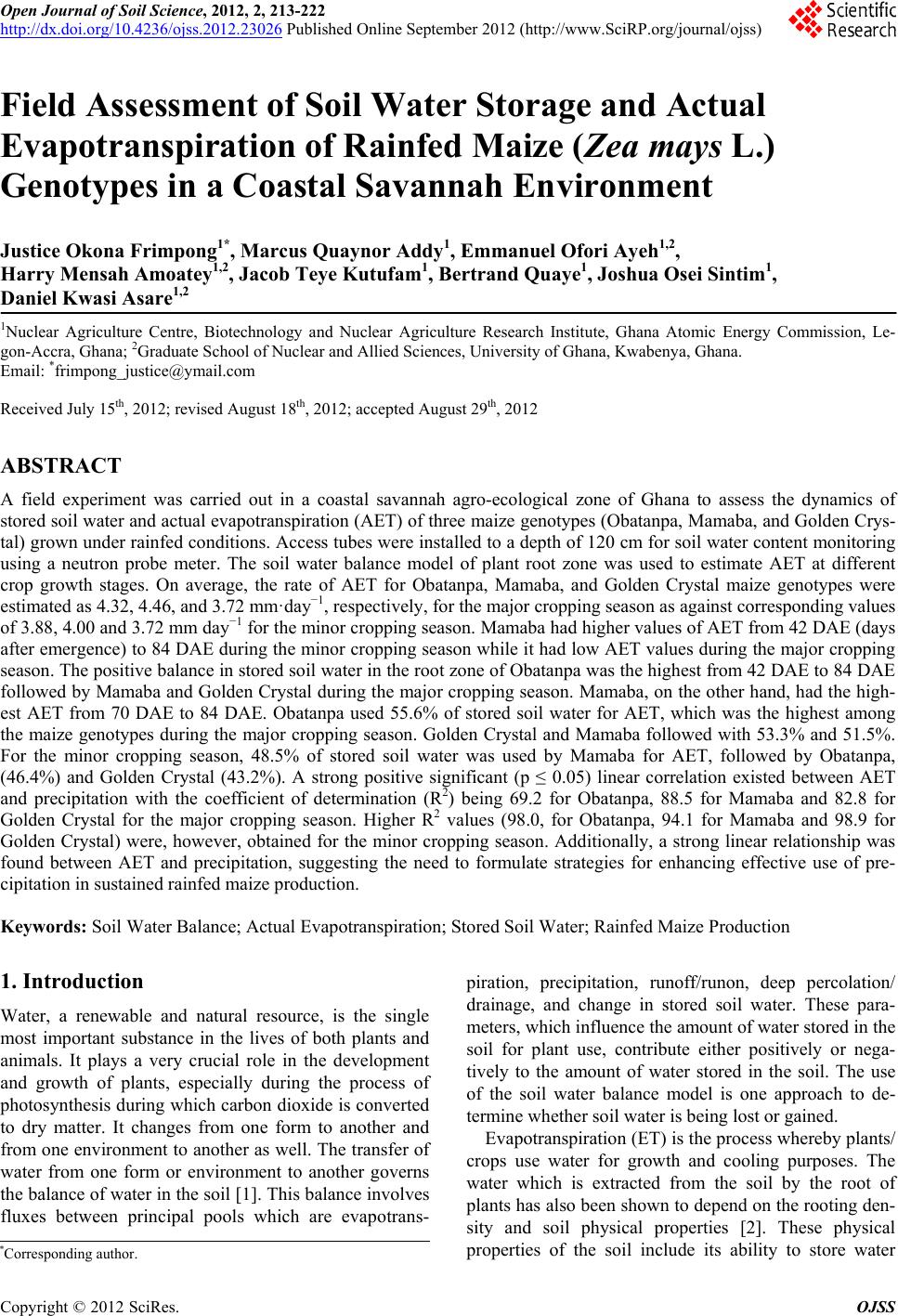 Open Journal of Soil Science, 2012, 2, 213-222 http://dx.doi.org/10.4236/ojss.2012.23026 Published Online September 2012 (http://www.SciRP.org/journal/ojss) 213 Field Assessment of Soil Water Storage and Actual Evapotranspiration of Rainfed Maize (Zea mays L.) Genotypes in a Coastal Savannah Environment Justice Okona Frimpong1*, Marcus Quaynor Addy1, Emmanuel Ofori Ayeh1,2, Harry Mensah Amoatey1,2, Jacob Teye Kutufam1, Bertrand Quaye1, Joshua Osei Sintim1, Daniel Kwasi Asare1,2 1Nuclear Agriculture Centre, Biotechnology and Nuclear Agriculture Research Institute, Ghana Atomic Energy Commission, Le- gon-Accra, Ghana; 2Graduate School of Nuclear and Allied Sciences, University of Ghana, Kwabenya, Ghana. Email: *frimpong_justice@ymail.com Received July 15th, 2012; revised August 18th, 2012; accepted August 29th, 2012 ABSTRACT A field experiment was carried out in a coastal savannah agro-ecological zone of Ghana to assess the dynamics of stored soil water and actual evapotranspiration (AET) of three maize genotypes (Obatanpa, Mamaba, and Golden Crys- tal) grown under rainfed conditions. Access tubes were installed to a depth of 120 cm for soil water content monitoring using a neutron probe meter. The soil water balance model of plant root zone was used to estimate AET at different crop growth stages. On average, the rate of AET for Obatanpa, Mamaba, and Golden Crystal maize genotypes were estimated as 4.32, 4.46, and 3.72 mm·day−1, respectively, for the major cropping season as against corresponding values of 3.88, 4.00 and 3.72 mm day−1 for the minor cropping season. Mamaba had higher values of AET from 42 DAE (days after emergence) to 84 DAE during the minor cropping season while it had low AET values during the major cropping season. The positive balance in stored soil water in the root zone of Obatanpa was the highest from 42 DAE to 84 DAE followed by Mamaba and Golden Crystal during the major cropping season. Mamaba, on the other hand, had the high- est AET from 70 DAE to 84 DAE. Obatanpa used 55.6% of stored soil water for AET, which was the highest among the maize genotypes during the major cropping season. Golden Crystal and Mamaba followed with 53.3% and 51.5%. For the minor cropping season, 48.5% of stored soil water was used by Mamaba for AET, followed by Obatanpa, (46.4%) and Golden Crystal (43.2%). A strong positive significant (p ≤ 0.05) linear correlation existed between AET and precipitation with the coefficient of determination (R2) being 69.2 for Obatanpa, 88.5 for Mamaba and 82.8 for Golden Crystal for the major cropping season. Higher R2 values (98.0, for Obatanpa, 94.1 for Mamaba and 98.9 for Golden Crystal) were, however, obtained for the minor cropping season. Additionally, a strong linear relationship was found between AET and precipitation, suggesting the need to formulate strategies for enhancing effective use of pre- cipitation in sustained rainfed maize production. Keywords: Soil Water Balance; Actual Evapotranspiration; Stored Soil Water; Rainfed Maize Production 1. Introduction Water, a renewable and natural resource, is the single most important substance in the lives of both plants and animals. It plays a very crucial role in the development and growth of plants, especially during the process of photosynthesis during which carbon dioxide is converted to dry matter. It changes from one form to another and from one environment to another as well. The transfer of water from one form or environment to another governs the balance of water in the soil [1]. This balance involves fluxes between principal pools which are evapotrans- piration, precipitation, runoff/runon, deep percolation/ drainage, and change in stored soil water. These para- meters, which influence the amount of water stored in the soil for plant use, contribute either positively or nega- tively to the amount of water stored in the soil. The use of the soil water balance model is one approach to de- termine whether soil water is being lost or gained. Evapotranspiration (ET) is the process whereby plants/ crops use water for growth and cooling purposes. The water which is extracted from the soil by the root of plants has also been shown to depend on the rooting den- sity and soil physical properties [2]. These physical properties of the soil include its ability to store water *Corresponding author. Copyright © 2012 SciRes. OJSS
 Field Assessment of Soil Water Storage and Actual Evapotranspiration of Rainfed Maize (Zea mays L.) Genotypes in a Coastal Savannah Environment 214 (soil water retention) for plant use and hydraulic con- ductivity i.e. the ease with which water moves within and between soil pores. Therefore, for plant growth and de- velopment to be normal, there must be a favourable bal- ance of water in the root zone of the plant. However, this balance has been under the influence of the prevailling weather conditions. Several factors have been known to limit rainfed maize production, the main factor being poor and erratic rainfall patterns [3,4], particularly in the savannah agro- ecological zone where maize production is extensively carried out. Frimpong et al. [5] reported rainfall amount of 502.4 mm and 290.7 mm during the major and minor maize cropping seasons, respectively, for the Kwabenya- Atomic area in the coastal savannah agro-ecological zone of Ghana. This poor amount of rainfall, which shares similar characteristics with rainfall conditions in some of the semi-arid regions of the world [6,7], affects the amount of stored soil water and the balance of soil water in the plant root zone as well as crop ET. Despite these limitations, several high yielding maize genotypes are being grown in this prevailing harsh environment [8]. As a result of the poor, erratic rainfall in this water stress prone environment, we assessed changes in stored soil water and balance of soil water in plant root zone as well as ET of three elite maize genotypes at different growth stages under rainfed conditions in a coastal sa- vannah agro-ecological zone. The information could be used to formulate strategies for ensuring effective and efficient use of stored soil water for sustainable rainfed maize production in the coastal savannah agro-ecological environment. 2. Materials and Methods The experimental site was the research farm of the Bio- technology and Nuclear Agriculture Research Institute (BNARI) of the Ghana Atomic Energy Commission (GAEC). The site lies on latitude 05˚40'N and longitude 0˚13'W and elevated at 76 m above the sea level. The study area, which is located in the coastal savannah agro- ecological zone of Ghana, receives less than 1000 mm of rainfall annually [9]. The mean monthly weather infor- mation for the study period is presented in Table 1. The soil at the site is the Haatso series, a well-drained sava- nnah ochrosol (Ferric Acrisol) [10] derived from quart- zite schist. Some of the chemical and physical chara- cteristics of the soil are presented in Table 2. The size of the experimental field was 27.0 m by 22.0 m, giving a total area of 594.0 m2. The randomised com- plete block design (RCBD) was used with four replicates. Three maize genotypes (Obatanpa, Mamaba and Golden Crystal) served as treatments. Each sub-plot measured 8.0 m by 4.0 m. Seeds of each maize genotype were sown on April 28, 2008 for the major season planting and September 01, 2008 for the minor season planting. Seed- lings were thinned to 2 plants per hill one week after germination to obtain a target plant density of 78,750 plants ha−1. The planting distance was 0.4 m within rows and 0.8 m between rows. Access tubes were installed in each of the sub plots to 120 cm soil depth before 50% seed ger- mination was observed. Access tubes were made of the polyvinyl chloride (PVC) pipe of 38.1 mm internal dia- meter with the bottom capped with same PVC material to Table 1. Monthly weather variables recorded at the experimental site during the study period. Months Tmax ˚C Tmin ˚C R. H. average % Sradiation M·J·m−2·day−1 Windspeed m·s−1 ETo mm·day−1 January 33.3 20.7 70.4 17.6 14.4 7.5 February 33.5 24.9 87.4 19.4 15.7 6.3 March 33.2 24.7 89.3 21.9 17.6 6.6 April 32.1 24.1 89.6 20.3 16.3 5.9 May 31.4 23.3 93.6 19.7 15.9 5.8 June 23.4 21.7 96.0 16.4 13.5 4.5 July 28.8 23.3 95.9 16.9 12.9 4.2 August 29.5 22.2 90.5 18.5 11.4 4.8 September 32.1 24.1 89.6 20.3 16.3 5.5 October 31.4 23.3 93.6 19.7 15.9 6.2 November 23.4 23.1 96 16.5 13.5 6.2 December 28.8 23.3 95.9 16.9 12.9 6.0 Average 30.1 23.2 90.7 18.7 14.7 5.8 Copyright © 2012 SciRes. OJSS
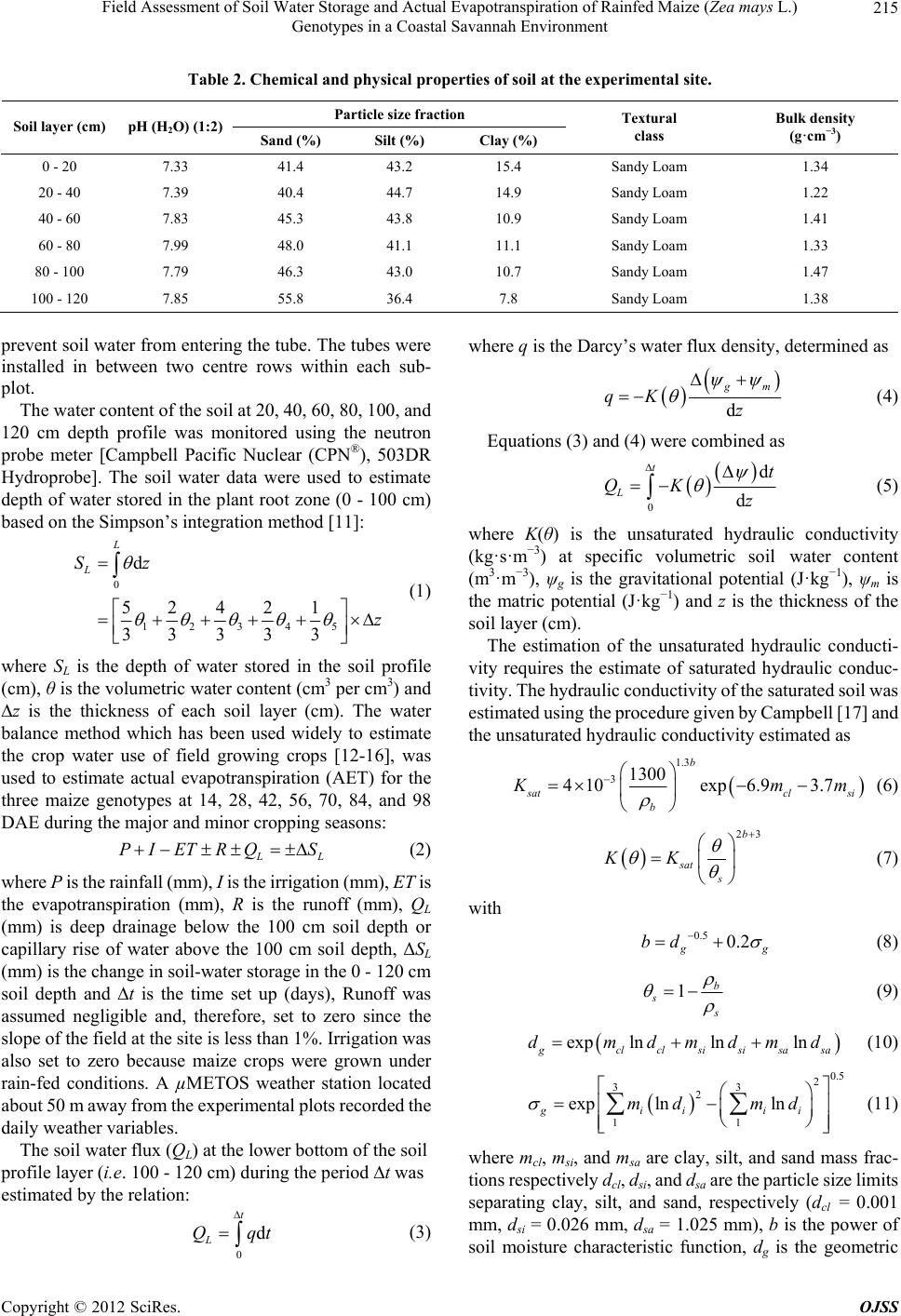 Field Assessment of Soil Water Storage and Actual Evapotranspiration of Rainfed Maize (Zea mays L.) Genotypes in a Coastal Savannah Environment 215 Table 2. Chemical and physical properties of soil at the experimental site. Particle size fraction Soil layer (cm) pH (H2O) (1:2) Sand (%) Silt (%) Clay (%) Textural class Bulk density (g·cm−3) 0 - 20 7.33 41.4 43.2 15.4 Sandy Loam 1.34 20 - 40 7.39 40.4 44.7 14.9 Sandy Loam 1.22 40 - 60 7.83 45.3 43.8 10.9 Sandy Loam 1.41 60 - 80 7.99 48.0 41.1 11.1 Sandy Loam 1.33 80 - 100 7.79 46.3 43.0 10.7 Sandy Loam 1.47 100 - 120 7.85 55.8 36.4 7.8 Sandy Loam 1.38 prevent soil water from entering the tube. The tubes were installed in between two centre rows within each sub- plot. The water content of the soil at 20, 40, 60, 80, 100, and 120 cm depth profile was monitored using the neutron probe meter [Campbell Pacific Nuclear (CPN®), 503DR Hydroprobe]. The soil water data were used to estimate depth of water stored in the plant root zone (0 - 100 cm) based on the Simpson’s integration method [11]: 0 12345 d 52 421 33 333 L L Sz z (1) where SL is the depth of water stored in the soil profile (cm), θ is the volumetric water content (cm3 per cm3) and ∆z is the thickness of each soil layer (cm). The water balance method which has been used widely to estimate the crop water use of field growing crops [12-16], was used to estimate actual evapotranspiration (AET) for the three maize genotypes at 14, 28, 42, 56, 70, 84, and 98 DAE during the major and minor cropping seasons: L PIETRQ S (2) where P is the rainfall (mm), I is the irrigation (mm), ET is the evapotranspiration (mm), R is the runoff (mm), QL (mm) is deep drainage below the 100 cm soil depth or capillary rise of water above the 100 cm soil depth, ΔSL (mm) is the change in soil-water storage in the 0 - 120 cm soil depth and ∆t is the time set up (days), Runoff was assumed negligible and, therefore, set to zero since the slope of the field at the site is less than 1%. Irrigation was also set to zero because maize crops were grown under rain-fed conditions. A µMETOS weather station located about 50 m away from the experimental plots recorded the daily weather variables. The soil water flux (QL) at the lower bottom of the soil profile layer (i.e. 100 - 120 cm) during the period ∆t was estimated by the relation: 0 d t L Qq where q is the Darcy’s water flux density, determined as d gm qK z (4) Equations (3) and (4) were combined as 0 d d t L t QK z (5) where K(θ) is the unsaturated hydraulic conductivity (kg·s·m−3) at specific volumetric soil water content (m3·m−3), ψg is the gravitational potential (J·kg−1), ψm is the matric potential (J·kg−1) and z is the thickness of the soil layer (cm). The estimation of the unsaturated hydraulic conducti- vity requires the estimate of saturated hydraulic conduc- tivity. The hydraulic conductivity of the saturated soil was estimated using the procedure given by Campbell [17] and the unsaturated hydraulic conductivity estimated as 1.3 31300 410exp 6.93.7 b atcl si b mm (6) 23b sat s KK (7) with 0.5 0.2 g bd (8) 1b s (9) exp lnlnln cl clsi si sa sa dmdmdmd (10) 0.5 2 33 2 11 exp lnln giiii md md (11) where mcl, msi, and msa are clay, silt, and sand mass frac- tions respectively dcl, dsi, and dsa are the particle size limits separating clay, silt, and sand, respectively (dcl = 0.001 mm, dsi = 0.026 mm, dsa = 1.025 mm), b is the power of soil moisture characteristic function, dg is the geometric t (3) Copyright © 2012 SciRes. OJSS
 Field Assessment of Soil Water Storage and Actual Evapotranspiration of Rainfed Maize (Zea mays L.) Genotypes in a Coastal Savannah Environment 216 mean particle diameter (mm), σg is the geometric standard deviation (mm), Ksat is the saturated hydraulic conducti- vity (kg·s·m−3), ρb is the soil bulk density (kg·m−3), K(θ) is the unsaturated hydraulic conductivity (kg·s·m−3), θ is the unsaturated moisture content (m3·m−3), θs is the saturated moisture content (m3·m−3). The matric potential (ψm) of soil at 80 - 100 cm and 100 - 120 cm soil layers was estimated based on the procedure given by Campbell [17]: b me s (12) 0.67 1300 b b ees (13) 0.5 0.5 es g d (14) where ψe is the air entry potential (J·kg−1) and ψes is the air entry potential (J·kg−1) at the soil bulk density of 1300 kg·m–3. Data on soil water in depth units, precipitation, and change in soil water storage were represented graphically with the aid of Microsoft excel in office 2007. Addi- tionally, the simple linear regression was used to deter- mine the correlation between AET and precipitation. 3. Results and Discussion 3.1. Climatic Characteristics at Experimental Site The highest maximum and minimum temperatures re- corded at experimental site were 33.5◦C and 24.9◦C for the month of February (Table 1). On the average, the maximum and minimum temperature throughout the year were 27.5◦C and 21.5◦C, respectively. The highest rela- tive humidity at the experimental site was 96 % which occurred in June and November. The mean relative humidity was 83%. Similarly, the highest solar radiation and wind speed at the site was 21.9 M·J·m−2·day−1 and 17.62 m·s−1, respectively, for the month of March. Ge- nerally, air temperatures, solar radiation, and wind speed were higher during the first half of the year as compare to the second half (Table 1). Generally, precipitation was higher during the major cropping season as compare to the minor cropping sea- son. Precipitations recorded for the major cropping sea- son from April-July were higher compare to values for the minor cropping season, September-December, (Fig- ure 1). The highest monthly total precipitation was re- corded in May (325.9 mm) during the major cropping season with the lowest occurring in June (89.0 mm). Similarly, during the minor cropping season, the monthly total precipitation was high in October (118.7 mm) with the lowest rainfall occurring in September (32.9 mm). 3.2. Soil Water Balance of Plant Root Zone The influence of the poor and erratic rainfall pattern on the soil water balance in the root zone of the three rainfed maize genotypes (Obatanpa, Mamaba, and Golden Crystal) is shown in Tables 3 an d 4. The observed trends in the balance of soil water in the root zone are similar to those reported by Baba-Kutigi et al. [18] for maize grown under rainfed conditions in the northern guinea savannah in Nigeria. Generally, the drainage component of the balance of soil water in the root zone for the three maize varieties was positive throughout the major and minor cropping seasons (Tables 3 and 4). This indicates that some amount of soil water was lost through deep drainage below the root zone which contributed negatively to the amount of water stored in the profile for plant use. This evidence con- tradicts the omission of the drainage component of the soil water balance model by some workers, based on the as- sumption that there was no drainage from the soil pro- file or better still the drainage component is insignificant under rainfed cropping systems [12-14,16,19]. 400 300 200 100 0 Precipitation (mm) Jan Feb Mar Apr May Jun Jul Aug Sep Oct Nov Dec Months Figure 1. Monthly precipitation distribution at Biotechnology and Nuclear Agriculture Research Institute research station in 2008 cropping season. Copyright © 2012 SciRes. OJSS
 Field Assessment of Soil Water Storage and Actual Evapotranspiration of Rainfed Maize (Zea mays L.) Genotypes in a Coastal Savannah Environment 217 Table 3. Soil water balance in the plant root zone of Obatanpa (O), Mamaba (M), and Golden Crystal (GC) during the major cropping season. Season DAE Maize Genotypes 1P (mm) 2I (mm) 3AET (mm)4D (mm) 5R (mm) 6ΔS (mm) O 21.1 - 22.0 1.8 - −0.8 Major 14 M 21.1 - 22.8 1.7 - 0.0 GC 21.1 - 20.6 1.5 - −1.9 O 42.1 - 18.2 1.9 - −25.9 Major 28 M 42.1 - 34.2 1.7 - −9.8 GC 42.1 - 27.5 1.4 - −16.1 O 63.2 - 89.1 1.6 - 24.3 Major 42 M 63.2 - 74.7 1.7 - 9.8 GC 63.2 - 76.9 1.5 - 12.1 O 54.2 - 63 1.6 - 7.3 Major 56 M 54.2 - 62.4 1.7 - 6.6 GC 54.2 - 61.9 1.6 - 6.2 O 46.1 - 55.5 1.4 - 7.9 Major 70 M 46.1 - 50.9 1.6 - 3.1 GC 46.1 - 60.1 1.8 - 12.2 O 88.8 - 102.7 1.5 - 12.3 Major 84 M 88.8 - 101.9 1.9 - 11.2 GC 88.8 - 104.4 2 - 13.6 O 103.7 - 82.9 1.5 - −22.3 Major 98 M 103.7 - 89.9 1.2 - −15.0 GC 103.7 - 90.5 2.4 - −15.5 1Precipitation; 2Irrigation; 3Runoff; 4Evapotranspiration; 5Drainage; 6Soil Water Storage. The balance of soil water in the root zone of the three maize genotypes was negative after monitoring on 14 DAE during the major cropping season under a uniform precipitation of 21.1 mm (Table 3). On the other hand, the balance of soil water was negative for Mamaba and Golden Crystal maize genotypes with the exception of Obatanpa for which the soil water balance was positive for the minor cropping season with an amount of precipitation of 14.2 mm (Table 4). A similar trend in the balance of soil water occurred at 28 DAE during both the major and minor cropping seasons. Similar results have been re- ported by Zhang et al. [20], for maize and winter wheat grown under different soil water treatments. For the major cropping season, the balance of water in the root zone was negative for the three maize genotypes with the root zone of Obatanpa losing the highest amount of water followed by those of Golden Crystal and Ma- maba. Soil water balance around the root of Golden Crystal was positive during the minor cropping season with Obatanpa and Mamaba recording a negative balance. The magnitude of water loss from the root zone was smaller for Obatanpa and Mamaba during minor cropping season as compared to that of Golden Crystal which gained water. The soil water balance in the root zone of the maize genotypes remained positive from 42 to 84 DAE during the major cropping season (Table 3). Obatanpa recorded the highest positive change in soil water storage at 42 DAE as against Mamaba which recorded the lowest at 70 DAE for the major cropping season. This gives an in- Copyright © 2012 SciRes. OJSS
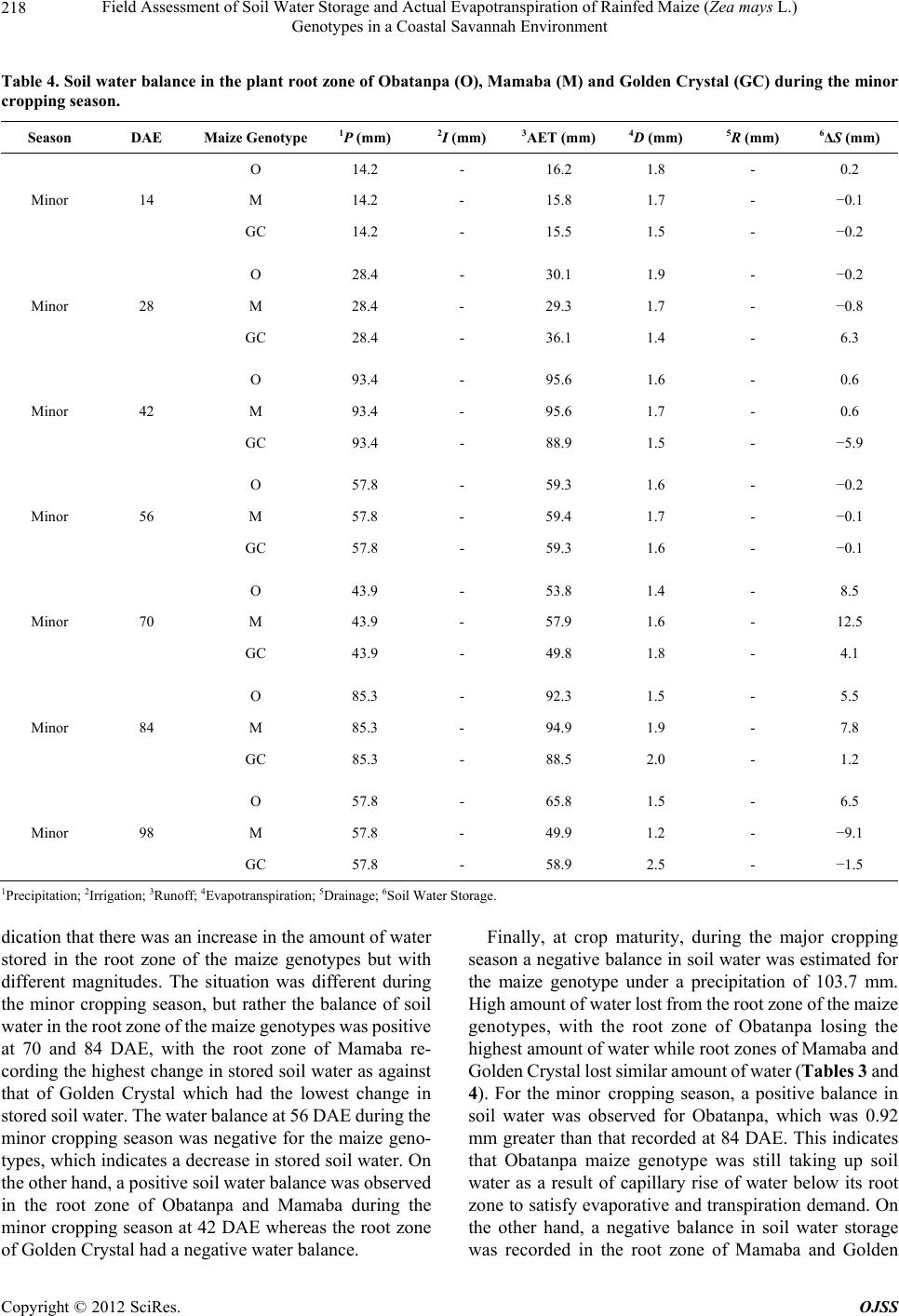 Field Assessment of Soil Water Storage and Actual Evapotranspiration of Rainfed Maize (Zea mays L.) Genotypes in a Coastal Savannah Environment 218 Table 4. Soil water balance in the plant root zone of Obatanpa (O), Mamaba (M) and Golden Crystal (GC) during the minor cropping season. Season DAE Maize Genotype 1P (mm) 2I (mm) 3AET (mm)4D (mm) 5R (mm) 6ΔS (mm) O 14.2 - 16.2 1.8 - 0.2 Minor 14 M 14.2 - 15.8 1.7 - −0.1 GC 14.2 - 15.5 1.5 - −0.2 O 28.4 - 30.1 1.9 - −0.2 Minor 28 M 28.4 - 29.3 1.7 - −0.8 GC 28.4 - 36.1 1.4 - 6.3 O 93.4 - 95.6 1.6 - 0.6 Minor 42 M 93.4 - 95.6 1.7 - 0.6 GC 93.4 - 88.9 1.5 - −5.9 O 57.8 - 59.3 1.6 - −0.2 Minor 56 M 57.8 - 59.4 1.7 - −0.1 GC 57.8 - 59.3 1.6 - −0.1 O 43.9 - 53.8 1.4 - 8.5 Minor 70 M 43.9 - 57.9 1.6 - 12.5 GC 43.9 - 49.8 1.8 - 4.1 O 85.3 - 92.3 1.5 - 5.5 Minor 84 M 85.3 - 94.9 1.9 - 7.8 GC 85.3 - 88.5 2.0 - 1.2 O 57.8 - 65.8 1.5 - 6.5 Minor 98 M 57.8 - 49.9 1.2 - −9.1 GC 57.8 - 58.9 2.5 - −1.5 1Precipitation; 2Irrigation; 3Runoff; 4Evapotranspiration; 5Drainage; 6Soil Water Storage. dication that there was an increase in the amount of water stored in the root zone of the maize genotypes but with different magnitudes. The situation was different during the minor cropping season, but rather the balance of soil water in the root zone of the maize genotypes was positive at 70 and 84 DAE, with the root zone of Mamaba re- cording the highest change in stored soil water as against that of Golden Crystal which had the lowest change in stored soil water. The water balance at 56 DAE during the minor cropping season was negative for the maize geno- types, which indicates a decrease in stored soil water. On the other hand, a positive soil water balance was observed in the root zone of Obatanpa and Mamaba during the minor cropping season at 42 DAE whereas the root zone of Golden Crystal had a negative water balance. Finally, at crop maturity, during the major cropping season a negative balance in soil water was estimated for the maize genotype under a precipitation of 103.7 mm. High amount of water lost from the root zone of the maize genotypes, with the root zone of Obatanpa losing the highest amount of water while root zones of Mamaba and Golden Crystal lost similar amount of water (Tables 3 and 4). For the minor cropping season, a positive balance in soil water was observed for Obatanpa, which was 0.92 mm greater than that recorded at 84 DAE. This indicates that Obatanpa maize genotype was still taking up soil water as a result of capillary rise of water below its root zone to satisfy evaporative and transpiration demand. On the other hand, a negative balance in soil water storage was recorded in the root zone of Mamaba and Golden Copyright © 2012 SciRes. OJSS
 Field Assessment of Soil Water Storage and Actual Evapotranspiration of Rainfed Maize (Zea mays L.) Genotypes in a Coastal Savannah Environment 219 Crystal maize genotypes. 3.3. Depth of Water Stored in Soil The depth of soil water stored for plant use peaked at 28 DAE, being 239.3, 228.4 and 237.3 mm, respectively, in the root zone of Obatanpa, Mamaba and Golden Crystal. This high amount of stored soil water is attributed to pre- cipitation events that occurred within 28 DAE. Mamaba used about 14.9 % of the stored soil water for evapo- transpiration followed by Golden Crystal and Obatanpa which used 11.6 % and 7.6 %, respectively, at 28 DAE. The depth of soil water stored dropped from 28 DAE to 84 DAE during the major cropping season (Figure 2(a)) but increased again from 84 to 98 DAE when crops were already matured. The decline in the stored soil water in the plant root zone could be attributed to decrease in pre- cipitation and increase in AET as crop growth and deve- lopment progressed with time. Similar amount of stored soil water in the root zones of the maize genotypes was observed at tasseling and silking stages of the crops (56 DAE). 29.92% of the stored soil water was absorbed by the roots of Obatanpa for plant evapotranspiration, with Golden Crystal and Mamaba following with 29.37% and 28.68% respectively. The use of stored soil water peaked at 84 DAE among the maize genotypes with Obatanpa taking up the highest (55.6%) amount of stored soil water followed by Golden Crystal (53.3%) and Mamaba (51.5%) for AET. There was a constant depth of water stored in the plant root zones of maize genotypes from 14 DAE to 56 DAE during the minor cropping season, which declined from 56 to 84 DAE but increased from 84 DAE to 98 DAE (Figure 2(b)). The highest depth of water stored during the minor cropping season occurred at 98 DAE when maize crops were already matured. These high values of stored soil water (232.92 mm for Obatanpa, 231.22 mm for Mamaba and 235.44 mm for Golden Crystal) during the minor cropping season were as a result of the high amount of precipitation (Table 4). Stored soil water was the highest for Golden Crystal at 84 DAE during the minor cropping season as against similar levels 199.2 mm and 195.7 mm for Obatanpa and Mamaba, respectively. Golden Crystal used 16.5% of stored soil water at 28 DAE for AET during the minor cropping season as against Mamaba and Oba- tanpa which used 14.0% and 13.8%, respectively. Further- more, the use of stored soil water peaked at 84 DAE during the minor cropping season, with 48.5% of stored soil water being taken up by Mamaba followed by 46.4% by Obatanpa and 43.2% by Golden Crystal during the major cropping season. 3.4. Rate of Actual Evapotranspiration Statistically, the rate of AET during the major and minor 250 200 150 Obatanpa Mamaba Golden Crystal 0 20 40 60 80 100 120 Days after emergence Depth of water stored (mm) (a) 250 200 150 Obatanpa Mamaba Golden Crystal 0 20 40 60 80 100 120 Days after emergence Depth of water stored (mm) (b) Figure 2. Depth of water stored within the rooting depth of the three maize genotypes during (a) major (b) minor crop- ping seasons. cropping seasons was similar among the maize genotypes. The rates of AET for the major cropping season for Obatanpa, Mamaba, and Golden Crystal were signify- cantly (p ≤ 0.05) higher than those of the minor cropping season. For Obatanpa, the rate of AET ranged between 1.30 and 7.33 mm·day−1, averaging 4.32 mm·day−1 during the major cropping season and also ranged between 1.16 and 6.60 mm·day−1 with an average value of 3.88 mm·day−1 during the minor cropping season. In the case of Mamaba the rate of AET ranged between 1.63 and 7.28 mm·day−1, averaging 4.46 mm·day−1 during the major cropping season as against the range of 1.13 - 6.78 mm·day−1 with an average value of 4.00 mm·day−1 during the minor cropping season. Similarly, the rate of AET for Golden Crystal ranged between 1.47 and 7.46 mm·day−1 with an average value of 4.47 mm·day−1 during the major cropping season as against the rate of AET which ranged between 1.11 and 6.32 mm·day−1 with an average of 3.72 mm·day−1 during the minor cropping season. At the early vegetative stage of the maize crops (14 and 28 DAE), AET values were low (Tables 3 and 4), in re- Copyright © 2012 SciRes. OJSS
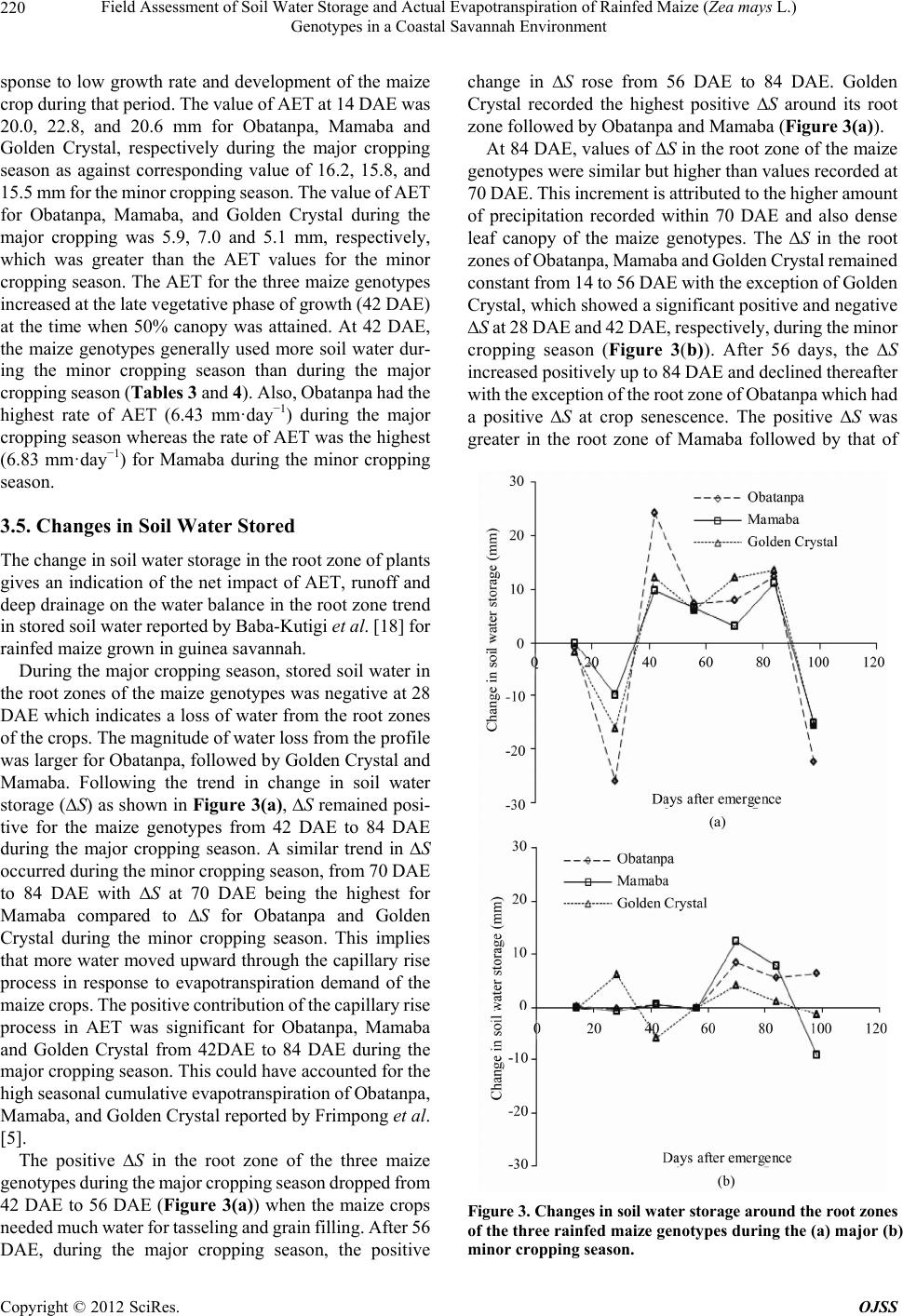 Field Assessment of Soil Water Storage and Actual Evapotranspiration of Rainfed Maize (Zea mays L.) Genotypes in a Coastal Savannah Environment 220 sponse to low growth rate and development of the maize crop during that period. The value of AET at 14 DAE was 20.0, 22.8, and 20.6 mm for Obatanpa, Mamaba and Golden Crystal, respectively during the major cropping season as against corresponding value of 16.2, 15.8, and 15.5 mm for the minor cropping season. The value of AET for Obatanpa, Mamaba, and Golden Crystal during the major cropping was 5.9, 7.0 and 5.1 mm, respectively, which was greater than the AET values for the minor cropping season. The AET for the three maize genotypes increased at the late vegetative phase of growth (42 DAE) at the time when 50% canopy was attained. At 42 DAE, the maize genotypes generally used more soil water dur- ing the minor cropping season than during the major cropping season (Tables 3 and 4). Also, Obatanpa had the highest rate of AET (6.43 mm·day−1) during the major cropping season whereas the rate of AET was the highest (6.83 mm·day−1) for Mamaba during the minor cropping season. 3.5. Changes in Soil Water Stored The change in soil water storage in the root zone of plants gives an indication of the net impact of AET, runoff and deep drainage on the water balance in the root zone trend in stored soil water reported by Baba-Kutigi et al. [18] for rainfed maize grown in guinea savannah. During the major cropping season, stored soil water in the root zones of the maize genotypes was negative at 28 DAE which indicates a loss of water from the root zones of the crops. The magnitude of water loss from the profile was larger for Obatanpa, followed by Golden Crystal and Mamaba. Following the trend in change in soil water storage (∆S) as shown in Figure 3(a), ∆S remained posi- tive for the maize genotypes from 42 DAE to 84 DAE during the major cropping season. A similar trend in ∆S occurred during the minor cropping season, from 70 DAE to 84 DAE with ∆S at 70 DAE being the highest for Mamaba compared to ∆S for Obatanpa and Golden Crystal during the minor cropping season. This implies that more water moved upward through the capillary rise process in response to evapotranspiration demand of the maize crops. The positive contribution of the capillary rise process in AET was significant for Obatanpa, Mamaba and Golden Crystal from 42DAE to 84 DAE during the major cropping season. This could have accounted for the high seasonal cumulative evapotranspiration of Obatanpa, Mamaba, and Golden Crystal reported by Frimpong et al. [5]. The positive ∆S in the root zone of the three maize genotypes during the major cropping season dropped from 42 DAE to 56 DAE (Figure 3(a)) when the maize crops needed much water for tasseling and grain filling. After 56 DAE, during the major cropping season, the positive change in ∆S rose from 56 DAE to 84 DAE. Golden Crystal recorded the highest positive ∆S around its root zone followed by Obatanpa and Mamaba (Figure 3(a)). At 84 DAE, values of ∆S in the root zone of the maize genotypes were similar but higher than values recorded at 70 DAE. This increment is attributed to the higher amount of precipitation recorded within 70 DAE and also dense leaf canopy of the maize genotypes. The ∆S in the root zones of Obatanpa, Mamaba and Golden Crystal remained constant from 14 to 56 DAE with the exception of Golden Crystal, which showed a significant positive and negative ∆S at 28 DAE and 42 DAE, respectively, during the minor cropping season (Figure 3(b)). After 56 days, the ∆S increased positively up to 84 DAE and declined thereafter with the exception of the root zone of Obatanpa which had a positive ∆S at crop senescence. The positive ∆S was greater in the root zone of Mamaba followed by that of Figure 3. Changes in soil water storage around the root zones of the three rainfed maize genotypes during the (a) major (b) minor cropping season. Copyright © 2012 SciRes. OJSS
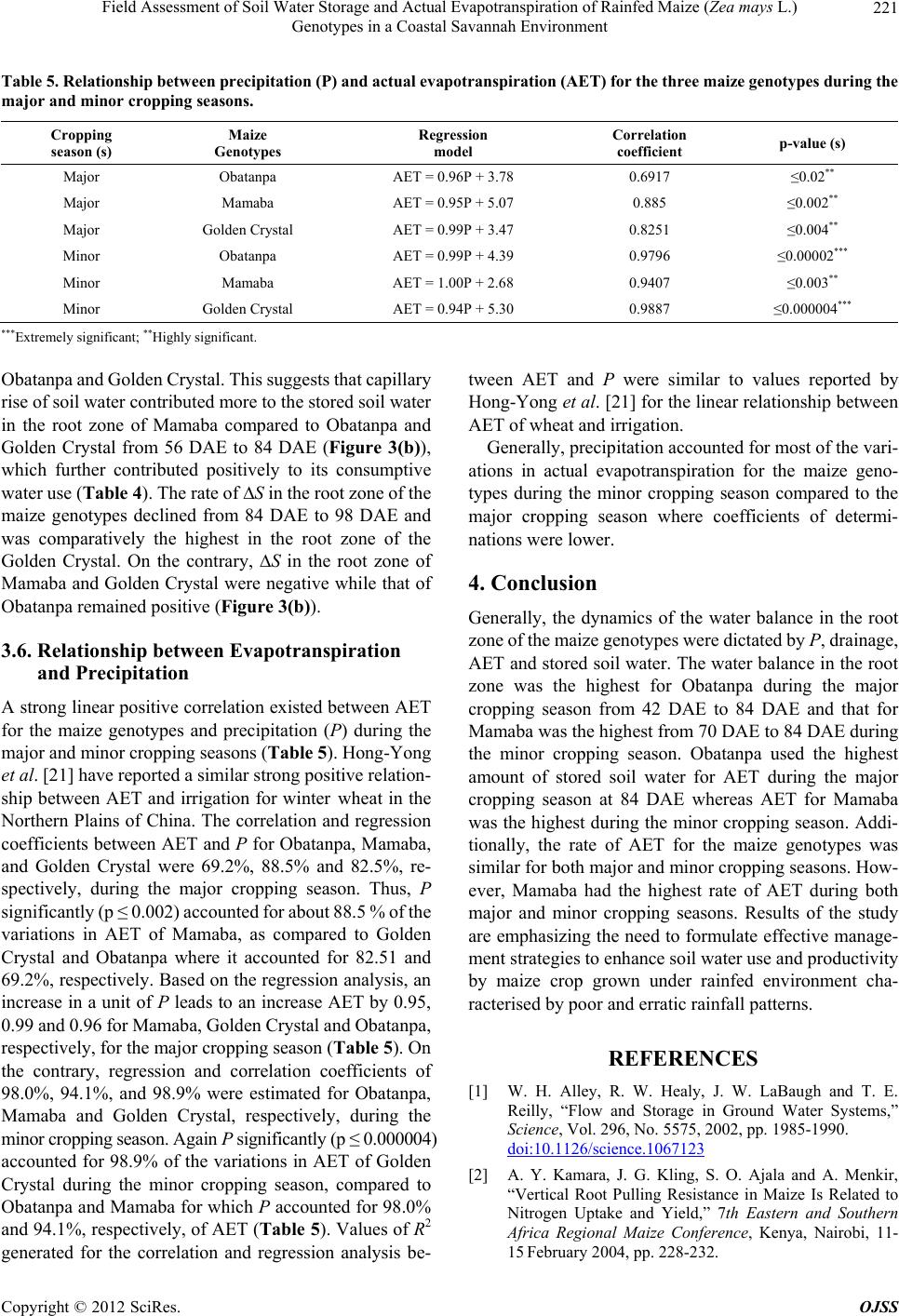 Field Assessment of Soil Water Storage and Actual Evapotranspiration of Rainfed Maize (Zea mays L.) Genotypes in a Coastal Savannah Environment Copyright © 2012 SciRes. OJSS 221 Table 5. Relationship between precipitation (P) and actual evapotranspiration (AET) for the three maize genotypes during the major and minor cropping seasons. Cropping season (s) Maize Genotypes Regression model Correlation coefficient p-value (s) Major Obatanpa AET = 0.96P + 3.78 0.6917 ≤0.02** Major Mamaba AET = 0.95P + 5.07 0.885 ≤0.002** Major Golden Crystal AET = 0.99P + 3.47 0.8251 ≤0.004** Minor Obatanpa AET = 0.99P + 4.39 0.9796 ≤0.00002*** Minor Mamaba AET = 1.00P + 2.68 0.9407 ≤0.003** Minor Golden Crystal AET = 0.94P + 5.30 0.9887 ≤0.000004*** ***Extremely significant; **Highly significant. Obatanpa and Golden Crystal. This suggests that capillary rise of soil water contributed more to the stored soil water in the root zone of Mamaba compared to Obatanpa and Golden Crystal from 56 DAE to 84 DAE (Figure 3(b)), which further contributed positively to its consumptive water use (Table 4). The rate of ∆S in the root zone of the maize genotypes declined from 84 DAE to 98 DAE and was comparatively the highest in the root zone of the Golden Crystal. On the contrary, ∆S in the root zone of Mamaba and Golden Crystal were negative while that of Obatanpa remained positive (Figure 3(b)). 3.6. Relationship between Evapotranspiration and Precipitation A strong linear positive correlation existed between AET for the maize genotypes and precipitation (P) during the major and minor cropping seasons (Table 5). Hong-Yong et al. [21] have reported a similar strong positive relation- ship between AET and irrigation for winter wheat in the Northern Plains of China. The correlation and regression coefficients between AET and P for Obatanpa, Mamaba, and Golden Crystal were 69.2%, 88.5% and 82.5%, re- spectively, during the major cropping season. Thus, P significantly (p ≤ 0.002) accounted for about 88.5 % of the variations in AET of Mamaba, as compared to Golden Crystal and Obatanpa where it accounted for 82.51 and 69.2%, respectively. Based on the regression analysis, an increase in a unit of P leads to an increase AET by 0.95, 0.99 and 0.96 for Mamaba, Golden Crystal and Obatanpa, respectively, for the major cropping season (Table 5). On the contrary, regression and correlation coefficients of 98.0%, 94.1%, and 98.9% were estimated for Obatanpa, Mamaba and Golden Crystal, respectively, during the minor cropping season. Again P significantly (p ≤ 0.000004) accounted for 98.9% of the variations in AET of Golden Crystal during the minor cropping season, compared to Obatanpa and Mamaba for which P accounted for 98.0% and 94.1%, respectively, of AET (Table 5). Values of R2 generated for the correlation and regression analysis be- tween AET and P were similar to values reported by Hong-Yong et al. [21] for the linear relationship between AET of wheat and irrigation. Generally, precipitation accounted for most of the vari- ations in actual evapotranspiration for the maize geno- types during the minor cropping season compared to the major cropping season where coefficients of determi- nations were lower. 4. Conclusion Generally, the dynamics of the water balance in the root zone of the maize genotypes were dictated by P, drainage, AET and stored soil water. The water balance in the root zone was the highest for Obatanpa during the major cropping season from 42 DAE to 84 DAE and that for Mamaba was the highest from 70 DAE to 84 DAE during the minor cropping season. Obatanpa used the highest amount of stored soil water for AET during the major cropping season at 84 DAE whereas AET for Mamaba was the highest during the minor cropping season. Addi- tionally, the rate of AET for the maize genotypes was similar for both major and minor cropping seasons. How- ever, Mamaba had the highest rate of AET during both major and minor cropping seasons. Results of the study are emphasizing the need to formulate effective manage- ment strategies to enhance soil water use and productivity by maize crop grown under rainfed environment cha- racterised by poor and erratic rainfall patterns. REFERENCES [1] W. H. Alley, R. W. Healy, J. W. LaBaugh and T. E. Reilly, “Flow and Storage in Ground Water Systems,” Science, Vol. 296, No. 5575, 2002, pp. 1985-1990. doi:10.1126/science.1067123 [2] A. Y. Kamara, J. G. Kling, S. O. Ajala and A. Menkir, “Vertical Root Pulling Resistance in Maize Is Related to Nitrogen Uptake and Yield,” 7th Eastern and Southern Africa Regional Maize Conference, Kenya, Nairobi, 11- 15 February 2004, pp. 228-232.
 Field Assessment of Soil Water Storage and Actual Evapotranspiration of Rainfed Maize (Zea mays L.) Genotypes in a Coastal Savannah Environment 222 [3] A. Mando, “The Impact of Termites and Mulch on the Water Balance of Crusted Sahelien Soil,” Soil Technology, Vol. 11, No. 2, 1997, pp. 121-138. doi:10.1016/S0933-3630(97)00003-2 [4] J. L. Araus, G. A. Slafer and M. P. Reynolds, “Plant Breeding and Drought in C3 Cereals: What Should We Breed for?” Annals of Botany, Vol. 89, No. 7, 2002, pp. 925-940. doi:10.1093/aob/mcf049 [5] J. O. Frimpong, H. M. Amoatey, E. O. Ayeh and D. K. Asare, “Productivity and Soil Water Use by Rainfed Maize Genotypes in a Coastal Savannah Environment,” International Agrophysics, Vol. 25, No. 2, 2011, pp. 123-129. [6] N. Hatibu, M. D. B. Young, J. W. Gowing, H. F. Mahoo and O. B. Mzirai, “Developing Improved Dry Land Crop- ping Systems for Maize in Semi-Arid Tanzania Part 1,” Journal of Experimental Agriculture, Vol. 39, No. 3, 2003, pp. 279-292. doi:10.1017/S0014479703001285 [7] S. P. Wani, P. Patrick, T. K. Sreedevi, H. P. Singh and P. Singh, “Efficient Management of Rainwater for Increased Crop Productivity and Groundwater Recharge in Asia” CABI Publishing and International Water Management Institute, Wallingford, 2003, p. 56. [8] A. A. Dankyi, P. Y. K. Sallah, A. Adu-Appiah and A. Gyamera-Antwi, “Determination of the Adoption of Quality Protein Maize, Obatanpa, in Southern Ghana— Logistic Regression Analysis.” Paper presented at the 5th West and Central Africa Regional Maize Workshop, IITA, Cotonou, 2-7 May 2005, pp. 6-13. [9] M. L. Morris, R. Tripp and A. A. Dankyi, “Adoption and Impacts of Improved Maize Production Technology: A Case Study of the Ghana Grains Development Project,” Economics Program Paper, D. F. CIMMYT, Mexico, 1999, pp. 3-41. [10] FAO/UNESCO, “Soil Map of the World, Revised Leg- end,” World Resources Report 60, FAO, Rome, 1994, p. 146. [11] International Atomic Energy Agency, “Neutron and Gamma Probes: Their Use in Agronomy,” Reports Series No. 16, IAEA, Vienna, 2002. [12] J. T. Musick, O. R. Jones, B. A. Stemart and D. A. Dusek, “Water-Yield Relationships for Irrigated and Dry Land Wheat in the US Southern Plains,” Agronomy Journal— Abstract, Vol. 86, No. 6, 1994, pp. 980-986. doi:10.2134/agronj1994.00021962008600060010x [13] F. R. Li, S. L. Zhao and G. T. Geballe, “Water Use Pat- terns and Agronomic Performance for Some Cropping Systems with and without Fallow Crops in a Semi-Arid Environment of Northwest China,” Agriculture, Ecosys- tems & Environment, Vol. 79, No. 2-3, 2000, pp. 129-142. doi:10.1016/S0167-8809(99)00149-8 [14] F. M. Li, S. L. Liu and S. Q. Li, “Effects of Early Soil Water Distribution on the Dry Matter Partition between Roots and Shoots of Winter Wheat,” Agricultural Water Management, Vol. 49, No. 3, 2001, pp. 163-171. doi:10.1016/S0378-3774(01)00088-9 [15] A. Sadeh and I. Ravina, “Relationships between Yield and Irrigation with Low-Quantity Water—A System Ap- proach,” Agricultural Systems, Vol. 64, No. 2, 2000, pp. 99-113. doi:10.1016/S0308-521X(00)00016-0 [16] H. X. Wang, L. Zhang, W. R. Dawes and C. M. Liu, “Im- proving Water Use Efficiency of Irrigated Crops in the North China Plain-Measurements and Modelling,” Agri- cultural Water Management, Vol. 48, No. 2, 2001, pp. 151-167. doi:10.1016/S0378-3774(00)00118-9 [17] G. Campbell, “Transport Models for Soil-Plant Systems,” Elsevier, Amsterdam, 1985. [18] A. N. Baba-Kutigi, L. A. Dim, A. C. Odunze and I. M. Umar, “Soil Water Balance Approach in the Root Zone of Maize (95 TZEE-Y) Using Capacitance Probe (Diviner 2000) in Northern Guinea Savannah of Nigeria,” Bayero Journal of Pure and Applied Sciences, Vol. 3, No. 2, 2010, pp. 87-90. [19] S. Z. Kang, Y. Zhang, Y. L. Liang, X. T. Hu, H. J. Cai and B. J. Gu, “Effects of Limited Irrigation on Yield and Water Use Efficiency of Winter Wheat in the Loess Pla- teau of China,” Agricultural Water Management, Vol. 55, No. 3, 2002, pp. 203-216. doi:10.1016/S0378-3774(01)00180-9 [20] Y. Zhang, E. Kendy, Y. Qiang, L. Changming, S. Yanjun and S. Hongyang, “Effect of Soil Water Deficit on Evapotranspiration, Crop Yield, and Water Use Effi- ciency in the North China Plain,” Agricultural Water Management, Vol. 64, No. 2, 2004, pp. 107-122. doi:10.1016/S0378-3774(03)00201-4 [21] H.-Y. Sun, C.-M. Liu, X.-Y. Zhang, Y.-J. Shen and Y.-Q. Zhang, “Effects of Irrigation on Water Balance, Yield and WUE of Winter Wheat in the North China Plain,” Agri- cultural Water Management, Vol. 85, No. 1-2, 2006, pp. 211-218. doi:10.1016/j.agwat.2006.04.008 Copyright © 2012 SciRes. OJSS
|