn just like the ethanol. M7: The particles of a substance enlarge during heating that substance. Three more misconceptions were detected in the test answers of certain sub-samples. Thus, among eighth-grade students, a notion that particles receive heat and enlarge was prevalent. In the pre-test, 33.3% of students have chosen this option. The percentage was not much reduced in the post-test (26.7%), underlining the fact that misconceptions are very resistant and hard to change. Two other misconceptions were observed in the second-year high-school sub-sample analysis. A statement commonly present among such students was that carbon, hy- drogen and oxygen atoms (of which ethanol is composed), will disappear when ethanol is ignited (31.6% in the pre-test and 7.0% in the post-test). Another favored discractor that repre- sented a misconception was the one about particles of a sub- stance shrinking during heating that substance. The percentage of students that held this erroneous notion prior to instruction was 28.1% and it was decreased to a great extent after the in- struction (3.5% of students have chosen this option in the post-test). Discussion The overall findings resulting from this research were posi- tive and suggested that the intervention program effectively contributed to the improvement in the knowledge of students and their understanding of particle theory concepts. The con- siderable increase in the post-test scores evidently supports this observation. However, several misconceptions were still pre- sent in the post-test. Some students, perhaps due to their poor understanding, might have been misled by the distractors. Mis- leading role of distractors was, presumably, the most pro- nounced in the eighth-grade sample, whose participants (by being the youngest) have limited knowledge. Also, beginners in learning chemistry might not have well developed chemistry concepts nor experience in chemistry and this could be assigned to the constant level of misconception for certain test items for eighth graders. Such was the case for the misconceptions re- ferred as M1 and M2. These were distractors for the same test item. It is likely students who were doubtful in answering this test item have preferred the M1 in the post-test instead of their Table 3. Percentage of correct responses of students on pre- and post-test. VIII II III IV Total Item No. Pre Post Pre Post Pre Post Pre Post Pre Post 1 10 13.3 8.8 47.4 0 51 0 49 4.3 43.3 2 63.3 96.7 29.8 82.5 52.9 92.2 44.9 89.8 45.5 89.3 3 53.3 16.7 40.4 80.7 52.9 66.7 40.8 42.9 46 56.7 4 10 26.7 3.5 56.1 5.9 31.4 8.2 42.9 6.4 41.2 5 23.3 80 29.8 71.9 76.5 88.2 73.5 95.9 52.9 84 6 10 83.3 15.8 87.7 47.1 72.5 40.8 55.1 29.9 74.3 Table 4. Percentage of misconceptions present in each of the sub-samples and the total sample. VIII II III IV Total Misconception No. Pre Post Pre Post Pre Post Pre Post Pre Post M1 36.7 30 54.4 15.8 15.7 19.6 2 22.4 27.3 20.9 M2 40 56.7 29.8 28.1 72.5 27.5 98 28.6 61 32.6 M3 26.7 3.3 49.1 5.3 19.6 3.9 40.8 6.1 35.3 4.8 M4 40 83.3 42.1 12.3 31.4 15.7 44.9 26.5 39.6 28.3 M5 33.3 30 49.1 19.3 43.1 13.7 69.4 22.4 50.3 20.3 M6 36.7 10 31.6 17.5 21.6 9.8 18.4 0 26.2 9.6 M7 76.7 6.7 49.1 5.3 35.3 15.7 51 32.7 50.3 15.5 Copyright © 2012 SciRes. 623
 M. I. STOJANOVSKA ET AL. previously chosen option in the pre-test (M2) or the correct answer (option “c”). Furthermore, data in Table 4 indicate that about 28% of the third- and the fourth-year students showed consistency in their answer regarding the first item (unfortu- nately, the wrong one) by choosing the M2 in the post-test. On the other hand, approximately 50% of each of these sub-sample students (cf. Table 3) experienced positive gain in respect to the tested concept on the first item (note that the percentage of correct responses for the third- and the fourth-year students is 0.0% in the pre-test), thus reducing the level of both M1 and M2 in the post-test. This was not the case with the eighth-grade students. Another observation is interesting to note at this point. It was perceived that percent distribution for chosen test options was more or less equally represented for eighth-grade students, thus lowering the percent of correct answer for account of other distractors (which were potential misconceptions). To justify this fact two examples are pointed out. It was mentioned in the Result Section that 33.3% of eighth-grade students in the pre- test and 26.7% in the post-test have chosen the option that par- ticles receive heat and enlarge (option “f” in the fourth test item). The percent of chosen option “f” was 3.9% and 0.0% for the third-year students and 2.0% and 0.0% for the fourth-year ones in the pre- and post-test respectively. This could explain the more pronounced level of M5 in the pre-test for students enrolled in higher grades. The second example refers to the first test item. It was noticed that 10.0% of eighth-grade students thought that the statement “one gram butadiene reacts with one gram bromine” correctly describes the equation. None of the third- or the fourth-year students has chosen this option. It is fair to mention that, when dealing with multiple-choice items, students have an opportunity to guess the correct answer or, on the contrary, to be misled by the distractors. For this reason, the interviews were an important part of the research. During the focus group discussions, students tried to explain the statements they have chosen (or written) and, thus, helped the researchers involved in this investigation to understand the reasoning behind their opinions. Excerpts from the interview transcripts that are presented in the paper could enable readers to examine the trustworthiness of the research procedure. The warm-up questions in the focus group discussions were aimed at the (mental) images that students had about atoms and molecules. On the basis of the experience in the microscopic world, an opinion that atoms and molecules are spherical pre- vailed as most of students recalled the illustrations they have seen in textbooks or models that they have been using earlier. Further, considering the phase changes, some students re- ferred to molecular distances and molecular forces as factors responsible for the change. Unfortunately, notions that mole- cules of liquid (or gaseous) water weigh less that the molecules of ice (or liquid water), that molecules can expand and stretch when a substance is heated and that the particles (atoms and molecules) can change their shape seem to be widespread even among students that do not fall in the category of low achievers. Here, an emphasis is given to those misconceptions found in the written responses of students that were represented by more than 20% (the list of such misconceptions was given in the Results Section but in this part they are reviewed in more de- tail). In addition, excerpts from the conversations between the researcher (R) and student (S) are given to support the findings from the test analysis. After every excerpt a brief description is given that includes the type of school (SS or HS for secondary and high school respectively), the level of education within the school in question (given by Roman numerals) and the sub- group to which the student belongs. M1 and M2: Butadiene reacts with two bromines or with one molecule of bromine. These two misconceptions were among the most persistent ones. The corresponding distractors were present with more than 20% in the pre-test (see Table 4) and even in the post-test, although the percentage of students who gave such answers decreased from 27.3 to 20.9% for the first and from 61.0 to 32.6% for the second option. Therefore, they can be considered to reflect potential misconceptions (M1 and M2). Although some improvement was obvious, the percentage of students holding erroneous views was still high, pointing once again to the general property of misconceptions: lots of efforts are needed to eliminate them and to engrave correct knowledge in the minds of the students. During the focus group discussion, students, for example, explained: “I have calculated correctly: there are two atoms of bromine and that is why butadiene reacts with two atoms of bromine.” (SS-VIII-high achievers) or “I considered that Br2 is one molecule of bromine, so my answer is that butadiene reacts with one molecule of bromine.” (HS-II-low achievers) These excerpts clearly point out to the vagueness of the ideas of students and their inability to properly use the macro and micro concepts and the corresponding terminology. Surely, a substance can not react with only one or a few particles. M3: Copper(II) sulfate from aqueous solution crystallizes as a solid substance composed of copper(II) sulfate mole- cules. The second item of the test covers the concept of crystalliza- tion of copper(II) sulfate from aqueous solution and the offered options were: with five molecules of water; as a solid substance composed of copper(II) sulfate molecules; as а pentahydrate; as an anhydrous salt. We assumed that the first distractоr would be an option of choice for the majority of students, but, instead, it turned out that such was the case with the second distractor (M3). The first option was chosen by less than 20% of the stu- dents in the pre-test and the second one with 35.3%. This indi- cates that most of the students have heard about crystalline hydrates and can recognize the formula of blue vitriol, thus connecting it with the term pentahydrate. Sometimes erroneous notions are not perceptible right away and can be discovered only when looking at the in-depth re- sponses. The next dialog is representative of that. R: “S12, what is your opinion? You have answered that copper(II) sulphate crystallizes as pentahydrate from water solutions. Why do you think this is the correct answer?” S12: “Last year we learned that ··· er ··· copper sulphate has five water molecules.” R: “Where? Five water molecules in ···?” S12: “In its structure.” (SS-VIII-high achievers) Discussing within certain groups, some students claimed that CuSO4·5H2O can be formed if five drops of water are added to CuSO4. It seems likely that for these students five drops are equivalent to five molecules (or five moles, perhaps?). In rela- tion to this, Tasker (1998) warns about misleading molecular animations available as CD supplements to some textbooks, thus generating deeply embedded misconceptions such as the one that assert presence of only a few ionic species in a drop of water. The presence of another very important misconception be- Copyright © 2012 SciRes. 624
 M. I. STOJANOVSKA ET AL. comes apparent from the second item analysis. Namely, the above mentioned high value of 35.3% strongly indicates that students fail to distinguish ionic from covalent substances, so they refer to all building particles as molecules. It seems that this type of misconception is widely spread. R: “Do you think that molecules of copper(II) sulfate exist?” S10: “Yes.” R: “Could you explain in more detail what do you mean?” S10: “I don’t know ··· I’m not sure.” S9: “I think that molecules of copper(II) sulfate do not exist because copper (II) sulfate is a compound.” R: “What about water? Is water a compound?” S9: “Well ··· yes.” R: “Does it consist of molecules?” S9: “Yes, it does.” R: “When is it right to say “molecules”?” S12: “When we talk about ions, it must be an ionic bonded compound.” (HS-II-high achievers) One possible reason for such a wrong concept can be found in some textbooks (Cvetković, 2002b: p. 33) where the author represents structural formulae of ionic compounds in the same way as those of the covalent ones, with a line between symbols of atoms implying the existence of mutual electron pairs. Many teachers apparently accept this scheme and make calotte models for every entity of every substance, including the ionic ones (Aleksovska, 2011). In this way, they create a distorted picture in the minds of their students and such a picture lasts for a very long time (in many cases, we dare say, it is a lifelong miscon- ception). There are some definitions of crystalline hydrates (Alek- sovska & Antonovska, 2010: p. 130) that use both macro and micro viewpoints. The above textbook defines crystalline hy- drates as: “A large number of salts obtained from aqueous solu- tions, in their structure, contain one or more molecules of wa- ter. These salts are called crystalline hydrates.” Obviously, if such definitions are encountered in the very early stages of the chemistry (or science) education, they might have harmful consequences and make difficult the discrimination between substances and their building blocks (particles). M4: In a process of ice melting, the volume of the system (mixture of ice and liquid water) increases and M5: Parti- cles change from solid to liquid ones. The most common misconceptions were those that reflected ideas about the dimensions and shape of molecules when the substance undergoes a phase change or any other change pro- voked by the changes in temperature or pressure. We tried to gauge the opinions that students have concerning very familiar phenomena: ice melting and freezing of liquid water. The total percentage of the incorrect answer (M4) decreases in the post-test (cf. Table 4), as one should expect. However, the scores of the eighth-grade students are not in agreement with the overall outcome (the reasons for this finding are not quite clear, but one could speculate that, being the youngest group, their overall chemical knowledge is restricted). To make items 3 and 4 more understandable, the researcher and students discussed (through interviews and instruction) about many everyday examples, such as the lakes freezing on the surface, the density of ice cubes, the bursting of plastic water-filled bottles when put in deep-freeze, the rock breaking due to freezing of water inside the gaps etc. It was found (Demircioğlu et al., 2005) that when chemistry concepts were related to everyday life during teaching, their retention in the learner’s mind was greater. On the other hand, Oloruntegbe et al. (2010) reported that students could not relate science con- cept learnt in school to home activities. During focus group discussions, most students, explaining the ice melting, recalled their everyday experiences but the offered explanations were not always the scientifically accepted ones: “My answer is that particles change from solid to liquid ones. For example, if we put ice cubes in a drink, after same time they will melt and turn to liquid state. According to this, I thought that this answer is the most appropriate.” (SS- VIII-high achievers) The responses of students about changing the “state” of the particles are reported in the literature (Griffiths & Preston, 1992). In his study, Taber (2001) claims that it is common for learners to make statements such as: “The substance melt be- cause its molecules melt.” As indicated before, 33.3% of secondary (eighth-grade) school students thought that particles receive heat and change their size. This view was clearly shown in the following ex- cerpts from one of the secondary school groups’ discussion: “An ice cube ··· when exposed to sunlight and heat, starts to melt and its particles broaden.” (SS-VIII-low achievers) or “I think that particles in ice are smaller than those in water ··· and when ice begins to melt, the volume decreases.” (SS-VIII-low achievers) M6: When ethanol is ignited, carbon, hydrogen and oxy- gen atoms, of which ethanol is composed, will ignite too and will burn just like the ethanol. The fifth item on the test deals with the nature of atoms in a chemical reaction. As an example of chemical reaction we con- sidered the combustion of ethanol. The concept of burning or combustion of certain substances can be found in the eighth-grade curriculum, and in the subsequent education it is studied in more details. Explanations about reactions such as burning of magnesium, ethyne or ethanol as well as the chemi- cal equations for the corresponding reactions can be found in many textbooks. One should, therefore, expect that students will be able to write down a chemical equation for this reaction and give a proper explanation. It was found, however, that stu- dents hold both tested misconceptions: (a) 26.2% of 187 stu- dents thought that atoms may be ignited and burned (M6) and (b) 14.4% thought that atoms disappear during chemical reac- tions. The latter was found to be the most prevalent option in the second-year high-school sub-sample. However, during interviewing we could notice some failures in knowledge and vagueness about the students’ perceptions about atoms. These are illustrated bellow. R: “Why do you think that atoms will burn?” S15: “Well, because ethanol has oxygen in its structure and it is able to burn.” (SS-VIII-high achievers) Some other type of misconceptions regarding the phase changes and the size of the particles were also documented. Some students believed that the particle size must be changed, while others failed in distinguishing between physical and chemical change. Here are comments of the students regarding these issues. R: “What will happen with the particles when a substance is ignited?” S21: “They change their phase.” S22: “I stick to my opinion that they will either increase their size or decrease it, because when they undergo a phase transi- tion their shape will be changed. They must be changed; they Copyright © 2012 SciRes. 625
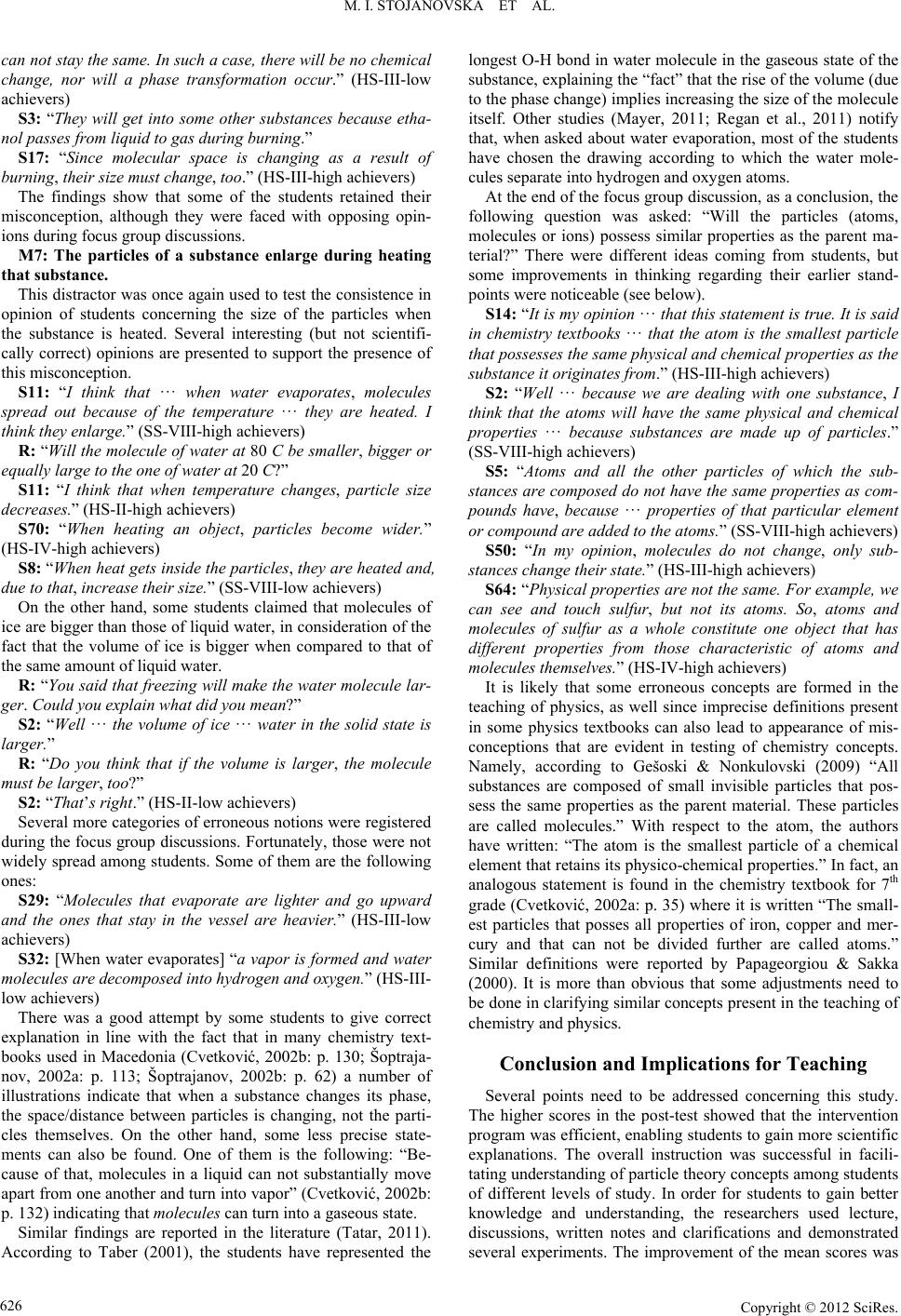 M. I. STOJANOVSKA ET AL. can not stay the same. In such a case, there will be no chemical change, nor will a phase transformation occur.” (HS-III-low achievers) S3: “They will get into some other substances because etha- nol passes from liquid to gas during burning.” S17: “Since molecular space is changing as a result of burning, their size must change, too.” (HS-III-high achievers) The findings show that some of the students retained their misconception, although they were faced with opposing opin- ions during focus group discussions. M7: The particles of a substance enlarge during heating that substance. This distractor was once again used to test the consistence in opinion of students concerning the size of the particles when the substance is heated. Several interesting (but not scientifi- cally correct) opinions are presented to support the presence of this misconception. S11: “I think that ··· when water evaporates, molecules spread out because of the temperature ··· they are heated. I think they enlarge.” (SS-VIII-high achievers) R: “Will the molecule of water at 80 C be smaller, bigger or equally large to the one of water at 20 C?” S11: “I think that when temperature changes, particle size decreases.” (HS-II-high achievers) S70: “When heating an object, particles become wider.” (HS-IV-high achievers) S8: “When heat gets inside the particles, they are heated and, due to that, increase their size.” (SS-VIII-low achievers) On the other hand, some students claimed that molecules of ice are bigger than those of liquid water, in consideration of the fact that the volume of ice is bigger when compared to that of the same amount of liquid water. R: “Y ou said that freezing will make the water molecule lar- ger. Could you explain what did you mean?” S2: “Well ··· the volume of ice ··· water in the solid state is larger.” R: “Do you think that if the volume is larger, the molecule must be larger, too?” S2: “That’s right.” (HS-II-low achievers) Several more categories of erroneous notions were registered during the focus group discussions. Fortunately, those were not widely spread among students. Some of them are the following ones: S29: “Molecules that evaporate are lighter and go upward and the ones that stay in the vessel are heavier.” (HS-III-low achievers) S32: [When water evaporates] “a vapor is formed and water molecules are decomposed into hydrogen and oxygen.” (HS-III- low achievers) There was a good attempt by some students to give correct explanation in line with the fact that in many chemistry text- books used in Macedonia (Cvetković, 2002b: p. 130; Šoptraja- nov, 2002a: p. 113; Šoptrajanov, 2002b: p. 62) a number of illustrations indicate that when a substance changes its phase, the space/distance between particles is changing, not the parti- cles themselves. On the other hand, some less precise state- ments can also be found. One of them is the following: “Be- cause of that, molecules in a liquid can not substantially move apart from one another and turn into vapor” (Cvetković, 2002b: p. 132) indicating that molecules can turn into a gaseous state. Similar findings are reported in the literature (Tatar, 2011). According to Taber (2001), the students have represented the longest O-H bond in water molecule in the gaseous state of the substance, explaining the “fact” that the rise of the volume (due to the phase change) implies increasing the size of the molecule itself. Other studies (Mayer, 2011; Regan et al., 2011) notify that, when asked about water evaporation, most of the students have chosen the drawing according to which the water mole- cules separate into hydrogen and oxygen atoms. At the end of the focus group discussion, as a conclusion, the following question was asked: “Will the particles (atoms, molecules or ions) possess similar properties as the parent ma- terial?” There were different ideas coming from students, but some improvements in thinking regarding their earlier stand- points were noticeable (see below). S14: “It is my opinion ··· that this statement is true. It is said in chemistry textbooks ··· that the atom is the smallest particle that possesses the same physical and chemical properties as the substance it originates from.” (HS-III-high achievers) S2: “Well ··· because we are dealing with one substance, I think that the atoms will have the same physical and chemical properties ··· because substances are made up of particles.” (SS-VIII-high achievers) S5: “Atoms and all the other particles of which the sub- stances are composed do not have the same properties as com- pounds have, because ··· properties of that particular element or compound are added to the atoms.” (SS-VIII-high achievers) S50: “In my opinion, molecules do not change, only sub- stances change their state.” (HS-III-high achievers) S64: “Physical properties are not the same. For example, we can see and touch sulfur, but not its atoms. So, atoms and molecules of sulfur as a whole constitute one object that has different properties from those characteristic of atoms and molecules themselves.” (HS-IV-high achievers) It is likely that some erroneous concepts are formed in the teaching of physics, as well since imprecise definitions present in some physics textbooks can also lead to appearance of mis- conceptions that are evident in testing of chemistry concepts. Namely, according to Gešoski & Nonkulovski (2009) “All substances are composed of small invisible particles that pos- sess the same properties as the parent material. These particles are called molecules.” With respect to the atom, the authors have written: “The atom is the smallest particle of a chemical element that retains its physico-chemical properties.” In fact, an analogous statement is found in the chemistry textbook for 7th grade (Cvetković, 2002a: p. 35) where it is written “The small- est particles that posses all properties of iron, copper and mer- cury and that can not be divided further are called atoms.” Similar definitions were reported by Papageorgiou & Sakka (2000). It is more than obvious that some adjustments need to be done in clarifying similar concepts present in the teaching of chemistry and physics. Conclusion and Implications for Teaching Several points need to be addressed concerning this study. The higher scores in the post-test showed that the intervention program was efficient, enabling students to gain more scientific explanations. The overall instruction was successful in facili- tating understanding of particle theory concepts among students of different levels of study. In order for students to gain better knowledge and understanding, the researchers used lecture, discussions, written notes and clarifications and demonstrated several experiments. The improvement of the mean scores was Copyright © 2012 SciRes. 626
 M. I. STOJANOVSKA ET AL. shown to be statistically significant for all sub-samples. Fur- thermore, the better results in the post-tests (for all samples) are in favor of the conclusion that indeed there is a noticeable im- provement in the achievements of students for all test items (except for one in the sample of eighth-grade students). With respect to the trends in understanding the particle the- ory concepts by students across the various levels of study, an increase of the t-values was noticed, although the second value in Table 2 is opposite to this reasoning. Nevertheless, it was practically impossible to detect a strict regularity about the learning progress and the achievement of students since there was not a big difference in understanding of students of differ- ent age. Similar results are reported in the literature (Özmen, 2011). One of the main points of this research was to detect misun- derstandings and difficulties regarding the particle theory con- cepts, especially those related to the three levels of thinking (macroscopic, sub-microscopic and symbolic). Seven miscon- ceptions represented by more than 20% were registered among students of all levels of study and three additional misconcep- tions were found in certain sub-samples. Many students claimed that the particles either decrease or increase their size when a substance is heated, their notions originating (errone- ously) from the observations of the macroscopic properties of substances. Approximately 35% of the students fail to recog- nize the ionic nature of copper(II) sulfate and even 88% of them believe that the substance (butadiene) reacts with one or more entities (atoms or molecules of bromine). At this point, it must be emphatically stated that neither the substances are molecules nor molecules are substances and such statements should be explicitly given in the textbooks and repeated, over and over again, by the teachers in the classrooms. In this re- spect, it is of little comfort that even the Nobel Foundation reported that the Nobel Prize in Physiology or Medicine 1998 was awarded jointly to Robert F. Furchgott, Louis J. Ignarro and Ferid Murad “for their discoveries concerning nitric oxide as a signalling molecule in the cardiovascular system” (The Nobel Prize in Physiology or Medicine 1998), thus showing how widespread is the imprudent mixing of macroscopic (nitric oxide, a substance) and sub-microscopic (molecule, a particle) points of view. We tried to get insight into ideas of the students about the particles and their connection to substances using a concept test and group discussions. The test items (which were developed by the authors) are provided in Appendix 1 and can be used to repeat the research to a different sample and to compare the findings. Although generalization beyond the data of this sam- ple can not be guaranteed, some considerations could be valu- able. In this study, we found out that a large portion of students held the belief that the molecules have the properties of the bulk matter. The reasons for emergence of these misconcep- tions are mainly due to the failure to distinguish between mac- roscopic and microscopic properties (Ben-Zvi & Gai, 1994; Bucat, 2004; Chandrasegaran et al., 2007; Treagust et al., 2011). Furthermore, the data analysis from the tests and focus group interviews clearly showed that students had certain difficulties about: 1) recognizing symbolic representations (e.g. confusing symbols for atoms and formulae for molecules); 2) making distinction between ionic and covalent substances and their particles and 3) the ability to distinguish substances from molecules (i.e. differentiate concepts at macroscopic and mi- croscopic level). The low understanding about the particle theory concepts may originate from the unstable previous knowledge of stu- dents and emphasising the rote learning (Dhindsa & Treagust, 2009; Salame et al., 2011) without making connections between tangible macroscopic phenomena and changes at the molecular level. The abstract nature of many chemical terms (such as atoms, molecules, ions, electrons etc.) may also be a source of misconceptions. The fact that we are not able to see particles and their interconnection and interaction, compels us to apply a model which would help in acquiring understanding. As Coll & Treagust (2003) noted “To understand and explain the macro- scopic, we need to have a picture or image-a model-of what is going on at the microscopic level”. Therefore, the necessity of using visualization techniques (models, animations or computer software) is obvious and it was mentioned as a required tool in teaching abstract concepts (José & Williamson, 2005; Milne, 1999). However, introducing models or images to students must be done with caution; otherwise it could be unproductive or even counterproductive. Eilks et al. (2012), with reference to their earlier studies, state that curriculum developers and text- book authors not always carefully incorporate important re- search evidence when preparing learning materials, as a result of which potentially misleading visualisations can be found in some German textbooks. Particle theory concepts are an integral part of the eighth- grade secondary-school curriculum in Republic of Macedonia. At the very beginning of learning chemistry as a subject, stu- dents encounter the bulk properties (physical and chemical) of substances, their changes, classifications etc. Next, they learn about the structure of substances. Chemical symbols, formulae and equations come later. Nonetheless, the results showed that the sample of students have not developed an accurate under- standing of these concepts. Furthermore, a remarkable consis- tency in reasoning was observed among students of different levels of study, thus confirming the fact that many students retain their misconceptions over the years. Therefore, it is of utmost importance to make a clear differ- entiation between sub-microscopic entities and macroscopic phenomena in chemistry (Silberberg, 2006) and, consequently, their appropriate implementation in chemistry teaching. By all means, a special caution should be taken when teaching the youngest students in order to enable them to create proper mental images concerning the basic chemical concepts. There are, at least, two main starting points useful in teaching chemistry: a) the “cognitive conflict” strategy that includes the use of diagnostic questions whose purpose is to stimulate stu- dents to talk about their ideas and utilizing the answers of the participating students to establish and organize teaching and b) carefully introducing the new material (ideas or concepts) visu- ally, using pictures, animations and models of atoms and molecules and presenting new ideas consistently in other topics, thus forming an all-inclusive, integrated whole, instead of teaching different topics isolated. Unfortunately, within the educational system in Macedonia many obstacles exist (including financial, experimental, per- sonal prerequisites and, especially, the time allowed to the teachers) which would hamper the implementation of our deep conviction outlined above. Our future studies will be aimed at other aspects of this (or a similar) research. An important issue will be testing the influ- ence of gender on achievements of students. Preparing teaching materials to reduce erroneous notions (and help teacher to di- Copyright © 2012 SciRes. 627
 M. I. STOJANOVSKA ET AL. agnose and address misconceptions before the instruction proc- ess) is deemed equally important. Acknowledgements The authors wish to express their sincere thanks to Prof. Bo- rislav Toshev, D.Sc. (University of Sofia, Bulgaria) for critical reading of the manuscript and the valuable suggestions he made. REFERENCES Aleksovska, S., & Antonovska, L. (2010). Chemistry for the 7th grade of eighth-year secondary education. Skopje: Ministry of Education and Science of Republic of Macedonia (in Macedonian). Aleksovska, S., & Stojanovski, K. (2003). Chemistry for the 3rd year of reformed gymnasium education. Skopje: Prosvetno Delo (in Mace- donian). Aleksovska, S., & Stojanovski, K. (2005). Chemistry for the 4th year of reformed gymnasium education. Skopje: Prosvetno Delo (in Mace- donian). Aleksovska, S. (2011). Personal communication. Anonimous (2003). Common misconceptions about matter. URL (last checked 2 July 2012). http://www.arps.org/users/ms/pricen/one%20pagers/common_misco nceptions%20chemistry.pdf Badrian, A., Abdinejad, T., & Naseriazar, A. (2011). A cross-age study of Iranian students’ various conceptions about the particulate nature of matter. Journal of T u rkish Science Education, 8 , 49-63. Banda, A., Mumba, F., Chabalengula, V. M., & Mbewe, S. (2011). Teachers’ understanding of the particulate nature of matter: The case of Zambian pre-service science teachers. Asia-Pacific Forum on Science Learning and Teaching, 1 2 , Article ID: 4. Barke, H. D., Hazari, A., & Yitbarek, S. (2009). Chapter 2: Students’ misconceptions and how to overcome them. In: Misconceptions in chemistry addressing perceptions in chemical education (pp. 21-36). Berlin: Springer-Verlag. BBC (2012). KS3 Bitesize: Science—Behaviour of matter. URL (last checked 2 July 2012). http://www.bbc.co.uk/schools/ks3bitesize/science/chemical_material_b ehaviour/behaviour_of_matter/activity.shtml Ben-Zvi, N., & Gai, R. (1994). Macro- and micro-chemical compre- hension of real-world phenomena. Journal of Chemical Education, 71, 730-732. doi:10.1021/ed071p730 Ben-Zvi, R., Eylon, B., & Silberstein, J. (1986). Is an atom of copper malleable? Journal of Chemical Education, 63, 64-66. doi:10.1021/ed063p64 Bindernagel, J. A., & Eilks, I. (2009). Evaluating roadmaps to portray and develop chemistry teachers’ PCK about curricular structures concerning sub-microscopic models. Chemistry Education Research and Practice, 10, 77-85. doi:10.1039/b908245j Bridle, C. A., & Yezierski, E. J. (2011). Evidence for the effectiveness of inquiry-based, particulate-level instruction on conceptions of the particulate nature of matter. Journal of Chemical Education, 89, 192- 198. doi:10.1021/ed100735u Bucat, B. (2004). Pedagogical content knowledge as a way forward: Applied research in chemistry education. Chemistry Education Re- search and Practice, 5, 215-228. doi:10.1039/b4rp90025a Çalýk, M., Ayas, A., & Ebenezer, J. V. (2005). A review of solution chemistry studies: Insights into students’ conceptions. Journal of Science Education and Techno l ogy, 14, 29-50. Canpolat, N. (2006). Turkish undergraduates’ misconceptions of eva- poration, evaporation rate, and vapour pressure. International Jour- nal of Science Education, 28 , 1757-1770. doi:10.1080/09500690600779957 Chandrasegaran, A. L., Treagust, D. F., & Mocerino, M. (2007). The development of a two-tier multiple-choice diagnostic instrument for evaluating secondary school students’ ability to describe and explain chemical reactions using multiple levels of representation. Chemistry Education Research and Practice, 8, 293-307. doi:10.1039/b7rp90006f Chiu, M.-H. (2005). A national survey of students’ conceptions in chemistry in Taiwan. Chemical Education Internationa l, 6, 8 p. Cliff, W. H. (2009). Chemistry misconceptions associated with under- standing calcium and phosphate homeostasis. Advances in Physiol- ogy Education, 33, 323-328. doi:10.1152/advan.00073.2009 Coll, R. K., & Treagust, D. F. (2003). Investigation of secondary school, undergraduate, and graduate learners’ mental models of ionic bond- ing. Journal of Research in Science Teaching, 40, 464-486. doi:10.1002/tea.10085 Cvetković, S. (2002a). Chemistry for the 7th grade. Skopje: Prosvetno Delo (in Macedonian). Cvetković, S. (2002b). Chemistry for the 1st year of reformed gymna- sium education. Skopje: Prosvetno Delo (in Macedonian). De Vos, W., & Verdonk, A. H. (1996). The particulate nature of matter in science education and in science. Journal of Research in Science Teaching, 33, 657-664. doi:10.1002/(SICI)1098-2736(199608)33:6<657::AID-TEA4>3.0.C O;2-N Demircioğlu, G. (2009). Comparison of the effect of conceptual change texts implemented after and before instruction on secondary school students’ understanding of acid-base concepts. Asia-Pacific Forum on Science Learning and Teaching, 10, Article ID: 5. Demircioğlu, G., Ayas, A., & Demircioğlu, H. (2005). Conceptual change achieved through a new teaching program on acids and bases. Chemistry Education Research and Practi ce, 6, 36-51. doi:10.1039/b4rp90003k Dhindsa, H. S., & Treagust, D. F. (2009). Conceptual understanding of Bruneian tertiary students: Chemical bonding and structure. Brunei International Journal of Science & Mathematics Education, 1, 33-51. Eilks, I., Witteck, T., & Pietzner, V. (2012). The role and potential dangers of visualisation when learning about sub-microscopic ex- planations in chemistry education. Centre for Educational Policy Studies Journal, 2, 125-145. Eybe, H., & Schmidt, H. (2004). Group discussions as a tool for inves- tigating students’ concepts, Chemistry Education Research and Practice, 5, 265-280. doi:10.1039/b4rp90028f Gabel, D. L., & Samuel, K. V. (1987). Understanding the particulate nature of matter. Journal of Chemical Educatio n, 64, 695-697. doi:10.1021/ed064p695 Gešoski, S., & Nonkulovski, F. (2009). Physics for the 7th grade of eighth-year-secondary education. Skopje: Ministry of Education and Science of Republic of Macedonia (in Macedonian). Gregorius, R. M. (n.d.). An eBook in flash to support inductive learning. URL (last checked 2 July 2012). http://www3.canisius.edu/~gregorir/ic2home/eBookPaper.pdf Griffiths, A. K., & Preston, A. K. (1992). Grade-12 students’ miscon- ceptions relating to fundamental characteristics of atoms and mole- cules. Journal of Research in Science Teaching, 29, 611-628. doi:10.1002/tea.3660290609 Herrmann Abell, C. F., & DeBoer, G. E. (2011). Using distractor-given standard-based multiple-choice assessment and Rasch modelling to investigate hierarchies of chemistry misconceptions and detect structural problems with individual items. Chemistry Education Re- search and Practise, 12, 184-192. doi:10.1039/c1rp90023d Hoque, K. E., Alam, G. M., Ariff, M. R. B. M., Mishra, P. K., & Rabby, T. G. (2011). Site-based management: Impact of leader’s roles on in- stitutional improvement. African Journal of Business Management, 5, 3623-3629. Horton, C. (With Other Members of the Modeling Instruction in High School Chemistry Action Research Teams at Arizona State Univer- sity) (2007). Student Alternative Conceptions in Chemistry. URL (last checked 2 July 2012). http://www.scribd.com/doc/52664732/student-alternative-conception s-in-chemistry Hussein, A. (2009). The use of triangulation in social sciences research: Can qualitative and quantitative methods be combined? Journal of Comparative Soci al Work, 1, 1-12. Jick, T. D. (1979). Mixing qualitative and quantitative methods: Trian- gulation in action. Administrative Science Quaterly, 24, 602-611. Copyright © 2012 SciRes. 628
 M. I. STOJANOVSKA ET AL. doi:10.2307/2392366 Johnstone, A. H. (2000). Teaching of chemistry—Logical or psycho- logical? Chemistry Education Research and Practice in Europe, 1, 9-15. doi:10.1039/a9rp90001b José, T. J., & Williamson, V. M. (2005). Molecular visualization in science education: An evaluation of an NSF-sponsored workshop. Journal of Chemical Ed uc a t io n , 82, 937-943. doi:10.1021/ed082p937 Kabapınar, F. M. (2009). Multi-frame illustrations: A molecular visual strategy in learning and teaching chemistry concepts. Australian Journal of Education i n C h em i s t r y , 69, 11-16. Kariper, İ. A. (2011). An investigation into the misconceptions, erro- neous ideas and limited conception of the pH concept in pre-service science teacher education. The Chemical Education Journ a l, 14. Kind, V. (2004). Beyond appearances: Students’ misconceptions about basic chemical ideas (2nd ed.). Durham: Durham University. URL (last checked 2 July 2012). http://www.rsc.org/images/Misconceptions_update_tcm18-188603.p df Kvale, S. (1996). Chapter 7: The interview situation. In Interviews: An Introduction to Qualitative Research Interviewing (pp. 124-135). London: Sage. Levy Nahum, T., Hofstein, A., Mamlok-Naaman, R., & Bar-Dov, Z. (2004). Can final examinations amplify students’ misconceptions in chemistry? Chemistry Education Research and Pract ice, 5, 301-325. Margel, H., Eylon, B.-S., & Scherz, Z. (2004). “We actually saw atoms with our own eyes” conceptions and convictions in using the scan- ning tunneling microscope in junior high school. Journal of Chemi- cal Education, 81, 558-566. doi:10.1021/ed081p558 Mayer, K. (2011). Addressing students’ misconceptions about gases, mass, and composition. Journal of Chemical Ed u c a ti o n , 88, 111-115. doi:10.1021/ed1005085 Meijer, M. R. (2011). Macro-meso-micro thinking with structure- property relations for chemistry education. An explorative design- based study. Ph.D. Thesis, Utrecht: Utrecht University. Milne, R. W. (1999). Animating reactions. A low-cost activity for par- ticle conceptualization at the secondary level. Journal of Chemical Education, 76, 50-51. doi:10.1021/ed076p50 Monković, M., Ivanovski, V., & Petruševski V. M. (2007). Demonstra- tions as a tool for ironing-out misconceptions: 3. A note on the reac- tion Pb2+(aq) + 2I–(aq) = PbI2(s): Exceptions from a priori expecta- tions. The Australian Journal of Education in Chemistry, 68, 17-19. Morgіl, İ., & Yörük, N. (2006). Cross-age study of understanding of some concepts in chemistry subjects in science curriculum. Journal of Turkish Science Educat i o n, 3, 15-27. Mulford, D. R., & Robinson, W. R. (2002). An inventory for alternate conceptions among first-semester general chemistry students. Jour- nal of Chemical Education, 79, 739-744. doi:10.1021/ed079p739 Muzaffar, H., Castelli, D. M., Goss, D., Scherer, J. A., & Chapman- Novakofski, K. (2011). Middle school students want more than games for health education on the internet. Creative Education, 2, 393-397. doi:10.4236/ce.2011.24056 Nair, S., & Ngang, T. K. (2012). Exploring parent’s and teacher’s views of primary pupils’ thinking skills and problem solving skills. Creative Education, 3, 30-36. doi:10.4236/ce.2012.31005 Nelson, P. G. (2003). Basic chemical concepts. Chemistry Education Research and Practic e, 4, 19-24. doi:10.1039/b2rp90033e Nyachwaya, J. M., Mohamed, A., Roehrig, G. H., Wood, N. B., Kern, A. L., & Schneider, J. L. (2011). The development of an open-ended drawing tool: An alternative diagnostic tool for assessing students’ understanding of the particulate nature of matter. Chemistry Educa- tion Research and Practice, 12, 121-132. doi:10.1039/c1rp90017j Oloruntegbe, K. O., Ikpe, A., & Kukuru, J. D. (2010). Factors in stu- dents’ ability to connect school science with community and real- world life. Ed uc a t io n a l Research and Reviews, 5, 372-379. Onwu, G. O. M., & Randal, E. (2006). Some aspects of students’ un- derstanding of representational model of the particulate nature of matter in chemistry in three different countries. Chemistry Education Research and Practic e, 7, 226-239. doi:10.1039/b6rp90012g Osborne, J., & Collins, S. (2001). Pupils’ views of the role and value of the science curriculum: A focus group study. International Journal of Science Education, 23 , 441-467. doi:10.1080/09500690010006518 Özmen, H., & Kenan, O. (2007). Determination of the Turkish primary students’ views about the particulate nature of matter. Asia-Pacific Forum on Science Learning and Teaching, 8, Article ID: 1. Özmen, H. (2011). Turkish primary school students’ conceptions about the particulate nature of matter. Internationa Journal of Environ- mental & Science Education, 6, 99-121. Pabuçcu, A., & Geban, Ö. (2006). Remediating misconceptions con- cerning chemical bonding through conceptual change text. Hacettepe University Journal of Education, 30, 184-192. Papageorgiou, G., & Sakka, D. (2000). Primary school teschers’ views of fundamental chemical concepts. Chemistry Education Research and Practice, 1, 237-247. doi:10.1039/a9rp90025j Petruševski, V. M., Monković, M., & Šoptrajanov, B. (2007). Demon- strations as a tool for ironing-out preconceptions: 1. On the reactions of alkali metal sulfates with concentrated sulfuric acid. The Chemical Educator, 12, 1-4. Petruševski, V. M., Taseska, M., & Monković, M. (2006). Demonstra- tions as a tool for ironing-out misconceptions: 2. Can a strong acid be displaced by a weak one? The Chemical Education Journal, 9 . Regan, A., Childs, P., & Hayes, S. (2011). The use of an intervention programme to improve undergraduate students’ chemical knowledge and address their misconceptions, Chemistry Education Research and Practice, 12, 219-227. doi:10.1039/c1rp90027g Roschelle, J. (1995). Learning in interactive environments: Prior knowl- edge and new experience. In: J. H. Falk, & L. D. Dierking (Eds.), Public institutions for personal learning: Establishing a research agenda (pp. 37-51). Washington DC: American Association of Mu- seums. Salame, I. I., Sarowar, S., Begum, S., & Krauss, D. (2011). Students’ alternative conceptions about atomic properties and the periodic table. The Chemical Educator, 16, 190-194. Schmidt, H., Baumgärtner, T., & Eybe, H. (2003). Changing ideas about the periodic table of elements and students’ alternative concep- tions of isotopes and allotropes. Journal of Research in Science Teaching, 40, 257-277. doi:10.1002/tea.10076 Silberberg, M. S. (2006). Chemistry. The molecular nature of matter and change (4th ed.). Boston, MA: McGraw-Hill. Singh, P. (2008). The unexpected reward of qualitative research in assessment: A case example. The Qualitative Report, 13, 278-300. Sirhan, G. (2007). Learning difficulties in chemistry: An overview. Journal of Turkish Science Education, 4, 2-20. Skamp, K. (2009). Atoms and molecules in primary science: What are teachers to do? Australian Journal of Education in Chemistry, 69, 5- 10. Šoptrajanov, B. (2002a). Chemistry for the 1st year of reformed gym- nasium education. Skopje: Prosvetno Delo (in Macedonian). Šoptrajanov, B. (2002b). Chemistry for the 2nd year of reformed gym- nasium education. Skopje: Prosvetno Delo (in Macedonian). Sözbilir, M., Pınarbaşı, T., & Canpolat, N. (2010). Prospective chemis- try teachers’ conceptions of chemical thermodynamics and kinetics. Eurasia Journal of Mathematics, Science & Technology Education, 6, 111-121. Stojanovska, M. I., Petruševski, V. M., & Šoptrajanov, B. T. (2012a). On the existence of hydrogen salts of monoprotic acids. Journal of Chemical Education, 89, 1168-1170. doi:10.1021/ed2005483 Stojanovska, M., Petruševski, V. M., & Šoptrajanov, B. (2012b). The concept of sublimation—Iodine as an example. Educatión Quimica, 23, 171. Taber, K. (2001). Building the structural concepts of chemistry: Some considerations from educational research. Chemistry Education Re- search and Practice in Europe, 2, 123-158. doi:10.1039/b1rp90014e Taber, K. S. (2011). Models, molecules and misconceptions: A com- mentary on “Secondary school students’ misconceptions of covalent bonding”. Journ a l of Turkish Science E d u c a t i o n , 8, 3-18. Tasker, R. (1998). The VisChem project: Molecular level animations in chemistry—Potential and caution. UniServe Science News, 9, 12-16. Tatar, E. (2011). Prospective primary school teachers’ misconceptions about states of matter. Educational Research and Reviews, 6, 197- 200. The Nobel Prize in Physiology or Medicine (1998). URL (last checked Copyright © 2012 SciRes. 629
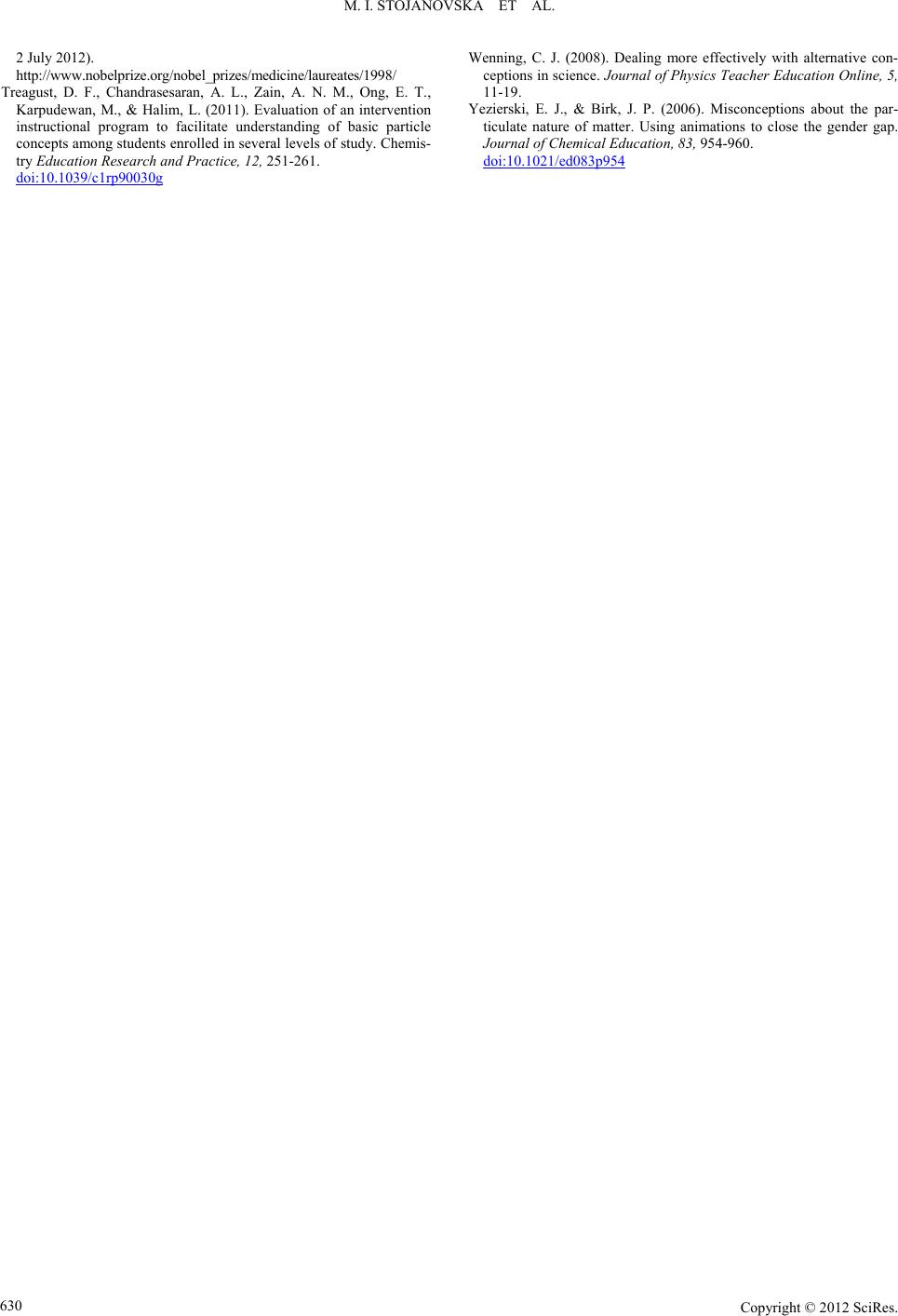 M. I. STOJANOVSKA ET AL. Copyright © 2012 SciRes. 630 2 July 2012). http://www.nobelprize.org/nobel_prizes/medicine/laureates/1998/ Treagust, D. F., Chandrasesaran, A. L., Zain, A. N. M., Ong, E. T., Karpudewan, M., & Halim, L. (2011). Evaluation of an intervention instructional program to facilitate understanding of basic particle concepts among students enrolled in several levels of study. Chemis- try Education Research and Practic e, 12, 251-261. doi:10.1039/c1rp90030g Wenning, C. J. (2008). Dealing more effectively with alternative con- ceptions in science. Journal of Physics Teacher Education Online, 5, 11-19. Yezierski, E. J., & Birk, J. P. (2006). Misconceptions about the par- ticulate nature of matter. Using animations to close the gender gap. Journal of Chemical Ed uc a t io n , 83, 954-960. doi:10.1021/ed083p954
 M. I. STOJANOVSKA ET AL. Appendix 1: The Multiple-Choice Instrument 1) Which of the following statements regarding the bromi- nation of butadiene represented by the equation CH2=CH-CH=CH2 + Br2 → CH2Br-CHBr-CH=CH2 correctly describes what happens? a) butadiene reacts with two bromines. b) butadiene reacts with one molecule of bromine. c) one mole butadiene reacts with one mole bromine. d) one gram butadiene reacts with one gram bromine. e) ______________________________________________ 2) Copper(II) sulfate from aqueous solution crystallizes: a) with five molecules of water. b) as a solid substance composed of copper(II) sulfate molecules. c) as а pentahydrate. d) as an anhydrous salt. e) ______________________________________________ 3) In the process of ice melting, the volume of the system (mixture of ice and liquid water): a) decreases. b) increases. c) remains the same. 4) The reason for your answer to the third question is: a) ice particles are smaller than the water particles. b) ice particles are bigger than the water particles. c) particles change from solid to liquid ones. d) the density of the system increases. e) the density of the system decreases. f) particles receive heat and enlarge. g) the change in temperature changes the size of the parti- cles. h) ______________________________________________ 5) What happens with the carbon, hydrogen and oxygen at- oms, of which ethanol is composed, when it is ignited? a) they will ignite too and will burn just like the ethanol. b) they will disappear. c) they will become part of some other substance(s), differ- ent from ethanol. d) ______________________________________________ 6) What happens with the particles of a substance during heating that substance? a) they enlarge. b) they shrink. c) their size does not change. d) ______________________________________________ Appendix 2: Interview Protocol Phase 1 (Briefing phase, not recorded) Information about the purpose of the research and explana- tions about the procedure of the interviewing process. Ethical considerations (permission for audio-tape record- ings, confidentiality of the obtained information etc.). Introductory questions, such as: “How do you picture atoms and molecules? What can you say about their appearance?”, “Name several substances and write down their symbolic representations”. “Are these substances composed of mole- cules?” Phase 2 (Main phase, recorded) Discussion related to M1 and M2: Write down one example of a chemical reaction. What do these symbolic representations stand for? What are the qualitative and quantitative information that can be drawn out? Discussion related to M3: Write down an example of a crystalline hydrate. What does this symbolic representation stand for? What are the quali- tative and quantitative information that can be drawn out? The discussion continues with students’ views of ionic and covalent substances, their distinction and their building par- ticles. Discussion related to M4 and M5: Have you ever put a plastic water-filled bottle in a deep- freeze? What happened? What happens if you put ice cubes in a glass of water? Why? Further discussions on the vol- ume and density of ice compared to those of liquid water (the lakes freezing on the surface, the rock breaking due to freezing of water inside the gaps etc.). Discussion related to M6: What happens with atoms of a certain substance upon burning? Is burning of alcohol different from the other types of burning? Discussion related to M5 and M7: [Making models of water molecules]. Water evaporates at any temperature, but this process is more significant upon heating and boiling. Could you describe what happens with the water molecules during evaporation? Water as a substance exists in three states: solid, liquid and gaseous. Explain what happens with the water molecules during a phase change. Do you think they are different in different phases? If you do, explain how. [An assignment was given to those students who claimed that molecules are different, to make new models of water molecules in dif- ferent phases]. Do you think that temperature, pressure or some other exter- nal factor have an influence on atoms or molecules? If you do, explain how. Do you have further examples of this? Gold has a characteristic golden color, copper is brick red and iodine is dark purple. Having this in mind, is it possible to draw a conclusion about the color of the atoms (or molecules) of which these substances consist? As a conclusion: Do you think that the properties of the building particles are the same as those of the parent material? Phase 3 (Debriefing phase, not recorded) Asking the participants whether they have anything else to add to the discussion or additional questions to pose. Copyright © 2012 SciRes. 631
|