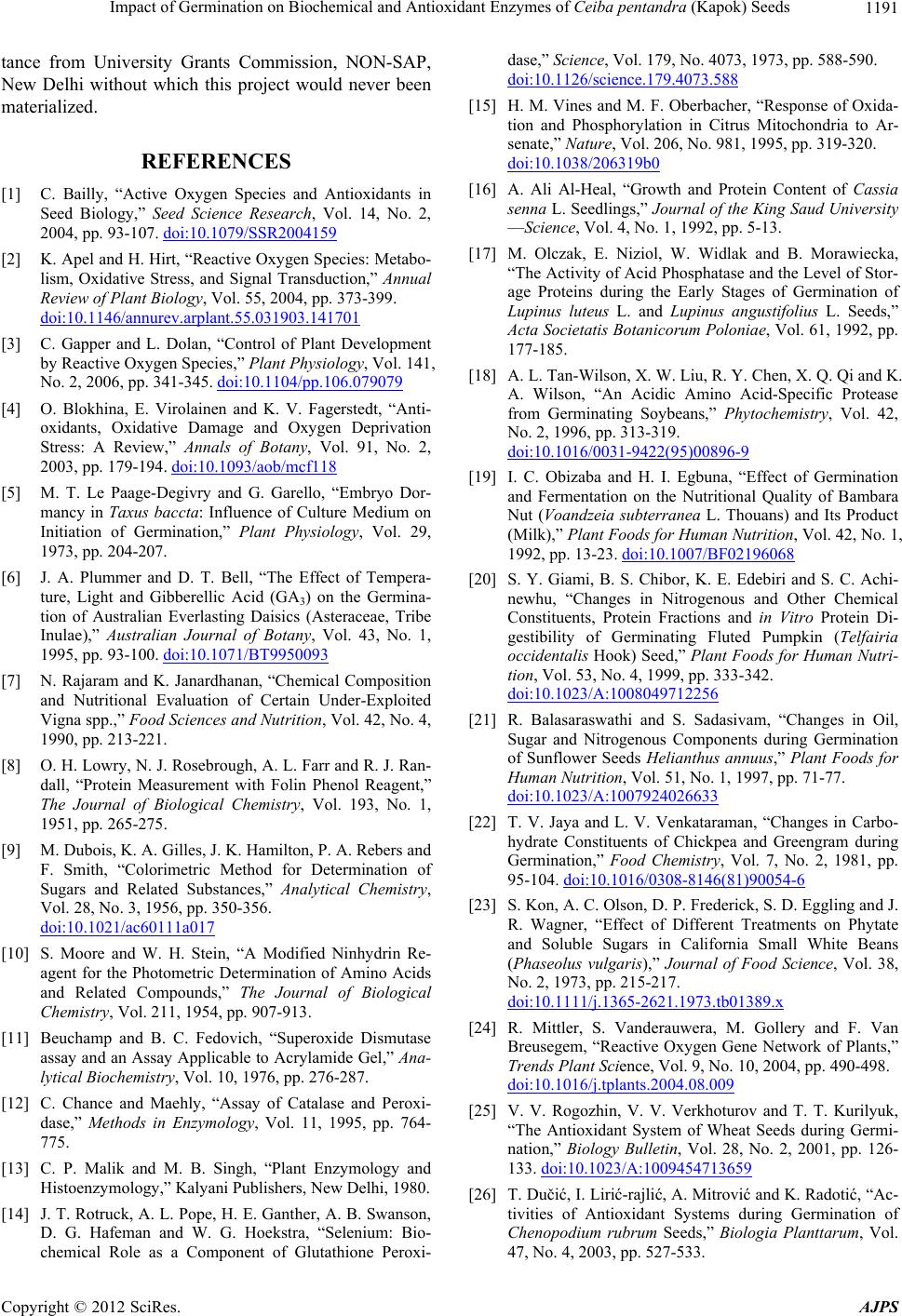
Impact of Germination on Biochemical and Antioxidant Enzymes of Ceiba pentandra (Kapok) Seeds 1191
tance from University Grants Commission, NON-SAP,
New Delhi without which this project would never been
materialized.
REFERENCES
[1] C. Bailly, “Active Oxygen Species and Antioxidants in
Seed Biology,” Seed Science Research, Vol. 14, No. 2,
2004, pp. 93-107. doi:10.1079/SSR2004159
[2] K. Apel and H. Hirt, “Reactive Oxygen Species: Metabo-
lism, Oxidative Stress, and Signal Transduction,” Annual
Review of Plant Biology, Vol. 55, 2004, pp. 373-399.
doi:10.1146/annurev.arplant.55.031903.141701
[3] C. Gapper and L. Dolan, “Control of Plant Development
by Reactive Oxygen Species,” Plant Physiology, Vol. 141,
No. 2, 2006, pp. 341-345. doi:10.1104/pp.106.079079
[4] O. Blokhina, E. Virolainen and K. V. Fagerstedt, “Anti-
oxidants, Oxidative Damage and Oxygen Deprivation
Stress: A Review,” Annals of Botany, Vol. 91, No. 2,
2003, pp. 179-194. doi:10.1093/aob/mcf118
[5] M. T. Le Paage-Degivry and G. Garello, “Embryo Dor-
mancy in Taxus baccta: Influence of Culture Medium on
Initiation of Germination,” Plant Physiology, Vol. 29,
1973, pp. 204-207.
[6] J. A. Plummer and D. T. Bell, “The Effect of Tempera-
ture, Light and Gibberellic Acid (GA3) on the Germina-
tion of Australian Everlasting Daisics (Asteraceae, Tribe
Inulae),” Australian Journal of Botany, Vol. 43, No. 1,
1995, pp. 93-100. doi:10.1071/BT9950093
[7] N. Rajaram and K. Janardhanan, “Chemical Composition
and Nutritional Evaluation of Certain Under-Exploited
Vigna spp.,” Food Sciences and Nutrition, Vol. 42, No. 4,
1990, pp. 213-221.
[8] O. H. Lowry, N. J. Rosebrough, A. L. Farr and R. J. Ran-
dall, “Protein Measurement with Folin Phenol Reagent,”
The Journal of Biological Chemistry, Vol. 193, No. 1,
1951, pp. 265-275.
[9] M. Dubois, K. A. Gilles, J. K. Hamilton, P. A. Rebers and
F. Smith, “Colorimetric Method for Determination of
Sugars and Related Substances,” Analytical Chemistry,
Vol. 28, No. 3, 1956, pp. 350-356.
doi:10.1021/ac60111a017
[10] S. Moore and W. H. Stein, “A Modified Ninhydrin Re-
agent for the Photometric Determination of Amino Acids
and Related Compounds,” The Journal of Biological
Chemistry, Vol. 211, 1954, pp. 907-913.
[11] Beuchamp and B. C. Fedovich, “Superoxide Dismutase
assay and an Assay Applicable to Acrylamide Gel,” Ana-
lytical Biochemistry, Vol. 10, 1976, pp. 276-287.
[12] C. Chance and Maehly, “Assay of Catalase and Peroxi-
dase,” Methods in Enzymology, Vol. 11, 1995, pp. 764-
775.
[13] C. P. Malik and M. B. Singh, “Plant Enzymology and
Histoenzymology,” Kalyani Publishers, New Delhi, 1980.
[14] J. T. Rotruck, A. L. Pope, H. E. Ganther, A. B. Swanson,
D. G. Hafeman and W. G. Hoekstra, “Selenium: Bio-
chemical Role as a Component of Glutathione Peroxi-
dase,” Science, Vol. 179, No. 4073, 1973, pp. 588-590.
doi:10.1126/science.179.4073.588
[15] H. M. Vines and M. F. Oberbacher, “Response of Oxida-
tion and Phosphorylation in Citrus Mitochondria to Ar-
senate,” Nature, Vol. 206, No. 981, 1995, pp. 319-320.
doi:10.1038/206319b0
[16] A. Ali Al-Heal, “Growth and Protein Content of Cassia
senna L. Seedlings,” Journal of the King Saud University
—Science, Vol. 4, No. 1, 1992, pp. 5-13.
[17] M. Olczak, E. Niziol, W. Widlak and B. Morawiecka,
“The Activity of Acid Phosphatase and the Level of Stor-
age Proteins during the Early Stages of Germination of
Lupinus luteus L. and Lupinus angustifolius L. Seeds,”
Acta Societatis Botanicorum Poloniae, Vol. 61, 1992, pp.
177-185.
[18] A. L. Tan-Wilson, X. W. Liu, R. Y. Chen, X. Q. Qi and K.
A. Wilson, “An Acidic Amino Acid-Specific Protease
from Germinating Soybeans,” Phytochemistry, Vol. 42,
No. 2, 1996, pp. 313-319.
doi:10.1016/0031-9422(95)00896-9
[19] I. C. Obizaba and H. I. Egbuna, “Effect of Germination
and Fermentation on the Nutritional Quality of Bambara
Nut (Voandzeia subterranea L. Thouans) and Its Product
(Milk),” Plant Foods for Human Nutrition, Vol. 42, No. 1,
1992, pp. 13-23. doi:10.1007/BF02196068
[20] S. Y. Giami, B. S. Chibor, K. E. Edebiri and S. C. Achi-
newhu, “Changes in Nitrogenous and Other Chemical
Constituents, Protein Fractions and in Vitro Protein Di-
gestibility of Germinating Fluted Pumpkin (Telfairia
occidentalis Hook) Seed,” Plant Foods for Human Nutri-
tion, Vol. 53, No. 4, 1999, pp. 333-342.
doi:10.1023/A:1008049712256
[21] R. Balasaraswathi and S. Sadasivam, “Changes in Oil,
Sugar and Nitrogenous Components during Germination
of Sunflower Seeds Helianthus annuus,” Plant Foods for
Human Nutrition, Vol. 51, No. 1, 1997, pp. 71-77.
doi:10.1023/A:1007924026633
[22] T. V. Jaya and L. V. Venkataraman, “Changes in Carbo-
hydrate Constituents of Chickpea and Greengram during
Germination,” Food Chemistry, Vol. 7, No. 2, 1981, pp.
95-104. doi:10.1016/0308-8146(81)90054-6
[23] S. Kon, A. C. Olson, D. P. Frederick, S. D. Eggling and J.
R. Wagner, “Effect of Different Treatments on Phytate
and Soluble Sugars in California Small White Beans
(Phaseolus vulgaris),” Journal of Food Science, Vol. 38,
No. 2, 1973, pp. 215-217.
doi:10.1111/j.1365-2621.1973.tb01389.x
[24] R. Mittler, S. Vanderauwera, M. Gollery and F. Van
Breusegem, “Reactive Oxygen Gene Network of Plants,”
Trends Plant Science, Vol. 9, No. 10, 2004, pp. 490-498.
doi:10.1016/j.tplants.2004.08.009
[25] V. V. Rogozhin, V. V. Verkhoturov and T. T. Kurilyuk,
“The Antioxidant System of Wheat Seeds during Germi-
nation,” Biology Bulletin, Vol. 28, No. 2, 2001, pp. 126-
133. doi:10.1023/A:1009454713659
[26] T. Dučić, I. Lirić-rajlić, A. Mitrović and K. Radotić, “Ac-
tivities of Antioxidant Systems during Germination of
Chenopodium rubrum Seeds,” Biologia Planttarum, Vol.
47, No. 4, 2003, pp. 527-533.
Copyright © 2012 SciRes. AJPS