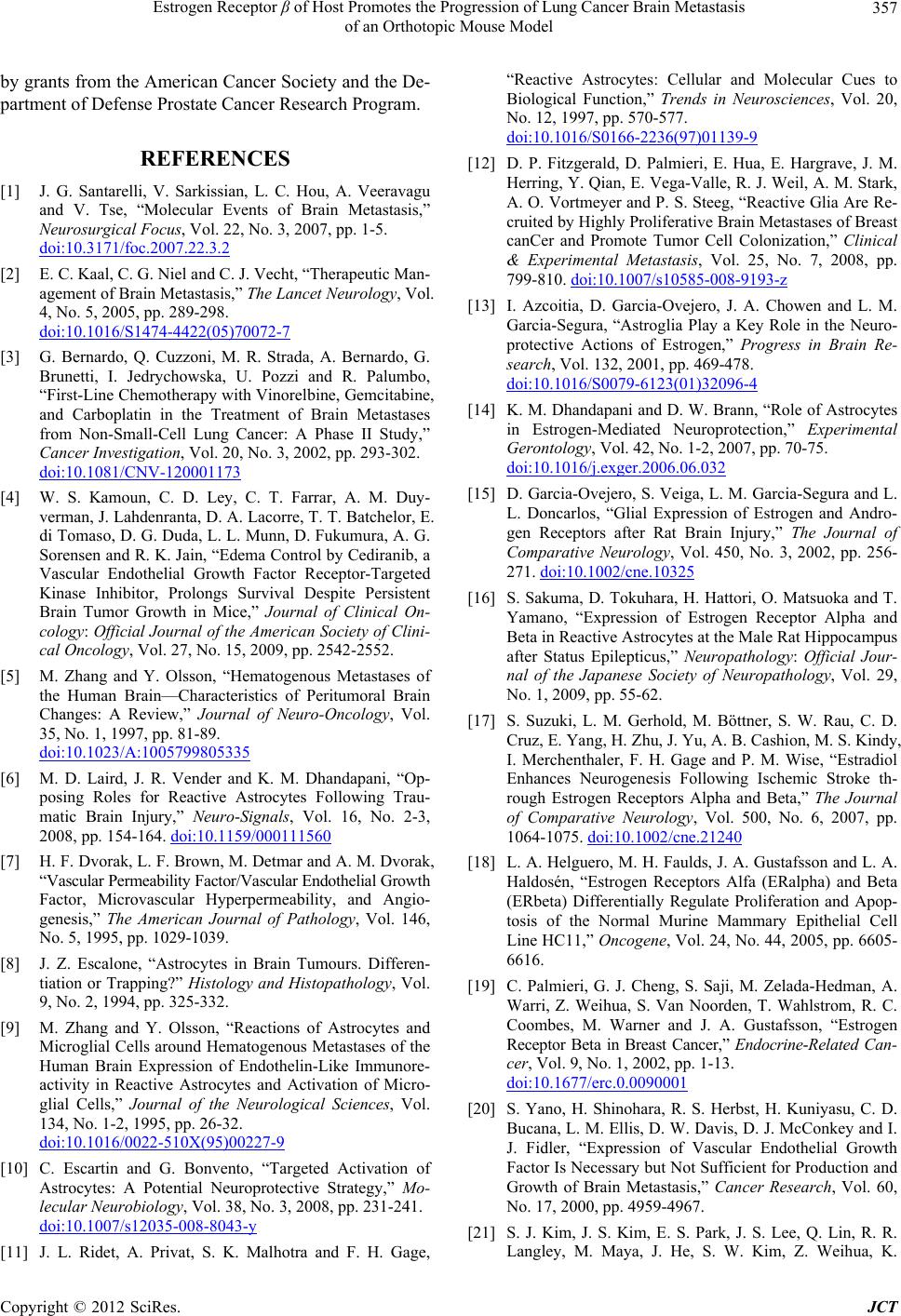
Estrogen Receptor β of Host Promotes the Progression of Lung Cancer Brain Metastasis
of an Orthotopic Mouse Model
357
by grants from the American Cancer Society and the De-
partment of Defense Prostate Cancer Research Program.
REFERENCES
[1] J. G. Santarelli, V. Sarkissian, L. C. Hou, A. Veeravagu
and V. Tse, “Molecular Events of Brain Metastasis,”
Neurosurgical Focus, Vol. 22, No. 3, 2007, pp. 1-5.
doi:10.3171/foc.2007.22.3.2
[2] E. C. Kaal, C. G. Niel and C. J. Vecht, “Therapeutic Man-
agement of Brain Metastasis,” The Lancet Neurology, Vol.
4, No. 5, 2005, pp. 289-298.
doi:10.1016/S1474-4422(05)70072-7
[3] G. Bernardo, Q. Cuzzoni, M. R. Strada, A. Bernardo, G.
Brunetti, I. Jedrychowska, U. Pozzi and R. Palumbo,
“First-Line Chemotherapy with Vinorelbine, Gemcitabine,
and Carboplatin in the Treatment of Brain Metastases
from Non-Small-Cell Lung Cancer: A Phase II Study,”
Cancer Investigation, Vol. 20, No. 3, 2002, pp. 293-302.
doi:10.1081/CNV-120001173
[4] W. S. Kamoun, C. D. Ley, C. T. Farrar, A. M. Duy-
verman, J. Lahdenranta, D. A. Lacorre, T. T. Batchelor, E.
di Tomaso, D. G. Duda, L. L. Munn, D. Fukumura, A. G.
Sorensen and R. K. Jain, “Edema Control by Cediranib, a
Vascular Endothelial Growth Factor Receptor-Targeted
Kinase Inhibitor, Prolongs Survival Despite Persistent
Brain Tumor Growth in Mice,” Journal of Clinical On-
cology: Official Journal of the American Society of Clini-
cal Oncology, Vol. 27, No. 15, 2009, pp. 2542-2552.
[5] M. Zhang and Y. Olsson, “Hematogenous Metastases of
the Human Brain—Characteristics of Peritumoral Brain
Changes: A Review,” Journal of Neuro-Oncology, Vol.
35, No. 1, 1997, pp. 81-89.
doi:10.1023/A:1005799805335
[6] M. D. Laird, J. R. Vender and K. M. Dhandapani, “Op-
posing Roles for Reactive Astrocytes Following Trau-
matic Brain Injury,” Neuro-Signals, Vol. 16, No. 2-3,
2008, pp. 154-164. doi:10.1159/000111560
[7] H. F. Dvorak, L. F. Brown, M. Detmar and A. M. Dvorak,
“Vascular Permeability Factor/Vascular Endothelial Growth
Factor, Microvascular Hyperpermeability, and Angio-
genesis,” The American Journal of Pathology, Vol. 146,
No. 5, 1995, pp. 1029-1039.
[8] J. Z. Escalone, “Astrocytes in Brain Tumours. Differen-
tiation or Trapping?” Histology and Histopathology, Vol.
9, No. 2, 1994, pp. 325-332.
[9] M. Zhang and Y. Olsson, “Reactions of Astrocytes and
Microglial Cells around Hematogenous Metastases of the
Human Brain Expression of Endothelin-Like Immunore-
activity in Reactive Astrocytes and Activation of Micro-
glial Cells,” Journal of the Neurological Sciences, Vol.
134, No. 1-2, 1995, pp. 26-32.
doi:10.1016/0022-510X(95)00227-9
[10] C. Escartin and G. Bonvento, “Targeted Activation of
Astrocytes: A Potential Neuroprotective Strategy,” Mo-
lecular Neurobiology, Vol. 38, No. 3, 2008, pp. 231-241.
doi:10.1007/s12035-008-8043-y
[11] J. L. Ridet, A. Privat, S. K. Malhotra and F. H. Gage,
“Reactive Astrocytes: Cellular and Molecular Cues to
Biological Function,” Trends in Neurosciences, Vol. 20,
No. 12, 1997, pp. 570-577.
doi:10.1016/S0166-2236(97)01139-9
[12] D. P. Fitzgerald, D. Palmieri, E. Hua, E. Hargrave, J. M.
Herring, Y. Qian, E. Vega-Valle, R. J. Weil, A. M. Stark,
A. O. Vortmeyer and P. S. Steeg, “Reactive Glia Are Re-
cruited by Highly Proliferative Brain Metastases of Breast
canCer and Promote Tumor Cell Colonization,” Clinical
& Experimental Metastasis, Vol. 25, No. 7, 2008, pp.
799-810. doi:10.1007/s10585-008-9193-z
[13] I. Azcoitia, D. Garcia-Ovejero, J. A. Chowen and L. M.
Garcia-Segura, “Astroglia Play a Key Role in the Neuro-
protective Actions of Estrogen,” Progress in Brain Re-
search, Vol. 132, 2001, pp. 469-478.
doi:10.1016/S0079-6123(01)32096-4
[14] K. M. Dhandapani and D. W. Brann, “Role of Astrocytes
in Estrogen-Mediated Neuroprotection,” Experimental
Gerontology, Vol. 42, No. 1-2, 2007, pp. 70-75.
doi:10.1016/j.exger.2006.06.032
[15] D. Garcia-Ovejero, S. Veiga, L. M. Garcia-Segura and L.
L. Doncarlos, “Glial Expression of Estrogen and Andro-
gen Receptors after Rat Brain Injury,” The Journal of
Comparative Neurology, Vol. 450, No. 3, 2002, pp. 256-
271. doi:10.1002/cne.10325
[16] S. Sakuma, D. Tokuhara, H. Hattori, O. Matsuoka and T.
Yamano, “Expression of Estrogen Receptor Alpha and
Beta in Reactive Astrocytes at the Male Rat Hippocampus
after Status Epilepticus,” Neuropathology: Official Jour-
nal of the Japanese Society of Neuropathology, Vol. 29,
No. 1, 2009, pp. 55-62.
[17] S. Suzuki, L. M. Gerhold, M. Böttner, S. W. Rau, C. D.
Cruz, E. Yang, H. Zhu, J. Yu, A. B. Cashion, M. S. Kindy,
I. Merchenthaler, F. H. Gage and P. M. Wise, “Estradiol
Enhances Neurogenesis Following Ischemic Stroke th-
rough Estrogen Receptors Alpha and Beta,” The Journal
of Comparative Neurology, Vol. 500, No. 6, 2007, pp.
1064-1075. doi:10.1002/cne.21240
[18] L. A. Helguero, M. H. Faulds, J. A. Gustafsson and L. A.
Haldosén, “Estrogen Receptors Alfa (ERalpha) and Beta
(ERbeta) Differentially Regulate Proliferation and Apop-
tosis of the Normal Murine Mammary Epithelial Cell
Line HC11,” Oncogene, Vol. 24, No. 44, 2005, pp. 6605-
6616.
[19] C. Palmieri, G. J. Cheng, S. Saji, M. Zelada-Hedman, A.
Warri, Z. Weihua, S. Van Noorden, T. Wahlstrom, R. C.
Coombes, M. Warner and J. A. Gustafsson, “Estrogen
Receptor Beta in Breast Cancer,” Endocrine-Related Can-
cer, Vol. 9, No. 1, 2002, pp. 1-13.
doi:10.1677/erc.0.0090001
[20] S. Yano, H. Shinohara, R. S. Herbst, H. Kuniyasu, C. D.
Bucana, L. M. Ellis, D. W. Davis, D. J. McConkey and I.
J. Fidler, “Expression of Vascular Endothelial Growth
Factor Is Necessary but Not Sufficient for Production and
Growth of Brain Metastasis,” Cancer Research, Vol. 60,
No. 17, 2000, pp. 4959-4967.
[21] S. J. Kim, J. S. Kim, E. S. Park, J. S. Lee, Q. Lin, R. R.
Langley, M. Maya, J. He, S. W. Kim, Z. Weihua, K.
Copyright © 2012 SciRes. JCT