 International Journal of Clinical Medicine, 2012, 3, 344-351 http://dx.doi.org/10.4236/ijcm.2012.35067 Published Online September 2012 (http://www.SciRP.org/journal/ijcm) 1 Evaluation of the Anti-Trypanosoma cruzi Effects of the Antipsychotic Drug Levomepromazine Inga Eva Stik Lange, Elisabeth Mieko Furusho Pral, Anahí Magdaleno, Ariel Mariano Silber* Departamento de Parasitologia, Instituto de Ciências, Universidade de São Paulo, São Paulo, Brazil. Email: *asilber@usp.br Received May 22nd, 2012; revised June 24th, 2012; accepted July 15th, 2012 ABSTRACT Chagas disease, caused by the protozoan Trypanosoma cruzi, is a relevant parasitic disease in the Americas. Current chemotherapy relies on Nifurtimox and Benznidazole, which present serious drawbacks, including high toxicity, low efficiency and the emergence of resistant strains. In the present work, the perspectives of levomepromazine, a tricyclic compound belonging to the family of phenotiazines with well-known properties as antipsychotics were evaluated as a potential anti-T. cruzi drug. We show that this drug is able to inhibit the proliferation of epimastigotes (IC50 = 0.41 ± 0.01 mM) and to interfere with the infection of the host cells (IC50 = 0.34 ± 0.01 mM). Interestingly, the treatment with levomepromazine affected the ability of metabolites such as glucose, proline and glutamate to fuel the recovery of epi- mastigotes after being submitted to metabolic stress. These findings prompt levomepromazine as a promising leader drug to obtain new trypanocidal activities. Keywords: Chagas Disease; Phenothiazines; Trypanothione Reductase; Amino Acid Metabolism; Chemotherapy 1. Introduction Trypanosoma cruzi, the causative agent for Chagas dis- ease, a relevant health problem in most American coun- tries, as it is broadly distributed from Mexico to southern Argentina and Chile, where it is endemic, with 10 - 12 million people infected and an estimated 25 million peo- ple at risk of acquiring the disease. Two drugs made available almost four decades ago for patients are still the only ones currently in use to treat Chagas disease: Nifur- timox (Nf) and Benznidazole (Bn). Both drugs are highly efficient in the acute phase of the disease; however, their efficiency to treat the chronic phase, when most patients are diagnosed, remains controversial. In addition, it is worth mentioning that other serious drawbacks have been reported for both drugs: their high toxicity, which in some cases forces the interruption of treatment, and evi- dence of emerging resistant strains. Taken together, these facts constitute major reasons to search for new thera- peutic alternatives [1]. Phenothiazines are a family of drugs related to the thi- azine class of heterocyclic compounds [2]. Phenothiazi- nes, which consist of a sulphur-containing tricyclic or- ganic compound with the formula S(C6H4)2NH, with side chains bound to the middle ring, have been commonly used psychotropic drugs since the 1950s. This family of compounds became well known and has been prescribed up to the present because of their antipsychotic effects [3]. Their action as dopamine blockers at D2 receptors and their neuroleptic activity has been well documented in the literature [4-7]. More recently, a large variety of structurally modified versions of these drugs has been introduced, broadening the spectrum of their targets. In this sense, papers published in the last twenty years showed antitumoural, antimicrobial, antiparasitic and anthelmintic activities for phenothiazine derivatives (re- viewed in [2]). The biological activities identified for members with different substitutions at different posi- tions, mainly 2 and 10, were particularly interesting to us. For example, it was shown that compounds having a methyl-thio substituent at position 10 and a fluorine sub- stituent at position 2 (triflupromazine) have interesting antimicrobial properties [2]. Variants of these drugs, such as triflupromazine and chlorpromazine, have been active against pathogenic amoebas like Naegleria fowleri, Acanthamoeba culbertsoni and A. polyphaga [8]. Despite unknown mechanisms of action, it was proposed that the drugs have an antagonistic effect on the amoebal calmodulins [9]. It was also proposed that chlorpromaz- ine, a lipophilic variant, could act by modifying the plasma membrane in some way, thereby diminishing the viability of these organisms. Chlorpromazine also showed inhibitory activity on the growth of Leishmania donovani promastigotes [10], the fungus Candida albicans [11], *Corresponding author. Copyright © 2012 SciRes. IJCM
 Evaluation of the Anti-Trypanosoma cruzi Effects of the Antipsychotic Drug Levomepromazine 345 the bacteria Staphylococcus aureus [12], Mycobacterium avium [13] and M. tuberculosis [14]. Shigella spp., Vi- brio cholera and V. parahaemolyticus are among the microorganisms reported to be sensitive to trifluop- erazine. In the present work, the possible trypanocidal or trypanostatic effect of Levomepromazine (LM), an anti- psychotic drug belonging to the family of phenothiazines, was investigated. LM was able to inhibit the growth of epimastigotes in a dose-dependent way, (IC50 = 0.41 mM) in the range of the doses reported in the literature for most of the tests with these drugs (which approximately range between 0.05 and 0.50 mM). Finally, it is worth mentioning that since the first half of past century, phe- nothiazines have been used safely in the treatment of human infection by the helminth Enterobius vermicularis [15]. In the present work, we report the anti-T. cruzi activity of levomepromazine. We also show its ability to syner- gise the deleterious effects of nutritional stress, meta- cyclogenesis and host-cell infection. 2. Materials and Methods 2.1. Reagents D-Glucose, L-proline, L-glutamate, rotenone, antimycin and 3-(4,5-dimethylthiazol-2-yl)-2,5-diphenyltetrazolium bromide (MTT) were obtained from Sigma (St. Louis, MO, USA). RPMI culture medium and foetal calf serum were obtained from Cultilab (Campinas, SP, Brazil). Levomepromazine (LM) (for chemical structure see Fig- ure 1) (Togrel®) was purchased from Ivvax (Argentina). All other reagents were from Amresco (Solon, OH, USA). 2.2. Cells and Parasites The Chinese Hamster Ovary cell line, CHO-K1, was rou- tinely cultivated in RPMI medium (Gibco BRL) supple- mented with 10% heat-inactivated foetal calf serum (FCS), 0.15% (w/v) NaHCO3, 100 units/mL penicillin and 100 μg/mL streptomycin at 37˚C in a humid atmos- phere containing 5% CO2. Epimastigotes of T. cruzi, CL strain, clone 14 [16], were maintained in the exponential Figure 1. Chemical structure of levomepromazine (LM), a phenothiazine derived compound. growth phase by subculturing every 48 h in liver infu- sion-tryptose (LIT) medium supplemented with 10% FCS at 28˚C [17]. Metacyclic trypomastigotes were ob- tained as previously described [18]. Briefly, 20 × 106 epimastigotes in the exponential growth phase were har- vested by centrifugation (5000 × g for 10 min), washed with PBS, and resuspended in TAU medium (190 mM NaCl, 17 mM KCl, 2 mM MgCl2, 2 mM CaCl2, 8 mM phosphate buffer pH 6.0). After 1-h incu bation at 28˚C, cultures were centrifuged (5000 × g for 10 min), resus- pended in TAU-3AG (TAU supplemented with 10 mM proline, 50 mM L-sodium glutamate, 2 mM L-sodium aspartate and 10 mM D-glucose) and incubated for 96 h at 28˚C. Culture-derived trypomastigotes were obtained by infection of CHO-K1 cells with trypomastigotes, as described previously [19]. Trypomastigotes were col- lected in the extracellular medium from five days post- infection. In all cases, parasites were counted in a Neubauer chamber. 2.3. Growth Inhibition Assays Epimastigote growth-inhibition assays were developed as previously described [20]. Briefly, epimastigotes in the exponential growth phase (approximately 5 × 107 cells/mL) were washed three times by centrifugation and resuspended in PBS and then cultured in fresh LIT me- dium supplemented with or without (controls) different concentrations of LM ranging from 0.2 to 1.1 mM at 28˚C. The assays were carried out in 96-well plates by inoculating epimastigotes in 200 μl of medium (2.5 × 106 cells/mL). Cell growth was estimated by absorbance readings at 620 nm every day for ten days. The absorb- ance was transformed into a cell density value (cells/mL) using a linear calibration equation previously obtained under the same conditions (R2 = 0.99551, p < 0.05). The concentration of LM that inhibited 50% of parasite growth (IC50) was determined in the exponential growth phase (fifth day) by adjusting the effect (growth inhibit- tion values) as a function of the drug concentration to a classical sigmoidal equation. As a cell growth inhibition control, growth curves in which 60 µM rotenone and 0.5 µM antimycin were added to the culture medium were run in parallel for all experiments. 2.4. The Effect of LM Growth Inhibition under Stress Conditions To analyse the combined effect of LM at different pH values and at different temperatures, the growth curves were generated as described above, by adjusting the LIT pH to the desired values or incubating the cultures at 28˚C (no stress), 33˚C or 37˚C. To evaluate the effect of LM combined with nutritional stress conditions, 2 × 107 parasites/mL were washed and resuspended in 0.2 mM Copyright © 2012 SciRes. IJCM
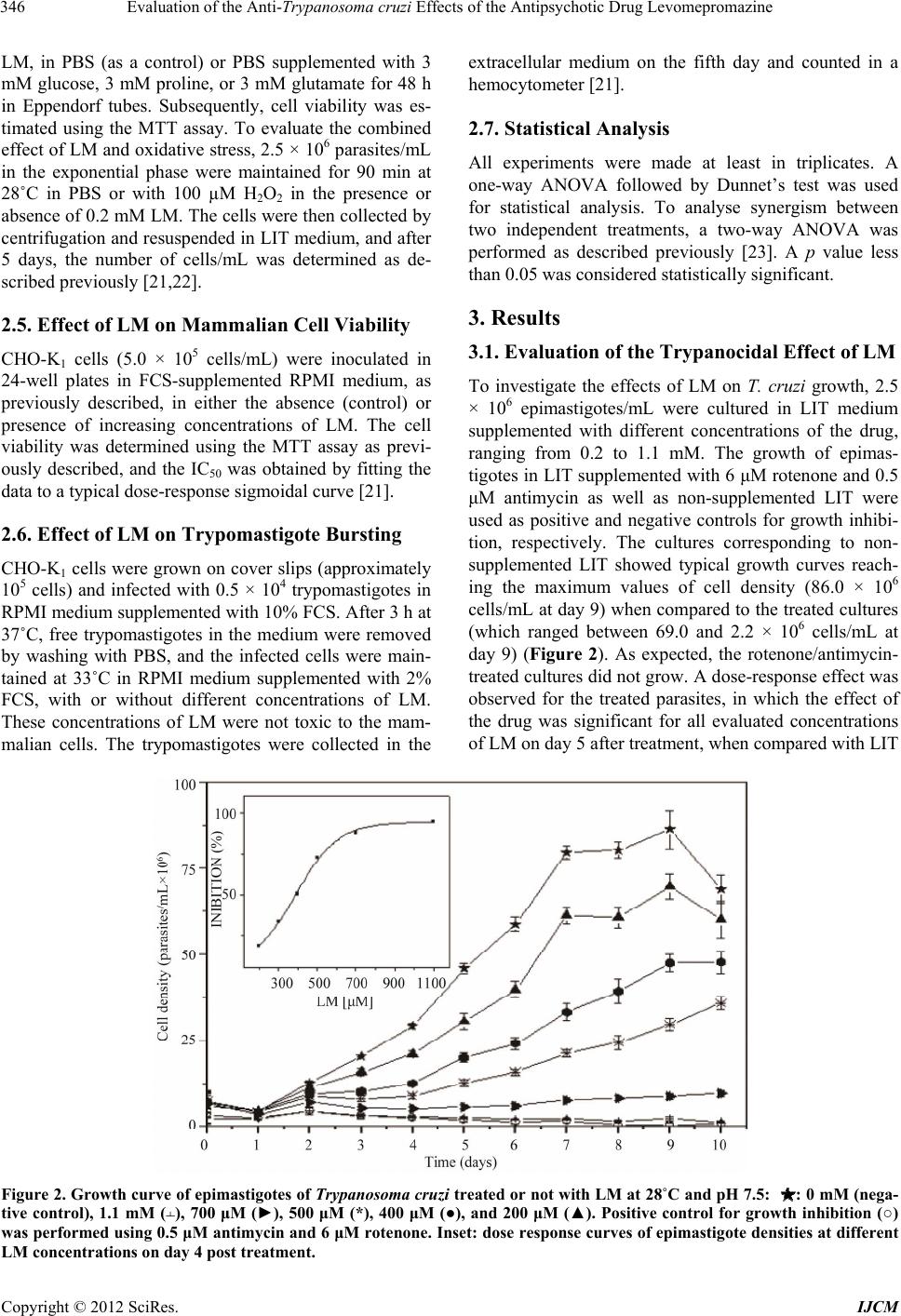 Evaluation of the Anti-Trypanosoma cruzi Effects of the Antipsychotic Drug Levomepromazine Copyright © 2012 SciRes. IJCM 346 LM, in PBS (as a control) or PBS supplemented with 3 mM glucose, 3 mM proline, or 3 mM glutamate for 48 h in Eppendorf tubes. Subsequently, cell viability was es- timated using the MTT assay. To evaluate the combined effect of LM and oxidative stress, 2.5 × 106 parasites/mL in the exponential phase were maintained for 90 min at 28˚C in PBS or with 100 µM H2O2 in the presence or absence of 0.2 mM LM. The cells were then collected by centrifugation and resuspended in LIT medium, and after 5 days, the number of cells/mL was determined as de- scribed previously [21,22]. extracellular medium on the fifth day and counted in a hemocytometer [21]. 2.7. Statistical Analysis All experiments were made at least in triplicates. A one-way ANOVA followed by Dunnet’s test was used for statistical analysis. To analyse synergism between two independent treatments, a two-way ANOVA was performed as described previously [23]. A p value less than 0.05 was considered statistically significant. 3. Results 2.5. Effect of LM on Mammalian Cell Viability 3.1. Evaluation of the Trypanocidal Effect of LM CHO-K1 cells (5.0 × 105 cells/mL) were inoculated in 24-well plates in FCS-supplemented RPMI medium, as previously described, in either the absence (control) or presence of increasing concentrations of LM. The cell viability was determined using the MTT assay as previ- ously described, and the IC50 was obtained by fitting the data to a typical dose-response sigmoidal curve [21]. To investigate the effects of LM on T. cruzi growth, 2.5 × 106 epimastigotes/mL were cultured in LIT medium supplemented with different concentrations of the drug, ranging from 0.2 to 1.1 mM. The growth of epimas- tigotes in LIT supplemented with 6 μM rotenone and 0.5 μM antimycin as well as non-supplemented LIT were used as positive and negative controls for growth inhibi- tion, respectively. The cultures corresponding to non- supplemented LIT showed typical growth curves reach- ing the maximum values of cell density (86.0 × 106 cells/mL at day 9) when compared to the treated cultures (which ranged between 69.0 and 2.2 × 106 cells/mL at day 9) (Figure 2). As expected, the rotenone/antimycin- treated cultures did not grow. A dose-response effect was observed for the treated parasites, in which the effect of the drug was significant for all evaluated concentrations of LM on day 5 after treatment, when compared with LIT 2.6. Effect of LM on Trypomastigote Bursting CHO-K1 cells were grown on cover slips (approximately 105 cells) and infected with 0.5 × 104 trypomastigotes in RPMI medium supplemented with 10% FCS. After 3 h at 37˚C, free trypomastigotes in the medium were removed by washing with PBS, and the infected cells were main- tained at 33˚C in RPMI medium supplemented with 2% FCS, with or without different concentrations of LM. These concentrations of LM were not toxic to the mam- malian cells. The trypomastigotes were collected in the Figure 2. Growth curve of epimastigotes of Trypanosoma cruzi treated or not with LM at 28˚C and pH 7.5: : 0 mM (nega- tive control), 1.1 mM (﬩), 700 μM (►), 500 μM (*), 400 μM (●), and 200 μM (▲). Positive control for growth inhibition (○) was performed using 0.5 μM antimycin and 6 μM rotenone. Inset: dose response curves of epimastigote densities at different LM concentrations on day 4 post treatment.
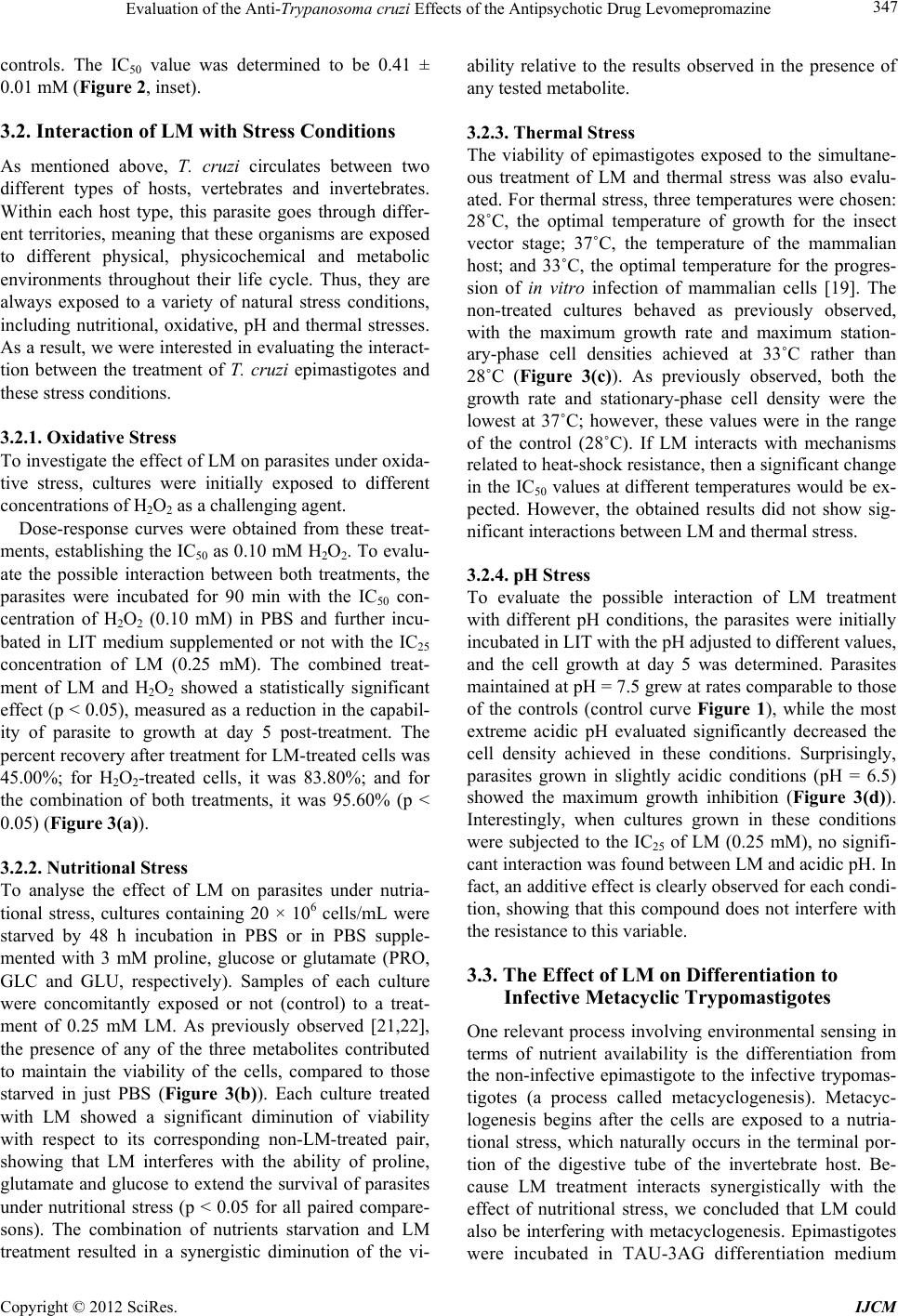 Evaluation of the Anti-Trypanosoma cruzi Effects of the Antipsychotic Drug Levomepromazine 347 controls. The IC50 value was determined to be 0.41 ± 0.01 mM (Figure 2, inset). 3.2. Interaction of LM with Stress Conditions As mentioned above, T. cruzi circulates between two different types of hosts, vertebrates and invertebrates. Within each host type, this parasite goes through differ- ent territories, meaning that these organisms are exposed to different physical, physicochemical and metabolic environments throughout their life cycle. Thus, they are always exposed to a variety of natural stress conditions, including nutritional, oxidative, pH and thermal stresses. As a result, we were interested in evaluating the interact- tion between the treatment of T. cruzi epimastigotes and these stress conditions. 3.2.1. Oxidative Stress To investigate the effect of LM on parasites under oxida- tive stress, cultures were initially exposed to different concentrations of H2O2 as a challenging agent. Dose-response curves were obtained from these treat- ments, establishing the IC50 as 0.10 mM H2O2. To evalu- ate the possible interaction between both treatments, the parasites were incubated for 90 min with the IC50 con- centration of H2O2 (0.10 mM) in PBS and further incu- bated in LIT medium supplemented or not with the IC25 concentration of LM (0.25 mM). The combined treat- ment of LM and H2O2 showed a statistically significant effect (p < 0.05), measured as a reduction in the capabil- ity of parasite to growth at day 5 post-treatment. The percent recovery after treatment for LM-treated cells was 45.00%; for H2O2-treated cells, it was 83.80%; and for the combination of both treatments, it was 95.60% (p < 0.05) (Figure 3(a)). 3.2.2. Nutritional Stress To analyse the effect of LM on parasites under nutria- tional stress, cultures containing 20 × 106 cells/mL were starved by 48 h incubation in PBS or in PBS supple- mented with 3 mM proline, glucose or glutamate (PRO, GLC and GLU, respectively). Samples of each culture were concomitantly exposed or not (control) to a treat- ment of 0.25 mM LM. As previously observed [21,22], the presence of any of the three metabolites contributed to maintain the viability of the cells, compared to those starved in just PBS (Figure 3(b)). Each culture treated with LM showed a significant diminution of viability with respect to its corresponding non-LM-treated pair, showing that LM interferes with the ability of proline, glutamate and glucose to extend the survival of parasites under nutritional stress (p < 0.05 for all paired compare- sons). The combination of nutrients starvation and LM treatment resulted in a synergistic diminution of the vi- ability relative to the results observed in the presence of any tested metabolite. 3.2.3. Thermal Stress The viability of epimastigotes exposed to the simultane- ous treatment of LM and thermal stress was also evalu- ated. For thermal stress, three temperatures were chosen: 28˚C, the optimal temperature of growth for the insect vector stage; 37˚C, the temperature of the mammalian host; and 33˚C, the optimal temperature for the progres- sion of in vitro infection of mammalian cells [19]. The non-treated cultures behaved as previously observed, with the maximum growth rate and maximum station- ary-phase cell densities achieved at 33˚C rather than 28˚C (Figure 3(c)). As previously observed, both the growth rate and stationary-phase cell density were the lowest at 37˚C; however, these values were in the range of the control (28˚C). If LM interacts with mechanisms related to heat-shock resistance, then a significant change in the IC50 values at different temperatures would be ex- pected. However, the obtained results did not show sig- nificant interactions between LM and thermal stress. 3.2.4. pH Stress To evaluate the possible interaction of LM treatment with different pH conditions, the parasites were initially incubated in LIT with the pH adjusted to different values, and the cell growth at day 5 was determined. Parasites maintained at pH = 7.5 grew at rates comparable to those of the controls (control curve Figure 1), while the most extreme acidic pH evaluated significantly decreased the cell density achieved in these conditions. Surprisingly, parasites grown in slightly acidic conditions (pH = 6.5) showed the maximum growth inhibition (Figure 3(d)). Interestingly, when cultures grown in these conditions were subjected to the IC25 of LM (0.25 mM), no signifi- cant interaction was found between LM and acidic pH. In fact, an additive effect is clearly observed for each condi- tion, showing that this compound does not interfere with the resistance to this variable. 3.3. The Effect of LM on Differentiation to Infective Metacyclic Trypomastigotes One relevant process involving environmental sensing in terms of nutrient availability is the differentiation from the non-infective epimastigote to the infective trypomas- tigotes (a process called metacyclogenesis). Metacyc- logenesis begins after the cells are exposed to a nutria- tional stress, which naturally occurs in the terminal por- tion of the digestive tube of the invertebrate host. Be- cause LM treatment interacts synergistically with the effect of nutritional stress, we concluded that LM could also be interfering with metacyclogenesis. Epimastigotes were incubated in TAU-3AG differentiation medium Copyright © 2012 SciRes. IJCM
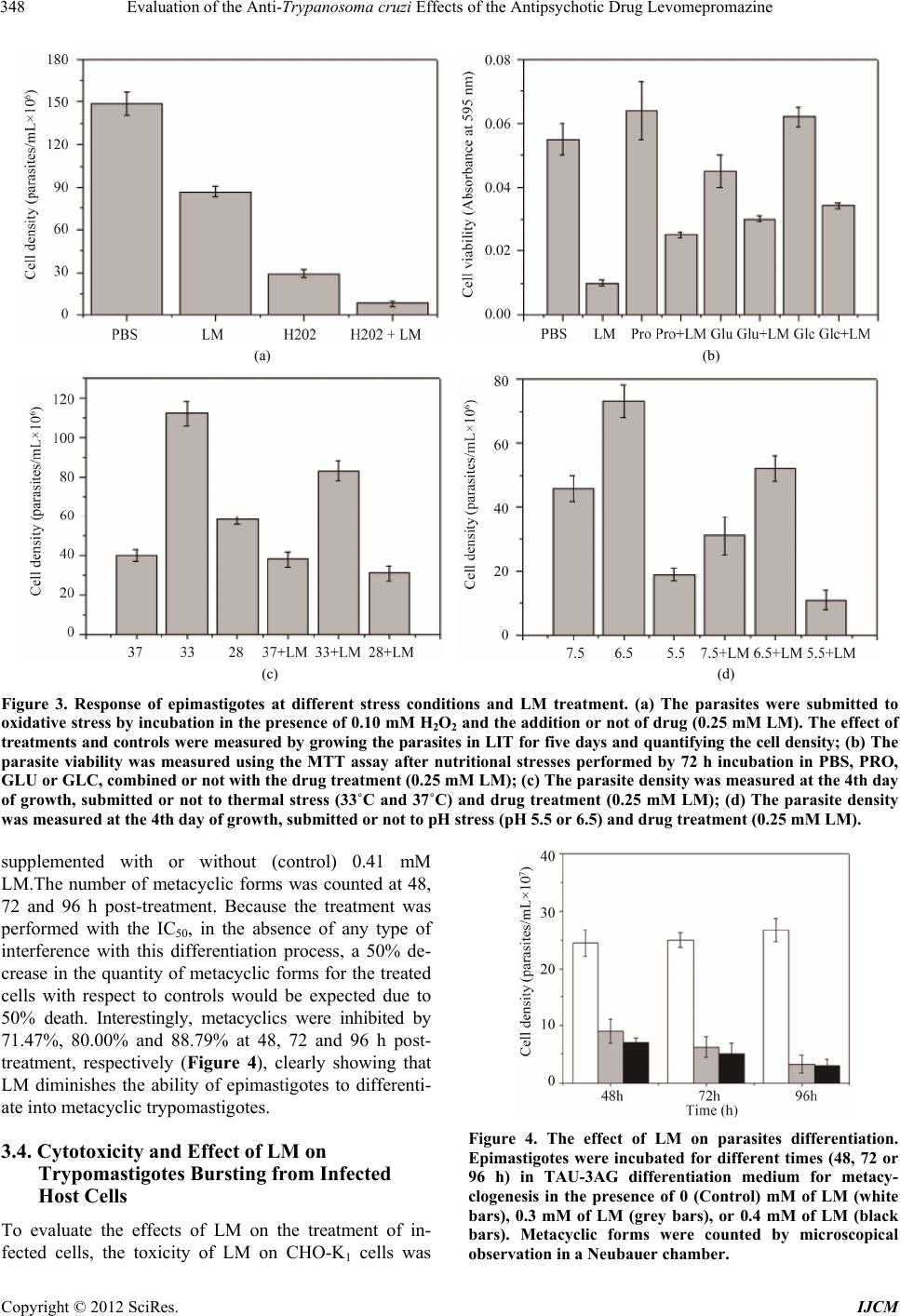 Evaluation of the Anti-Trypanosoma cruzi Effects of the Antipsychotic Drug Levomepromazine 348 (a) (b) (c) (d) Figure 3. Response of epimastigotes at different stress conditions and LM treatment. (a) The parasites were submitted to oxidative stress by incubation in the presence of 0.10 mM H2O2 and the addition or not of drug (0.25 mM LM). The effect of treatments and controls were measured by growing the parasites in LIT for five days and quantifying the cell density; (b) The parasite viability was measured using the MTT assay after nutritional stresses performed by 72 h incubation in PBS, PRO, GLU or GLC, combined or not with the drug treatment (0.25 mM LM); (c) The parasite density was measured at the 4th day of growth, submitted or not to thermal stress (33˚C and 37˚C) and drug treatment (0.25 mM LM); (d) The parasite density was measured at the 4th day of growth, submitted or not to pH stress (pH 5.5 or 6.5) and drug treatment (0.25 mM LM). supplemented with or without (control) 0.41 mM LM.The number of metacyclic forms was counted at 48, 72 and 96 h post-treatment. Because the treatment was performed with the IC50, in the absence of any type of interference with this differentiation process, a 50% de- crease in the quantity of metacyclic forms for the treated cells with respect to controls would be expected due to 50% death. Interestingly, metacyclics were inhibited by 71.47%, 80.00% and 88.79% at 48, 72 and 96 h post- treatment, respectively (Figure 4), clearly showing that LM diminishes the ability of epimastigotes to differenti- ate into metacyclic trypomastigotes. 3.4. Cytotoxicity and Effect of LM on Trypomastigotes Bursting from Infected Host Cells To evaluate the effects of LM on the treatment of in- fected cells, the toxicity of LM on CHO-K1 cells was Figure 4. The effect of LM on parasites differentiation. Epimastigotes were incubated for different times (48, 72 or 96 h) in TAU-3AG differentiation medium for metacy- clogenesis in the presence of 0 (Control) mM of LM (white bars), 0.3 mM of LM (grey bars), or 0.4 mM of LM (black bars). Metacyclic forms were counted by microscopical observation in a Neubauer chamber. Copyright © 2012 SciRes. IJCM
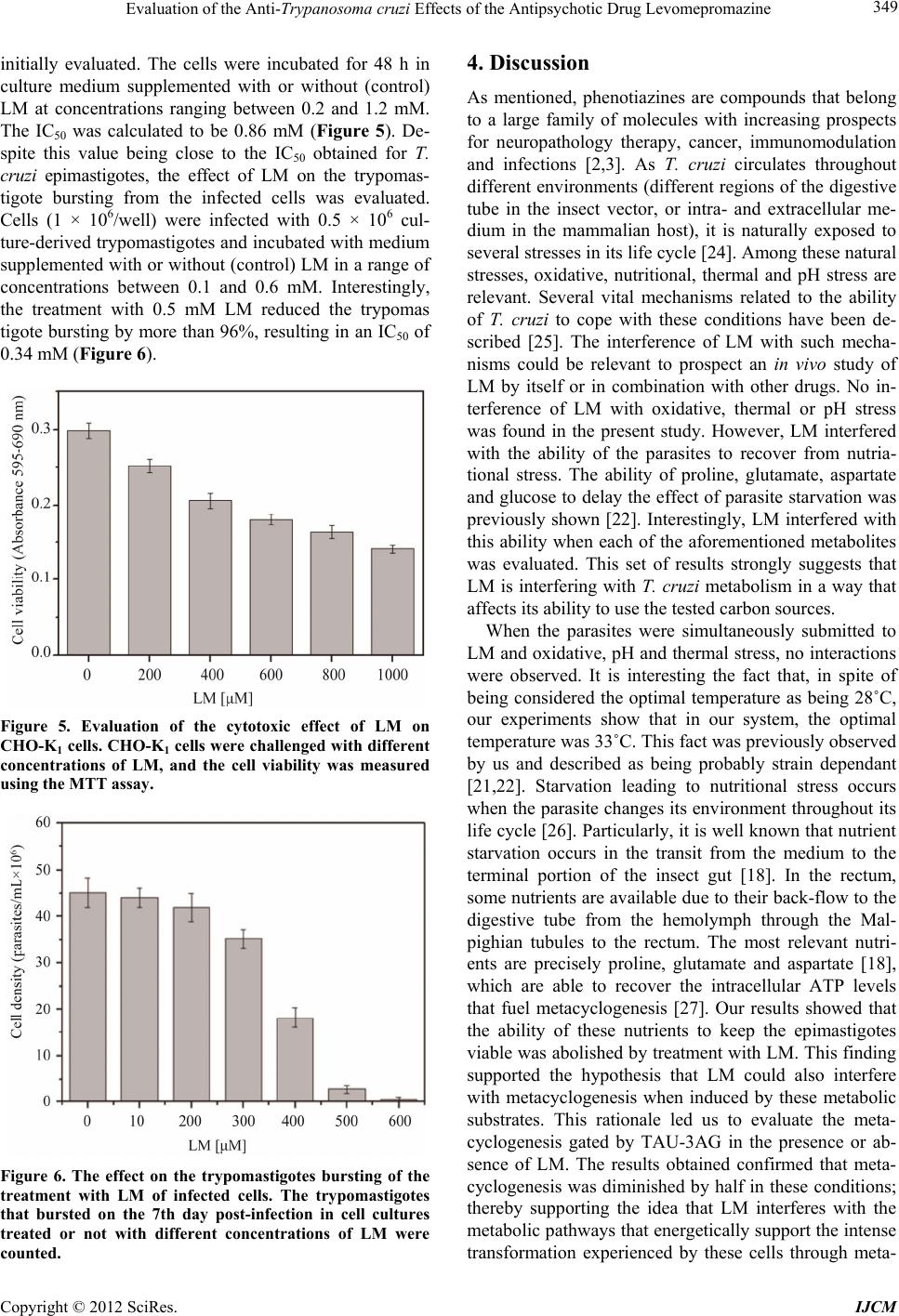 Evaluation of the Anti-Trypanosoma cruzi Effects of the Antipsychotic Drug Levomepromazine 349 initially evaluated. The cells were incubated for 48 h in culture medium supplemented with or without (control) LM at concentrations ranging between 0.2 and 1.2 mM. The IC50 was calculated to be 0.86 mM (Figure 5). De- spite this value being close to the IC50 obtained for T. cruzi epimastigotes, the effect of LM on the trypomas- tigote bursting from the infected cells was evaluated. Cells (1 × 106/well) were infected with 0.5 × 106 cul- ture-derived trypomastigotes and incubated with medium supplemented with or without (control) LM in a range of concentrations between 0.1 and 0.6 mM. Interestingly, the treatment with 0.5 mM LM reduced the trypomas tigote bursting by more than 96%, resulting in an IC50 of 0.34 mM (Figure 6). Figure 5. Evaluation of the cytotoxic effect of LM on CHO-K1 cells. CHO-K1 cells were challenged with different concentrations of LM, and the cell viability was measured using the MTT assay. Figure 6. The effect on the trypomastigotes bursting of the treatment with LM of infected cells. The trypomastigotes that bursted on the 7th day post-infection in cell cultures treated or not with different concentrations of LM were counted. 4. Discussion As mentioned, phenotiazines are compounds that belong to a large family of molecules with increasing prospects for neuropathology therapy, cancer, immunomodulation and infections [2,3]. As T. cruzi circulates throughout different environments (different regions of the digestive tube in the insect vector, or intra- and extracellular me- dium in the mammalian host), it is naturally exposed to several stresses in its life cycle [24]. Among these natural stresses, oxidative, nutritional, thermal and pH stress are relevant. Several vital mechanisms related to the ability of T. cruzi to cope with these conditions have been de- scribed [25]. The interference of LM with such mecha- nisms could be relevant to prospect an in vivo study of LM by itself or in combination with other drugs. No in- terference of LM with oxidative, thermal or pH stress was found in the present study. However, LM interfered with the ability of the parasites to recover from nutria- tional stress. The ability of proline, glutamate, aspartate and glucose to delay the effect of parasite starvation was previously shown [22]. Interestingly, LM interfered with this ability when each of the aforementioned metabolites was evaluated. This set of results strongly suggests that LM is interfering with T. cruzi metabolism in a way that affects its ability to use the tested carbon sources. When the parasites were simultaneously submitted to LM and oxidative, pH and thermal stress, no interactions were observed. It is interesting the fact that, in spite of being considered the optimal temperature as being 28˚C, our experiments show that in our system, the optimal temperature was 33˚C. This fact was previously observed by us and described as being probably strain dependant [21,22]. Starvation leading to nutritional stress occurs when the parasite changes its environment throughout its life cycle [26]. Particularly, it is well known that nutrient starvation occurs in the transit from the medium to the terminal portion of the insect gut [18]. In the rectum, some nutrients are available due to their back-flow to the digestive tube from the hemolymph through the Mal- pighian tubules to the rectum. The most relevant nutri- ents are precisely proline, glutamate and aspartate [18], which are able to recover the intracellular ATP levels that fuel metacyclogenesis [27]. Our results showed that the ability of these nutrients to keep the epimastigotes viable was abolished by treatment with LM. This finding supported the hypothesis that LM could also interfere with metacyclogenesis when induced by these metabolic substrates. This rationale led us to evaluate the meta- cyclogenesis gated by TAU-3AG in the presence or ab- sence of LM. The results obtained confirmed that meta- cyclogenesis was diminished by half in these conditions; thereby supporting the idea that LM interferes with the metabolic pathways that energetically support the intense transformation experienced by these cells through meta- Copyright © 2012 SciRes. IJCM
 Evaluation of the Anti-Trypanosoma cruzi Effects of the Antipsychotic Drug Levomepromazine 350 cyclogenesis. Finally, the effect of LM on trypomastigote burst was also evaluated. Our results showed that the treatment of infected cells with 0.5 mM LM resulted in more than 90% inhibition of trypomastigote bursting. The treatment of T. cruzi with another phenothiazine derived drug, thi- oridazine (usually prescribed as a neuroleptic drug), at low doses seemed to be promising for epimastigotes (IC50 = 5 μM). However, trypomastigotes were less sen- sitive to the drug, showing an IC50 of 0.5 mM [28]. In addition, the treatment of mice with thioridazine partially prevented the development of parasitemia; however, some cardiac damage was not prevented, even in the ab- sence of the evidence of amastigote nests [29]. Collectively, our data support the idea that the meta- bolic steps involved in the oxidation of glucose, proline, aspartate and glutamate are involved in the antiparasitic activity of LM. Further experiments will be conducted to define the molecular targets involved in this antiparasitic activity, which will, in turn, lead to the optimisation of more efficient LM-derived drugs. 5. Acknowledgements The authors are deeply acknowledged to Dr. Laura Tanga for helpful discussions and interesting suggestions. Fi- nancial Support: this work was supported by grants from the Fundação de Amparo à Pesquisa do Estado de São Paulo (FAPESP grant #11/50631-1 to AMS), Instituto Nacional de Biologia Estrutural e Química Medicinal em Doenças Infecciosas (INBEQMeDI), and Conselho Na- cional de Desenvolvimento Científico e Tecnológico (CNPq grant # 470272/2011-2 to AMS). REFERENCES [1] S. B. Boscardin, A. C. Torrecilhas, R. Manarin, S. Revelli, E. G. Rey, R. R. Tonelli and A. M. Silber, “Chagas’ Dis- ease: An Update on Immune Mechanisms and Thera- peutic Strategies,” Journal of Cellular and Molecular Medicine, Vol. 14, No. 6, 2010, pp. 1373-1384. doi:10.1111/j.1582-4934.2010.01007.x [2] G. Sudeshna and K. Parimal, “Multiple Non-Psychiatric Effects of Phenothiazines: A Review,” European Journal of Pharmacology, Vol. 648, No. 1, 2010, pp. 6-14. doi:10.1016/j.ejphar.2010.08.045 [3] M. J. Ohlow and B. Moosmann, “Phenothiazine: The Seven Lives of Pharmacology’s First Lead Structure,” Drug Discovery Today, Vol. 16, No. 3, 2011, pp. 119- 131. doi:10.1016/j.drudis.2011.01.001 [4] A. P. Feinberg and S. H. Snyder, “Phenothiazine Drugs: Structure-Activity Relationships Explained by a Confor- mation That Mimics Dopamine,” Proceedings of the Na- tional Academy of Science of the USA, Vol. 72, No. 5, 1975, pp. 1899-1903. doi:10.1073/pnas.72.5.1899 [5] S. Sammut, K. E. Bray and A. R. West, “Dopamine D2 Receptor-Dependent Modulation of Striatal NO Synthase Activity,” Psychopharmacology, Vol. 191, No. 3, 2007, pp. 793-803. doi:10.1007/s00213-006-0681-z [6] T. Silverstone, “Clinically Relevant Differences between Antipsychotic Compounds,” Acta Psychiatrica Scandi- navica, Supplement, Vol. 358, 1990, pp. 88-91. doi:10.1111/j.1600-0447.1990.tb05296.x [7] S. H. Snyder, S. P. Banerjee, H. I. Yamamura and D. Greenberg, “Drugs, Neurotransmitters, and Schizophre- nia,” Science, Vol. 184, No. 4143, 1974, pp. 1243-1253. doi:10.1126/science.184.4143.1243 [8] F. L. Schuster and N. Mandel, “Phenothiazine Compounds Inhibit in Vitro Growth of Pathogenic Free-Living Amo- ebae,” Antimicrobials Agents and Chemotherapy, Vol. 25, No. 1, 1984, pp. 109-112. doi:10.1128/AAC.25.1.109 [9] A. Makioka, M. Kumagai, S. Kobayashi and T. Takeuchi, “Possible Role of Calcium Ions, Calcium Channels and Calmodulin in Excystation and Metacystic Development of Entamoeba invadens,” Parasitology Research, Vol. 88, No. 9, 2002, pp. 837-843. doi:10.1007/s00436-002-0676-6 [10] R. D. Pearson, A. A. Manian, J. L. Harcus, D. Hall and E. L. Hewlett, “Lethal Effect of Phenothiazine Neuroleptics on the Pathogenic Protozoan Leishmania donovani,” Sci- ence, Vol. 217, No. 4557, 1982, pp. 369-371. doi:10.1126/science.6124040 [11] N. C. Wood and K. M. Nugent, “Inhibitory Effects of Chlorpromazine on Candida Species,” Antimicrobial Ag- ents and Chemotherapy, Vol. 27, No. 5, 1985, pp. 692- 694. doi:10.1128/AAC.27.5.692 [12] D. Ordway, M. Viveiros, C. Leandro, M. Jorge Arroz, J. Molnar, J. E. Kristiansen and L. Amaral, “Chlorpromazine Has Intracellular Killing Activity against Phagocytosed Staphylococcus aureus at Clinical Concentrations,” Jour- nal of Infection and Chemotherapy, Vol. 8, No. 3, 2002, pp. 227-231. doi:10.1007/s10156-002-0188-4 [13] A. J. Crowle, E. R. Ross, D. L. Cohn, J. Gilden and M. H. May, “Comparison of the Abilities of Mycobacterium avium and Mycobacterium intracellulare to Infect and Multiply in Cultured Human Macrophages from Normal and Human Immunodeficiency Virus-Infected Subjects,” Infection and Immunity, Vol. 60, 1992, pp. 3697-3703. [14] P. Ratnakar and P. S. Murthy, “Antitubercular Activity of Trifluoperazine, a Calmodulin Antagonist,” FEMS Mi- crobiology Letters, Vol. 76, 1992, pp. 73-76. doi:10.1111/j.1574-6968.1992.tb05442.x [15] H. Most, “Studies on the Effectiveness of Phenothiazine in Human Nematode Infections,” American Journal of Tropical Medicine and Hygiene, Vol. s1, 1943, pp. 459- 464. [16] Z. Brener and E. Chiari, “Morphological Variations Ob- served in Different Strains of Trypanosoma cruzi,” Re- vista do Instituto de Medicina Tropical de Sao Paulo, Vol. 19, 1963, pp. 220-224. [17] E. P. Camargo, “Growth and Differentiation in Trypano- soma cruzi. I. Origin of Metacyclic Trypanosomes in Liquid Media,” Revista do Instituto de Medicina Tropical de Sao Paulo, Vol. 12, 1964, pp. 93-100. Copyright © 2012 SciRes. IJCM
 Evaluation of the Anti-Trypanosoma cruzi Effects of the Antipsychotic Drug Levomepromazine Copyright © 2012 SciRes. IJCM 351 [18] V. T. Contreras, J. M. Salles, N. Thomas, C. M. Morel and S. Goldenberg, “In Vitro Differentiation of Trypa- nosoma cruzi under Chemically Defined Conditions,” Molecular and Biochemical Parasitology, Vol. 16, 1985, pp. 315-327. doi:10.1016/0166-6851(85)90073-8 [19] R. R. Tonelli, A. M. Silber, M. Almeida-de-Faria, I. Y. Hirata, W. Colli and M. J. Alves, “L-Proline Is Essential for the Intracellular Differentiation of Trypanosoma cruzi,” Cellular Microbiology, Vol. 6, 2004, pp. 733-741. doi:10.1111/j.1462-5822.2004.00397.x [20] I. Stolic, K. Miskovic, A. Magdaleno, A. M. Silber, I. Piantanida, M. Bajic and L. Glavas-Obrovac, “Effect of 3,4-Ethylenedioxy-Extension of Thiophene Core on the DNA/RNA Binding Properties and Biological Activity of Bisbenzimidazole Amidines,” Bioorganic and Medicinal Chemistry, Vol. 17, No. 6, 2009, pp. 2544-2554. doi:10.1016/j.bmc.2009.01.071 [21] A. Magdaleno, I. Y. Ahn, L. S. Paes and A. M. Silber, “Actions of a Proline Analogue, L-Thiazolidine-4-Car- boxylic Acid (T4C), on Trypanosoma cruzi,” PLoS ONE, Vol. 4, No. 2, 2009, Article ID: e4534. doi:10.1371/journal.pone.0004534 [22] A. Magdaleno, B. Suarez Mantilla, S. C. Rocha, E. M. Pral and A. M. Silber, “The Involvement of Glutamate Metabolism in the Resistance to Thermal, Nutritional, and Oxidative Stress in Trypanosoma cruzi,” Enzyme Res- earch, Vol. 2011, 2011, Article ID: 486928. doi:10.4061/2011/486928 [23] B. K. Slinker, “The Statistics of Synergism,” Journal of Molecular and Cellular Cardiology, Vol. 30, No. 4, 1998, pp. 723-731. doi:10.1006/jmcc.1998.0655 [24] A. M. Silber, W. Colli, H. Ulrich, M. J. Alves and C. A. Pereira, “Amino Acid Metabolic Routes in Trypanosoma cruzi: Possible Therapeutic Targets against Chagas’ Dis- ease,” Current Drug Targets: Infectious Disorders, Vol. 5, 2005, pp. 53-64. doi:10.2174/1568005053174636 [25] P. Lisvane Silva, B. S. Mantilla, M. J. Barison, C. Wrenger and A. M. Silber, “The Uniqueness of the Try- panosoma cruzi Mitochondrion: Opportunities to Identify New Drug Target for the Treatment of Chagas Disease,” Current Pharmaceutical Design, Vol. 17, 2011, pp. 2074- 2099. doi:10.2174/138161211796904786 [26] J. J. Cazzulo, “Energy Metabolism in Trypanosoma cruz i,” Subcellular Biochemistry, Vol. 18, 1992, pp. 235- 257. [27] P. Paglini-Oliva, A. R. Fernandez, R. Fretes and A. Pesl- man, “Structural, Ultrastructural Studies and Evolution of Trypanosoma cruzi-Infected Mice Treated with Thiori- dazine,” Experimental and Molecular Pathology, Vol. 65, 1998, pp. 78-86. doi:10.1006/exmp.1998.2227 [28] M. S. Lo Presti, H. W. Rivarola, J. M. Bustamante, A. R. Fernandez, J. E. Enders, R. Fretes, S. Gea and P. A. Pag- lini-Oliva, “Thioridazine Treatment Prevents Cardiopathy in Trypanosoma cruzi Infected Mice,” International Jour- nal of Antimicrobial Agents, Vol. 23, 2004, pp. 634-636. doi:10.1016/j.ijantimicag.2003.10.006 [29] H. W. Rivarola, A. R. Fernandez, J. E. Enders, R. Fretes, S. Gea, M. Suligoy, J. A. Palma and P. Paglini-Oliva, “Thioridazine Treatment Modifies the Evolution of Try- panosoma cruzi Infection in Mice,” Annals of Tropical Medicine and Parasitology, Vol. 93, No. 7, 1999, pp. 695-702. doi:10.1080/00034989957943
|