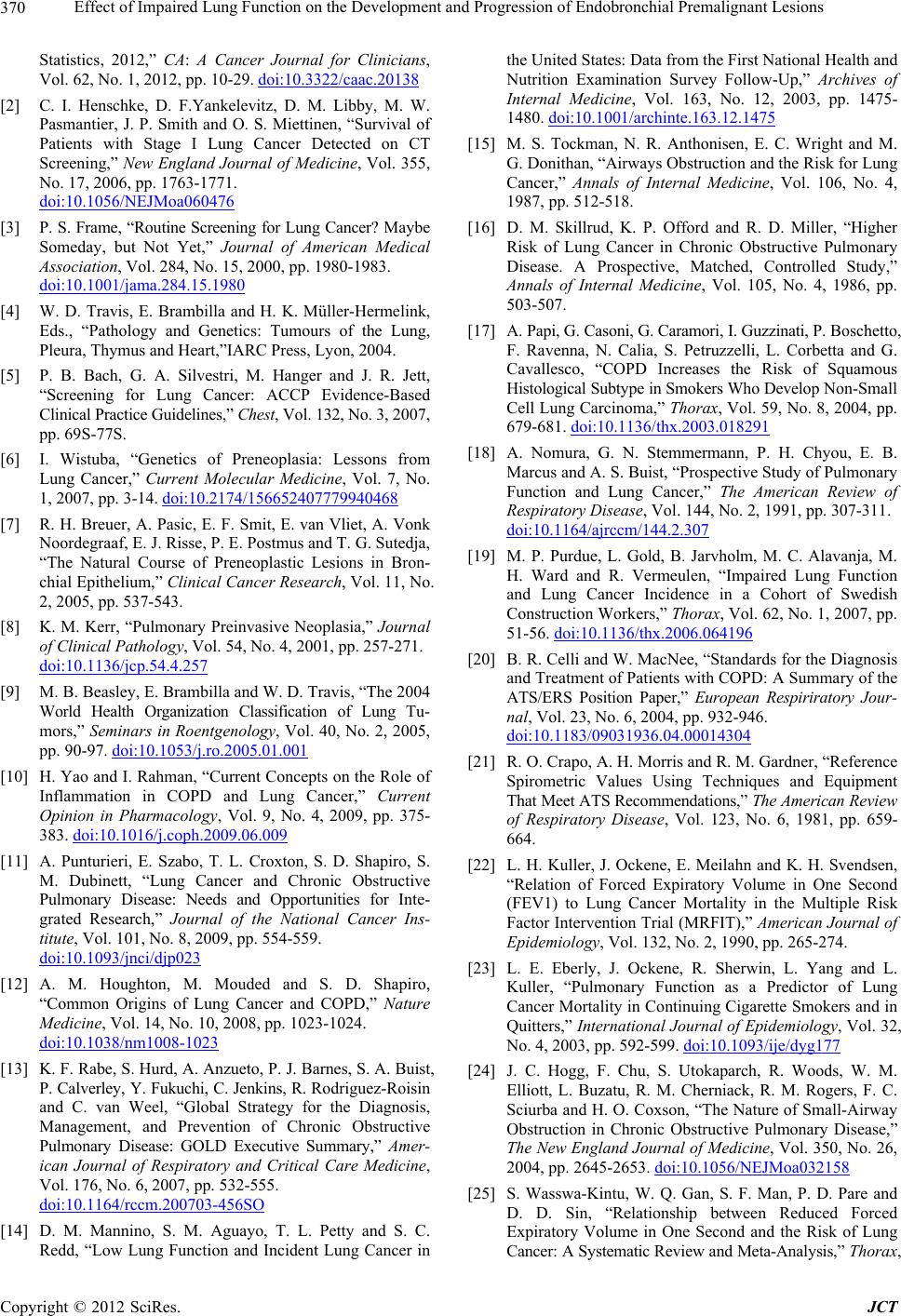
Effect of Impaired Lung Function on the Development and Progression of Endobronchial Premalignant Lesions
370
Statistics, 2012,” CA: A Cancer Journal for Clinicians,
Vol. 62, No. 1, 2012, pp. 10-29. doi:10.3322/caac.20138
[2] C. I. Henschke, D. F.Yankelevitz, D. M. Libby, M. W.
Pasmantier, J. P. Smith and O. S. Miettinen, “Survival of
Patients with Stage I Lung Cancer Detected on CT
Screening,” New England Journal of Medicine, Vol. 355,
No. 17, 2006, pp. 1763-1771.
doi:10.1056/NEJMoa060476
[3] P. S. Frame, “Routine Screening for Lung Cancer? Maybe
Someday, but Not Yet,” Journal of American Medical
Association, Vol. 284, No. 15, 2000, pp. 1980-1983.
doi:10.1001/jama.284.15.1980
[4] W. D. Travis, E. Brambilla and H. K. Müller-Hermelink,
Eds., “Pathology and Genetics: Tumours of the Lung,
Pleura, Thymus and Heart,”IARC Press, Lyon, 2004.
[5] P. B. Bach, G. A. Silvestri, M. Hanger and J. R. Jett,
“Screening for Lung Cancer: ACCP Evidence-Based
Clinical Practice Guidelines,” Chest, Vol. 132, No. 3, 2007,
pp. 69S-77S.
[6] I. Wistuba, “Genetics of Preneoplasia: Lessons from
Lung Cancer,” Current Molecular Medicine, Vol. 7, No.
1, 2007, pp. 3-14. doi:10.2174/156652407779940468
[7] R. H. Breuer, A. Pasic, E. F. Smit, E. van Vliet, A. Vonk
Noordegraaf, E. J. Risse, P. E. Postmus and T. G. Sutedja,
“The Natural Course of Preneoplastic Lesions in Bron-
chial Epithelium,” Clinical Cancer Research, Vol. 11, No.
2, 2005, pp. 537-543.
[8] K. M. Kerr, “Pulmonary Preinvasive Neoplasia,” Journal
of Clinical Pathology, Vol. 54, No. 4, 2001, pp. 257-271.
doi:10.1136/jcp.54.4.257
[9] M. B. Beasley, E. Brambilla and W. D. Travis, “The 2004
World Health Organization Classification of Lung Tu-
mors,” Seminars in Roentgenology, Vol. 40, No. 2, 2005,
pp. 90-97. doi:10.1053/j.ro.2005.01.001
[10] H. Yao and I. Rahman, “Current Concepts on the Role of
Inflammation in COPD and Lung Cancer,” Current
Opinion in Pharmacology, Vol. 9, No. 4, 2009, pp. 375-
383. doi:10.1016/j.coph.2009.06.009
[11] A. Punturieri, E. Szabo, T. L. Croxton, S. D. Shapiro, S.
M. Dubinett, “Lung Cancer and Chronic Obstructive
Pulmonary Disease: Needs and Opportunities for Inte-
grated Research,” Journal of the National Cancer Ins-
titute, Vol. 101, No. 8, 2009, pp. 554-559.
doi:10.1093/jnci/djp023
[12] A. M. Houghton, M. Mouded and S. D. Shapiro,
“Common Origins of Lung Cancer and COPD,” Nature
Medicine, Vol. 14, No. 10, 2008, pp. 1023-1024.
doi:10.1038/nm1008-1023
[13] K. F. Rabe, S. Hurd, A. Anzueto, P. J. Barnes, S. A. Buist,
P. Calverley, Y. Fukuchi, C. Jenkins, R. Rodriguez-Roisin
and C. van Weel, “Global Strategy for the Diagnosis,
Management, and Prevention of Chronic Obstructive
Pulmonary Disease: GOLD Executive Summary,” Amer-
ican Journal of Respiratory and Critical Care Medicine,
Vol. 176, No. 6, 2007, pp. 532-555.
doi:10.1164/rccm.200703-456SO
[14] D. M. Mannino, S. M. Aguayo, T. L. Petty and S. C.
Redd, “Low Lung Function and Incident Lung Cancer in
the United States: Data from the First National Health and
Nutrition Examination Survey Follow-Up,” Archives of
Internal Medicine, Vol. 163, No. 12, 2003, pp. 1475-
1480. doi:10.1001/archinte.163.12.1475
[15] M. S. Tockman, N. R. Anthonisen, E. C. Wright and M.
G. Donithan, “Airways Obstruction and the Risk for Lung
Cancer,” Annals of Internal Medicine, Vol. 106, No. 4,
1987, pp. 512-518.
[16] D. M. Skillrud, K. P. Offord and R. D. Miller, “Higher
Risk of Lung Cancer in Chronic Obstructive Pulmonary
Disease. A Prospective, Matched, Controlled Study,”
Annals of Internal Medicine, Vol. 105, No. 4, 1986, pp.
503-507.
[17] A. Papi, G. Casoni, G. Caramori, I. Guzzinati, P. Boschetto,
F. Ravenna, N. Calia, S. Petruzzelli, L. Corbetta and G.
Cavallesco, “COPD Increases the Risk of Squamous
Histological Subtype in Smokers Who Develop Non-Small
Cell Lung Carcinoma,” Thorax, Vol. 59, No. 8, 2004, pp.
679-681. doi:10.1136/thx.2003.018291
[18] A. Nomura, G. N. Stemmermann, P. H. Chyou, E. B.
Marcus and A. S. Buist, “Prospective Study of Pulmonary
Function and Lung Cancer,” The American Review of
Respiratory Disease, Vol. 144, No. 2, 1991, pp. 307-311.
doi:10.1164/ajrccm/144.2.307
[19] M. P. Purdue, L. Gold, B. Jarvholm, M. C. Alavanja, M.
H. Ward and R. Vermeulen, “Impaired Lung Function
and Lung Cancer Incidence in a Cohort of Swedish
Construction Workers,” Thorax, Vol. 62, No. 1, 2007, pp.
51-56. doi:10.1136/thx.2006.064196
[20] B. R. Celli and W. MacNee, “Standards for the Diagnosis
and Treatment of Patients with COPD: A Summary of the
ATS/ERS Position Paper,” European Respiriratory Jour-
nal, Vol. 23, No. 6, 2004, pp. 932-946.
doi:10.1183/09031936.04.00014304
[21] R. O. Crapo, A. H. Morris and R. M. Gardner, “Reference
Spirometric Values Using Techniques and Equipment
That Meet ATS Recommendations,” The American Review
of Respiratory Disease, Vol. 123, No. 6, 1981, pp. 659-
664.
[22] L. H. Kuller, J. Ockene, E. Meilahn and K. H. Svendsen,
“Relation of Forced Expiratory Volume in One Second
(FEV1) to Lung Cancer Mortality in the Multiple Risk
Factor Intervention Trial (MRFIT),” American Journal of
Epidemiology, Vol. 132, No. 2, 1990, pp. 265-274.
[23] L. E. Eberly, J. Ockene, R. Sherwin, L. Yang and L.
Kuller, “Pulmonary Function as a Predictor of Lung
Cancer Mortality in Continuing Cigarette Smokers and in
Quitters,” International Journal of Epidemiology, Vol. 32,
No. 4, 2003, pp. 592-599. doi:10.1093/ije/dyg177
[24] J. C. Hogg, F. Chu, S. Utokaparch, R. Woods, W. M.
Elliott, L. Buzatu, R. M. Cherniack, R. M. Rogers, F. C.
Sciurba and H. O. Coxson, “The Nature of Small-Airway
Obstruction in Chronic Obstructive Pulmonary Disease,”
The New England Journal of Medicine, Vol. 350, No. 26,
2004, pp. 2645-2653. doi:10.1056/NEJMoa032158
[25] S. Wasswa-Kintu, W. Q. Gan, S. F. Man, P. D. Pare and
D. D. Sin, “Relationship between Reduced Forced
Expiratory Volume in One Second and the Risk of Lung
Cancer: A Systematic Review and Meta-Analysis,” Thorax,
Copyright © 2012 SciRes. JCT