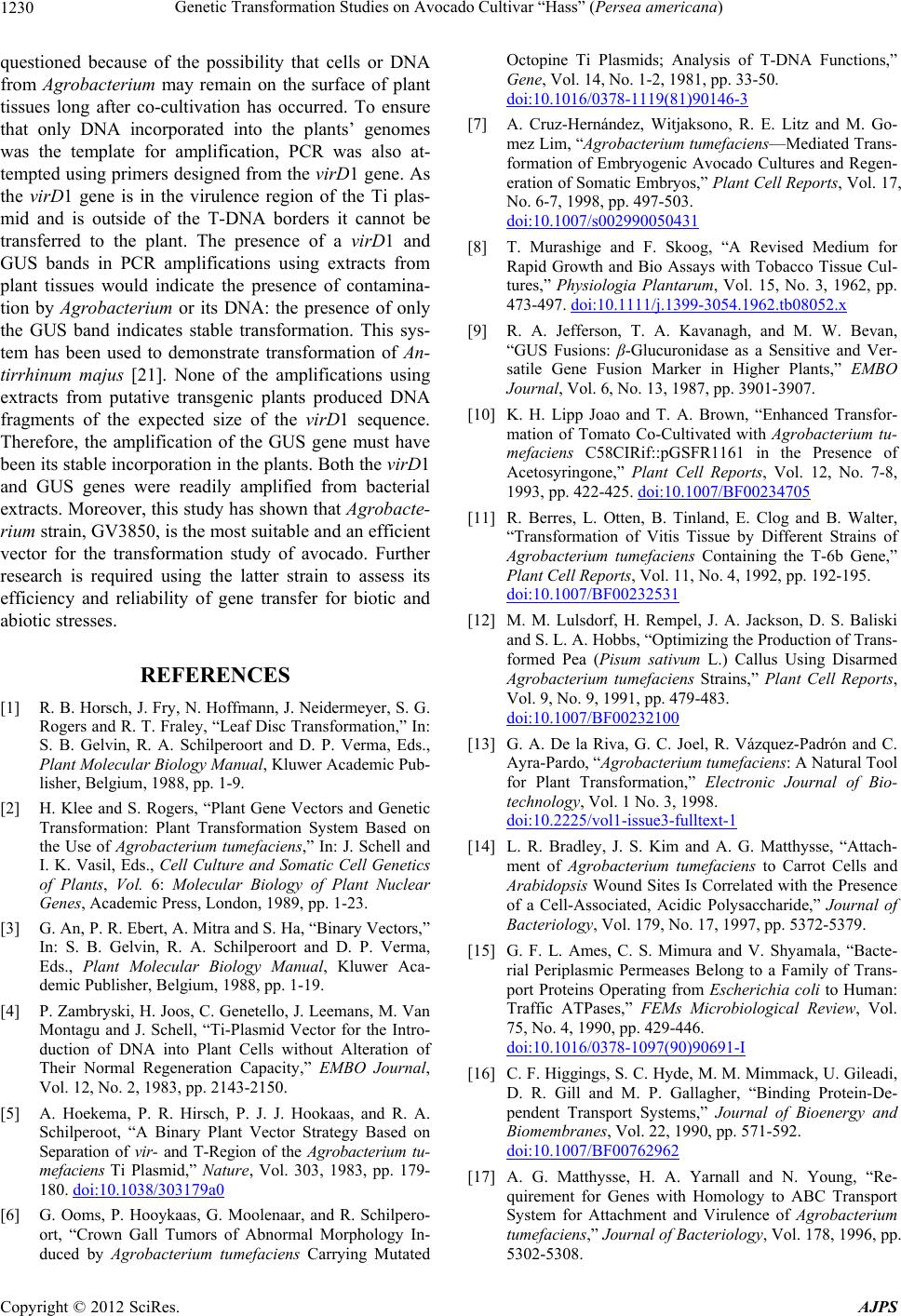
Genetic Transformation Studies on Avocado Cultivar “Hass” (Persea americana)
1230
questioned because of the possibility that cells or DNA
from Agrobacterium may remain on the surface of plant
tissues long after co-cultivation has occurred. To ensure
that only DNA incorporated into the plants’ genomes
was the template for amplification, PCR was also at-
tempted using primers designed from the virD1 gene. As
the virD1 gene is in the virulence region of the Ti plas-
mid and is outside of the T-DNA borders it cannot be
transferred to the plant. The presence of a virD1 and
GUS bands in PCR amplifications using extracts from
plant tissues would indicate the presence of contamina-
tion by Agrobacterium or its DNA: the presence of only
the GUS band indicates stable transformation. This sys-
tem has been used to demonstrate transformation of An-
tirrhinum majus [21]. None of the amplifications using
extracts from putative transgenic plants produced DNA
fragments of the expected size of the virD1 sequence.
Therefore, the amplification of the GUS gene must have
been its stable incorporation in the plan ts. Both the virD1
and GUS genes were readily amplified from bacterial
extracts. Moreover , this study has shown that Agrobacte-
rium strain, GV3850, is the most suitable and an efficient
vector for the transformation study of avocado. Further
research is required using the latter strain to assess its
efficiency and reliability of gene transfer for biotic and
abiotic stresses.
REFERENCES
[1] R. B. Horsch, J. Fry, N. Hoffmann, J. Neidermeyer, S. G.
Rogers and R. T. Fraley, “Leaf Disc Transformation,” In:
S. B. Gelvin, R. A. Schilperoort and D. P. Verma, Eds.,
Plant Molecular Biology Manual, Kluwer Academic Pub-
lisher, Belgium, 1988, pp. 1-9.
[2] H. Klee and S. Rogers, “Plant Gene Vectors and Genetic
Transformation: Plant Transformation System Based on
the Use of Agrobacterium tumefaciens,” In: J. Schell and
I. K. Vasil, Eds., Cell Culture and Somatic Cell Genetics
of Plants, Vol. 6: Molecular Biology of Plant Nuclear
Genes, Academic Press, London, 1989, pp. 1-23.
[3] G. An, P. R. Ebert, A. Mitra and S. Ha, “Binary Vectors,”
In: S. B. Gelvin, R. A. Schilperoort and D. P. Verma,
Eds., Plant Molecular Biology Manual, Kluwer Aca-
demic Publisher, Belgium, 1988, pp. 1-19.
[4] P. Zambry ski, H. Joos, C. Genetello, J. Leemans, M. Van
Montagu and J. Schell, “Ti-Plasmid Vector for the Intro-
duction of DNA into Plant Cells without Alteration of
Their Normal Regeneration Capacity,” EMBO Journal,
Vol. 12, No. 2, 1983, pp. 2143-2150.
[5] A. Hoekema, P. R. Hirsch, P. J. J. Hookaas, and R. A.
Schilperoot, “A Binary Plant Vector Strategy Based on
Separation of vir- and T-Region of the Agrobacterium tu-
mefaciens Ti Plasmid,” Nature, Vol. 303, 1983, pp. 179-
180. doi:10.1038/303179a0
[6] G. Ooms, P. Hooykaas, G. Moolenaar, and R. Schilpero-
ort, “Crown Gall Tumors of Abnormal Morphology In-
duced by Agrobacterium tumefaciens Carrying Mutated
Octopine Ti Plasmids; Analysis of T-DNA Functions,”
Gene, Vol. 14, No. 1-2, 1981, pp. 33-50.
doi:10.1016/0378-1119(81)90146-3
[7] A. Cruz-Hernández, Witjaksono, R. E. Litz and M. Go-
mez Lim, “Agrobacterium tumefaciens—Mediated Trans-
formation of Embryogenic Avocado Cultures and Regen-
eration of Somatic Embryos,” Plant Cell Reports, Vol. 17,
No. 6-7, 1998, pp. 497-503.
doi:10.1007/s002990050431
[8] T. Murashige and F. Skoog, “A Revised Medium for
Rapid Growth and Bio Assays with Tobacco Tissue Cul-
tures,” Physiologia Plantarum, Vol. 15, No. 3, 1962, pp.
473-497. doi:10.1111/j.1399-3054.1962.tb08052.x
[9] R. A. Jefferson, T. A. Kavanagh, and M. W. Bevan,
“GUS Fusions: β-Glucuronidase as a Sensitive and Ver-
satile Gene Fusion Marker in Higher Plants,” EMBO
Journal, Vol. 6, No. 13, 1987, pp. 3901-3907.
[10] K. H. Lipp Joao and T. A. Brown, “Enhanced Transfor-
mation of Tomato Co-Cultivated with Agrobacterium tu-
mefaciens C58CIRif::pGSFR1161 in the Presence of
Acetosyringone,” Plant Cell Reports, Vol. 12, No. 7-8,
1993, pp. 422-425. doi:10.1007/BF00234705
[11] R. Berres, L. Otten, B. Tinland, E. Clog and B. Walter,
“Transformation of Vitis Tissue by Different Strains of
Agrobacterium tumefaciens Containing the T-6b Gene,”
Plant Cell Reports, Vol. 11, No. 4, 1992, pp. 192-195.
doi:10.1007/BF00232531
[12] M. M. Lulsdorf, H. Rempel, J. A. Jackson, D. S. Baliski
and S. L. A. Hobbs, “Optimizing the Production of Trans-
formed Pea (Pisum sativum L.) Callus Using Disarmed
Agrobacterium tumefaciens Strains,” Plant Cell Reports,
Vol. 9, No. 9, 1991, pp. 479-483.
doi:10.1007/BF00232100
[13] G. A. De la Riva, G. C. Joel, R. Vázquez-Padrón and C.
Ayra-Pardo, “Agrobacterium tumefaciens: A Natural Tool
for Plant Transformation,” Electronic Journal of Bio-
technology, Vol. 1 No. 3, 1998.
doi:10.2225/vol1-issue3-fulltext-1
[14] L. R. Bradley, J. S. Kim and A. G. Matthysse, “Attach-
ment of Agrobacterium tumefaciens to Carrot Cells and
Arabidopsis Wound Sites Is Correlated with the Presence
of a Cell-Associated, Acidic Polysaccharide,” Journal of
Bacteriology, Vol. 179, No. 17, 1997, pp. 5372-5379.
[15] G. F. L. Ames, C. S. Mimura and V. Shyamala, “Bacte-
rial Periplasmic Permeases Belong to a Family of Trans-
port Proteins Operating from Escherichia coli to Human:
Traffic ATPases,” FEMs Microbiological Review, Vol.
75, No. 4, 1990, pp. 429-446.
doi:10.1016/0378-1097(90)90691-I
[16] C. F. Higgings, S. C. Hyde, M. M. Mimmack, U. Gileadi,
D. R. Gill and M. P. Gallagher, “Binding Protein-De-
pendent Transport Systems,” Journal of Bioenergy and
Biomembranes, Vol. 22, 1990, pp. 571-592.
doi:10.1007/BF00762962
[17] A. G. Matthysse, H. A. Yarnall and N. Young, “Re-
quirement for Genes with Homology to ABC Transport
System for Attachment and Virulence of Agrobacterium
tumefaciens,” Journal of Bacteriology, Vol. 178, 1996, pp.
5302-5308.
Copyright © 2012 SciRes. AJPS