 International Journal of Clean Coal and Energy, 2012, 1, 35-42 http://dx.doi.org/10.4236/ijcce.2012.13004 Published Online August 2012 (http://www.SciRP.org/journal/ijcce) Thermal Behavior of Coal and Biomass Blends in Inert and Oxidizing Gaseous Environments Ping Wang1*, Sheila W. Hedges1, Kent Casleton2, Chris Guenther2 1Department of Energy (DOE), National Energy Technology Laboratory (NETL), Pittsburgh, USA 2Department of Energy (DOE), National Energy Technology Laboratory (NETL), Morgantown, USA Email: *ping.wang@netl.doe.gov Received June 7, 2012; revised July 18, 2012; accepted August 10, 2012 ABSTRACT Oxy-fuel combustion and gasification (pre-combustion) may have potential for capturing carbon dioxide at lower costs for power generation. Oxy-co-firing and co-gasifying coal with biomass could further reduce effective CO2 emissions and utilize renewable energy resources. A key feature of these two approaches is that they process fuel in concentrated CO2 or O2/CO2 instead of N2 or O2/N2. Accurate predictive models of these processes using blends of coal and biomass can be used in process simulation and could aid in the development and implementation of these technologies. To de- velop these accurate predictive models, it is important to understand the conversion routes and thermal behavior of these fuels in appropriate gas environments. The objectives of this study are to investigate the impact of inert and oxida- tive gaseous environments on thermal behavior and reactivity of coal and biomass blends and to study the effect of bio- mass percentage on coal/biomass blend co-utilization. Fuel samples included a Powder River Basin (PRB) sub-bituminous coal, yellow pine wood sawdust pellets, and mixtures of 10 and 20 weight percent wood in coal. The samples were tested under N2, CO2, and 10% O2 in CO2 by volume using a non-isothermal thermogravimetric method for tempera- tures up to 1000˚C. Fuel weight losses of both coal and wood are essentially the same in CO2 as in N2 in the low tem- perature range, but higher in 10% O2 in CO2 compared to N2 and CO2. However, total weight losses at 1000˚C under CO2 and 10% O2 in CO2 are similar and higher than in N2 due to char gasification by the CO2 and combustion by O2. The char combustion in10% O2 in CO2 takes place at lower temperature than char gasification in CO2. Coal and wood blends have higher reactivity compared to coal alone in the lower temperature range due to the high volatile matter content of wood. Interactions of wood and coal in these gas environments and blend percentage are discussed. Keywords: Pyrolysis; Gasification; Combustion; Coal-Biomass Blends 1. Introduction Coal is the dominant energy resource for electricity gen- eration in the US because coal is abundant and less ex- pensive than other options. The combustion of coal to gen- erate electricity emits pollutants such as gaseous oxides of sulfur (SO2) and nitrogen (NOx) and the greenhouse gas CO2. Co-firing technology simultaneously fires coals with biomass in a coal-fired boiler. It generates “green” power by utilizing renewable energy resources and reduces coal CO2 emission since biomass is renewable and carbon neu- tral [1,2]. In addition, most biomass has little or no sul- fur or nitrogen, therefore co-firing could lower SO2 and NOx levels. These co-firing advantages were demon- strated in most of the co-firing tests in Europe and the United States (depending on biomass used) [3-6]. The tests were at low biomass fraction (typically 20% or less) [3,4], and wood was the predominant biomass compo- nent [7,8]. Oxy-fuel combustion and integrated gasification com- bined cycle (IGCC) with CO2 capture (pre-combustion) technologies may have potential for capturing the green- house gas carbon dioxide at lower costs. In both proc- esses oxygen is used in a coal boiler or gasifier instead of air and produces mainly CO2 and water in the flue gas from oxy-fuel [9] and concentrated carbon dioxide in syngas from gasification [10,11], and thus has a benefit for CO2 capture. Oxy-co-firing and co-gasification of coal with biomass could further reduce effective CO2 emissions and utilize renewable energy resources. Oxy-fuel combustion burns coal in O2/CO2 environ- ments instead of O2/N2 as in conventional co-firing. Simi- larly, the gasification process is also in an O2/CO2 envi- ronment in the gasifier. Pilot and laboratory scale ex- periments have revealed some differences in oxy-fuel combustion and conventional air combustion, including effects such as delayed ignition and reduced flame tem- *Corresponding author. C opyright © 2012 SciRes. IJCCE
 P. WANG ET AL. 36 perature [12,13]. Higher heat capacity of CO2 and lower oxygen diffusion rate in CO2 compared to N2 contribute to these effects. In addition, the oxy-fuel combustion pro- cess is affected by fuel properties [14], and differences between biomass and coal are expected to have addi- tional significant impact. To accelerate the deployment of commercial oxy-fuel and gasification power plants, the development of advanced and validated simulation tools such as computational fluid dynamics (CFD) models is a potentially effective appro- ach. These models need to account for a measure of fuel flexibility. This fuel flexibility should not only include the wide range of variability expected in coal compositions but should also include the possibility of coal and biomass co-utilization. The development of improved and vali- dated CFD models requires accurate characterization of the thermal behavior of different fuels in different gas environments. Experimental studies are necessary to un- derstand the mechanisms of the combustion and gasifica- tion processes and to obtain experimental data needed to validate the models. The objectives of this study are to investigate the impact of inert and oxidative gaseous en- vironments on thermal behavior and reactivity of coal, biomass and blends and to study the effect of biomass percentage on coal/biomass blend thermal behavior. 2. Materials and Experiments 2.1. Materials A Powder River Basin (PRB) sub-bituminous coal and a yellow pine wood sawdust pelletized material were selected for this study. These feedstocks were obtained from the US Department of Energy’s National Carbon Capture Center (NCCC) managed by Southern Company. The re- ceived PRB coal was already ground and dried, and is a subsample of the material that is fed in their operating plant. The wood pellets are cylindrical in shape with a diameter of 8 mm and 32 mm long. They were pro- duced from southern yellow pine with less than 1 wt% bark (no added chemicals) by Green Circle Bio Energy, Inc. The proximate, ultimate and ash mineral analyses results listed in Table 1 were provided by Southern Com- pany. Wood pellet samples were ground using a high speed rotary mill. Both coal and the ground wood were sifted with a sifter and finally dried in an oven. The par- ticle size fraction of 100 to 300 μm was used for all ex- periments. The proximate, ultimate and ash composition analyses of prepared samples were obtained. The analy- sis results of received and prepared samples are similar (data not shown) so the prepared samples are expected to be representative of the received materials. The wood has dramatically different properties compared to the coal. It has high volatility, low contents of S and N, low heating value and high potassium. 2.2. Experiments The reactivity and thermal behavior of PRB coal, wood, and blends of 10% and 20% (wt.) wood in coal were stud- ied in inert gas N2 and oxidizing gases of CO2 and 10% O2 in CO2 (O2:CO2 = 1:9 by volume). The tests were con- ducted by a using non-isothermal method in a PerkinEl- mer Pyris 1 thermogravimetric analyzer (TGA). Samples of approximately 10 mg fuel were heated from room tem- perature to 100˚C at 20˚C/min and held at 100˚C for 20 min; the samples then heated to 1000˚C at 20˚C/min. The same gas was used for purge and process gas flow with a total flow rate of approximately 125 ml/min. The experi- ments were performed in triplicate (quadruplicate or more for 10% O2 in CO2, particularly for samples with wood) to assess their reproducibility. The TGA thermograms recorded percent sample weight as a function of measured sample temperature (or time). The first derivative of the thermal curves (DTG) was cal- culated for each thermogram to facilitate identification of multi-step processes. The thermal behavior has been char- acterized by calculating the percent weight loss, ∆W, for Table 1. Proximate, ultimate and ash composition of powd e r river basin (P RB) sub-bituminous c oal and w ood*. Proximate analysis (% dry basis) Ultimate analysis (% dry basis) HHV (Btu/lb) volatile matter fixed carbon ash C H N S O(diff) (dry basis) PRB coal 40.83 50.34 8.83 67.24 4.23 1.53 0.38 17.79 11,439 Wood pellet 85.19 13.40 1.42 53.20 6.24 0.12 0.02 39.00 8839 Ash mineral analysis (oxides and ignited % wt.) Aluminum Barium Calcium Iron MagnesiumManganesePhosphorous PotassiumSiliconSodium Strontium SulfurTitanium PRB coal 16.00 0.52 19.24 5.52 4.68 - 0.96 0.75 38.711.22 0.23 10.691.08 Wood pellet 13.80 0.21 21.20 4.15 6.12 2.57 1.74 4.06 37.801.07 0.30 5.500.77 *provided by Southern Company. Copyright © 2012 SciRes. IJCCE
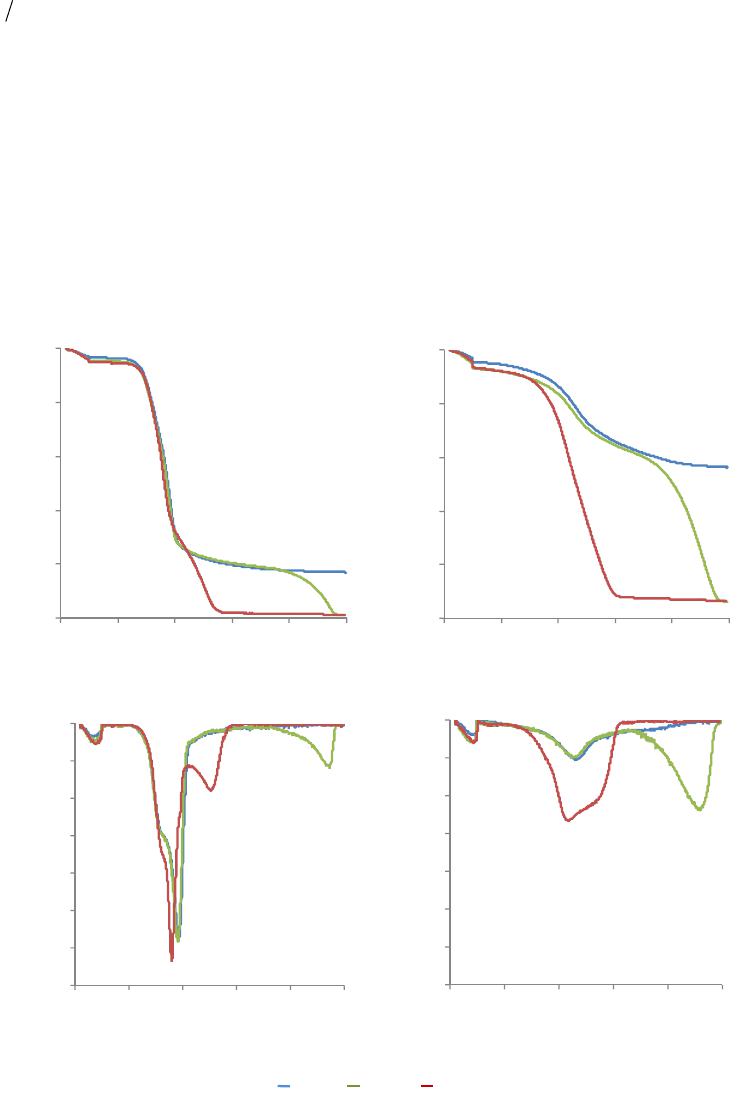 P. WANG ET AL. 37 each thermal process (dehydration, devolatilization/py- rolysis, gasification and/or combustion) and the tempera- ture of maximum rate of weight loss (Tmax) for each well- separated weight loss feature, as well as the final percent- age of residue remaining at end of each experiment. The percent final residue or solid yield (dry basis) is calcu- lated as f 100 mm*100 , where mf and m100 are the weights of the feedstock sample after heating to 1000˚C and after drying at100˚C, respectively. Interactions of wood and coal in these gas environments and blend percentages are checked by comparison of ex- perimental thermal curves and calculated weight loss pro- files of blends based on weight loss of parent fuels and the ratio of blending. The calculated weight loss profile for the blend is given by lend,i woodwood,iwoodcoal,i Wr*W 1rW, where rwood is the weight faction of wood in the blends, and Wwood,i and Wcoal,i are the percentage sample weight of wood and coal, respectively, at temperature i. If the experimental weight loss was not significantly different from the cal- culated one, this indicates that synergistic effects be- tween coal and wood are absent at the selected experi- ment conditions. In this case, the weight loss of blends can be predicted by a linear relation of parent fuel weight loss properties and the percentage wood in the blends. 3. Results and Discussion 3.1. Thermal Events, Thermal Behavior and Reactivity of Coal and Wood in N2, CO2 and 10% O2 in CO2 Figure 1 shows curves of the weight loss (TG) and weight loss rates (DTG) of the wood and coal samples as func- tion of temperature in inert (N2) and oxidizing gases of CO2 and 10% O2 in CO2. The weight loss below 101˚C is attributed to moisture loss from the samples in all gases. In N2, devolatilization/pyrolysis took place as expected. 0 20 40 60 80 100 0200 400 600 8001000 Weight (%) Temp erature ( o C) 0 20 40 60 80 100 0200400 6008001000 Weight ( %) Tempe rature ( o C) (a) (b) -0. 35 -0. 30 -0. 25 -0. 20 -0. 15 -0. 10 -0. 05 0.00 0200 400 600 8001000 Weight loss rate (% s -1 ) Temperature ( o C) -0.35 -0.30 -0.25 -0.20 -0.15 -0.10 -0.05 0.00 0200 400 600 8001000 Weight loss rate (% s-1) Tempe rature (oC) (c) (d) Legend: 10% O 2 in CO 2 CO 22 Figure 1. The sample weight percent and derivative weight loss curves (TG and DTG) of yellow pine wood ((a) and (c)) and PRB coal ((b) and (d)) in N2, CO2, and 10%O2 in CO2. Copyright © 2012 SciRes. IJCCE
P. WANG ET AL. 38 The weight loss is due to release of gases (such as CO, H2, CH4, CO2 and H2O) and tars as volatile matter (VM) by thermal rupture of chemical bonds in the fuel. The residual solid char is essentially carbon (fixed carbon, FC) with mineral matter and some of the nitrogen and sulfur [15]. The DTG curve for coal pyrolysis has a broad peak with higher temperature Tmax = 463˚C ± 2˚C and lower maximum weight loss rate Rmax = 5.2 × 10–2% s−1 com- pared to wood which has a sharp peak in the derivative with lower temperature of maximum rate of weight loss, Tmax = 385˚C ± 2˚C, and higher maximum loss rate Rmax = 2.9 × 10–1% s−1. As expected, coal pyrolysis gives higher percent char yield Ychar = 56.4 ± 0.2 (wet basis) and lower volatiles Yvol = 39.0 ± 0.3 (wet basis) compared to wood Ychar = 17.0 ± 0.2 and Yvol = 79.6 ± 0.2 (wet basis). In CO2, total weight losses at 1000˚C for coal and wood are higher than in N2 due to the combination of CO2 char gasification and devolatilization (Figure 1). These two process steps are clearly seen in the DTG curves. De- volatilization occurs similarly to that seen in N2 and is followed by char gasification at the higher temperatures. The char gasification is mainly via the Boudouard reac- tion [15]. It takes places at lower temperature, Tmax = 918˚C ± 4˚C, and at higher rates Rmax = 1.2 × 10–1% s−1 for coal compared to wood Tmax = 946˚C ± 5˚C and Rmax =5.6 × 10–2% s−1. This is in agreement with the studies by Rathnam et al. [16], who used coals in a drop tube reactor (DTR) at 1400˚C and Al-Mark- hadmeh and Scheffknecht [17] who examined coals in an entrained flow reactor (EFR) at temperatures from 700˚C to 1150˚C. However, the opposite results were obtained in a DTR for coals at 1300˚C by Borrego and Alvarez [18] and for biomass at 950˚C by Borrego et al. [19]; they attributed this to CO2 participation in cross-linking reac- tions at the surface of the devolatilizing particles. 2 Cs CO2CO In 10% O2 in CO2, total weight losses at 1000˚C for coal and wood are the same as in CO2 and higher than in N2 due to mainly char combustion (Figure 1). The fuel thermal decomposition process has two main steps based on DTG curves. Devolatilization and volatile combustion are followed by char combustion, which are clearly shown in two main regions of weight loss for wood but over- lapped in one broad feature for coal. The weight loss in the char combustion is similar to that observed in the char gasification, which is clearly seen by comparing the ther- mal curves in CO2 and in the mixture of oxygen and CO2 for wood. In the presence of oxygen, devolatilization rates are the same as with N2 at lower temperatures and then faster than in N2 and CO2 environments at a slightly higher temperatures. This is likely due to volatiles combustion. Above a certain temperature, once volatiles are released from the solid, these compounds undergo oxidation within the gas film surrounding the particle and result in particle temperature increases. 3.2. Effect of Biomass Percentage on the Reactivity and Thermal Behavior of Coal/Biomass Blends Figure 2 shows TG and DTG curves of the coal, wood, 10% and 20% wood in coal blends as function of tem- perature in oxidizing gases of CO2 (a) and 10% O2 in CO2 (b). TG and DTG curves of the blends in N2 are the same as in CO2 except without the gasification event. The weight losses of the blends are higher than coal alone in both the devolatilization and char gasification regions but same with coal in combustion region. Devolatilization oc- curs over a wider temperature range in CO2 than in 10% O2 in CO2. The dried blends have two different regions of weight loss. In the lower temperature range (T < 415˚C in N2 and CO2 and T < 390˚C in 10% O2 in CO2), the trends of weight loss rates for blends are similar to that of wood. In the higher temperature region, however, they are quite close to coal since wood has low fixed carbon, and there is a low ratio of wood in these blends. Simi- larly, the blends have temperatures for maximum rate of weight loss (Tmax) close to that of wood in the lower temperature region, and comparable to coal in the higher temperature region (Table 2). The weight loss rates of blends are higher than coal alone in all three gases in the lower temperature region (Figure 2) due to high volatile matter (VM) (Table 1) and reactivity of wood. In CO2, the weight loss rate of the 10% wood blend at Tmax = 385˚C (6.7 × 10–2% s−1) is 2.7 times higher than for coal at the same temperature (2.5 × 10–2% s−1). However, in 10% O2, the rate at Tmax = 374˚C (7.7 × 10–2% s−1) is only 1.3 times higher than coal (6.0 × 10–2% s−1) at that tem- perature. In addition, the 20% wood blends have higher weight loss rates than the 10% wood blends in N2 and CO2 but both blends have similar weight loss rates in 10% O2 in CO2. As described in Section 2.2, the final residues of blends after heating to 1000˚C were calculated based on final resi- dues (dry basis) of parent fuels and ratio of blending. These are compared with experimental results in Table 3. There is no significant difference between experimental and calculated final residues of blends. As discussed above, the wood affects the blends more in the lower tempera- ture ranges. So, a similar comparison of calculated weight loss to observed experimental loss was performed in the low temperature range from 100˚C to 415˚C. As can be seen in Figure 3, there are no significant differences be- tween the weight losses from experiments and calcula- tion in N2 and CO2 but the two values do appear to be slightly different in 10% O2 in CO2. However, these dif- ferences are quite small and may not be statistically sig- nificant. As described in Section 2.2, TG curves of blends were calculated based on weight loss profiles of the parent fuels Copyright © 2012 SciRes. IJCCE
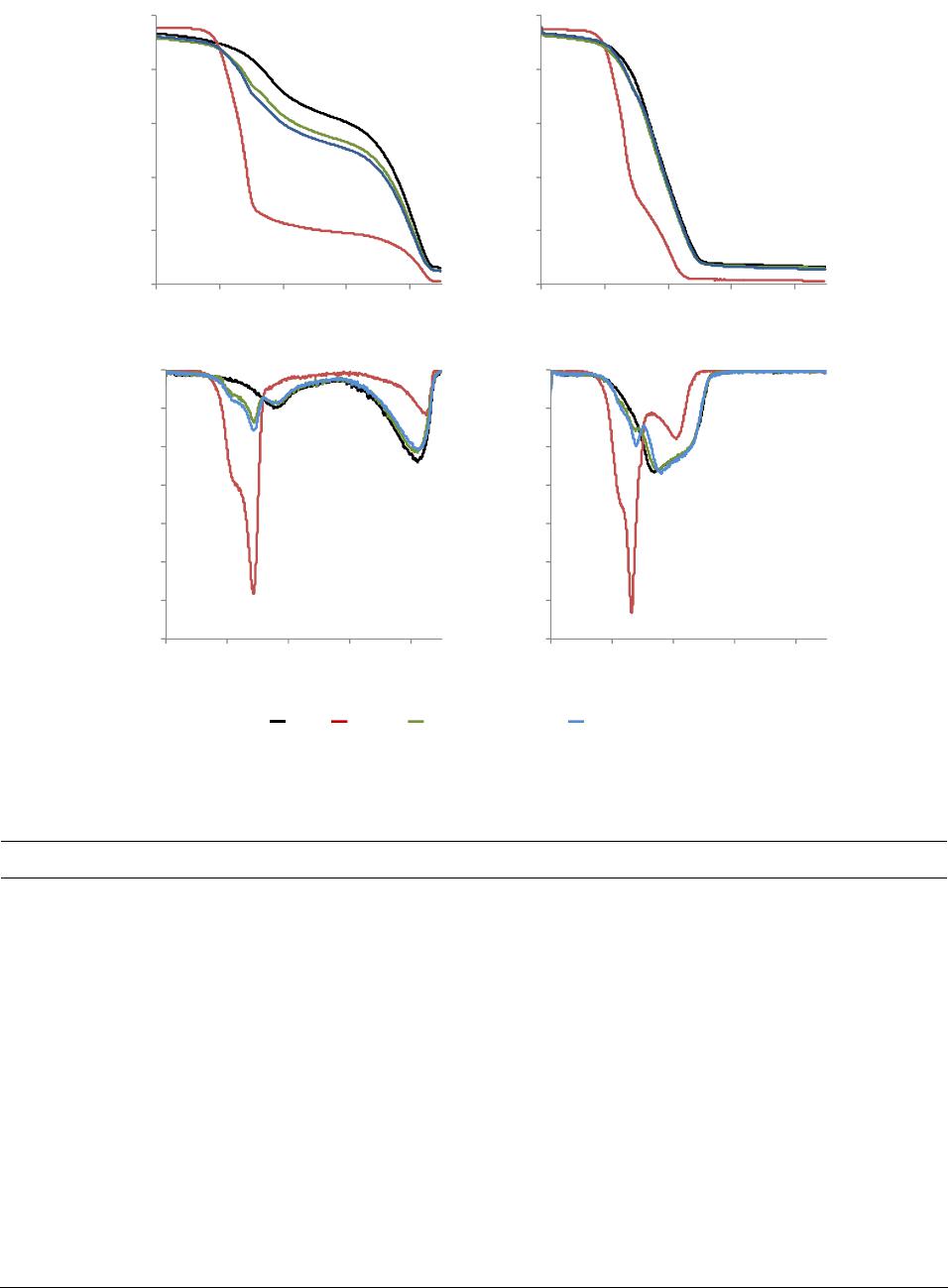 P. WANG ET AL. 39 0 20 40 60 80 100 100 300 500 700900 Weight (%) Temperatu re ( o C) 0 20 40 60 80 100 100 300 500 700900 Weight (%) Temperatu re ( o C) (a) (b) -0.35 -0.30 -0.25 -0.20 -0.15 -0.10 -0.05 0.00 100 300 500 700900 Weight loss rate (% s -1 ) Temp erat ure ( o C) -0.35 -0.30 -0.25 -0.20 -0.15 -0.10 -0.05 0.00 100 300 500 700900 Weight loss rate (% s -1 ) Temp erat ure ( o C) (c) (d) Legend: wood coal 20 % wood in blend 10% wood in blend Figure 2. The percent sample weight and derivative weight loss curves (TG and DTG) of PRB coal, yellow pine wood, 10 wt% and 20 wt% wood in blend in CO2 (a) and 10% O2 in CO2 (b). Table 2. Maximum temperatures of PRB coal, yellow pine wood and their blends in N2, CO2, and 10% O2 in CO2. Gas Sample Maximum temperature (˚C) N2 devolatilization PRB coal 463 ± 2 10 wt% wood in blend 380 ± 4 20 wt% wood in blend 379 ± 2 Wood pellet 385 ± 2 CO2 devolatilization char gasification PRB coal 457 ± 7 918 ± 4 10 wt% wood in blend 385 ± 4 914 ± 6 20 wt% wood in blend 382 ± 3 913 ± 13 Wood pellet 383 ± 1 946 ± 5 devolatilization/ volatile combustion char combustion 10% O2/CO2 PRB coal 432 ± 4 10 wt% wood in blend 440 ± 11 20 wt% wood in blend 453 ± 7 Wood pellet 369 ± 13 510 ± 12 Copyright © 2012 SciRes. IJCCE
 P. WANG ET AL. 40 Table 3. Comparison of final residues (dry base) of PRB coal, yellow pine wood and their blends after heating to 1000˚C in N2, CO2, and 10% O2 in CO2. Calculated values are based on parent fuel values and blending ratios as described above in section 2.2. Samples Final Residue (%) N 2 CO2 10% O2/CO2 PRB coal 59.1 ± 0.2 6.6 ± 0.3 7.1 ± 0.1 Wood pellet 17.6 ± 0.2 1.3 ± 0.1 1.2 ± 0.2 10 wt% wood in blend 54.3 ± 1.1 5.5 ± 0.3 6.7 ± 0.5 20 wt% wood in blend 48.0 ± 2.3 5.3 ± 0.5 5.1 ± 0.5 Calculation based on parent residues and ratio of blend 10 wt% wood in blend 55.0 6.1 6.5 20 wt% wood in blend 50.8 5.5 5.9 0 5 10 15 20 25 30 35 40 N2CO210% O2 in CO2 Weight loss (%) Gas environments coal cal. 10 % wood/coal exp. 10 % wood/coal cal. 20 % wood/coal exp. 20 % wood/coal Figure 3. Comparison of experimental weight loss (dry basis) of coal and wood blends in N2, CO2, 10% O2 in CO2 from ex- periments and calculated weight loss base d on parent fuel thermograms and ratio of blends in 100˚C - 415˚C. and the ratio of fuel blending for N2, CO2 and 10% O2 in CO2 environments. Comparison of these calculated curves with the experimental TG curves showed no sig- nificant differences (Figure 4. Note, N2 data not shown). Overall, there appear to be no significant interaction ob- servable from the weight loss profiles alone between coal and biomass in the solid phase during co-pyrolysis, co- gasification, and co-combustion in this study. These may be explained by the low ratio of wood char in the blend char. Based on the char yields (final residues) of coal and wood from the experimental results in N2, the ratio of wood char contribution to the total blend char for 10 and 20 wt% wood blends is estimated to be 3.2% and 6.9%, respec- tively. This result is in agreement with the results ob- tained by Biagini et al. [20], Vuthaluru [21], and Mogh- taderi et al. [22] on co-pyrolysis and Gil et al. [23] on co-combustion using TGA. For co-pyrolysis the same re- sults are obtained using a drop tube furnace [22]. To shed light on the issue of possible reaction between coal and biomass or synergetic effect of biomass on coal, further study is need because of the inherent heterogeneity of wood and coal, the small sample size, and the small number of replicate determinations used in the present study. 4. Conclusion Oxy-co-firing (combining biomass co-firing and oxy-fuel technologies) and co-gasification of coal and biomass to- gether could further reduce effective CO2 emissions and utilize renewable energy resources. These processes gas- ify and combust fuels in concentrated CO2 and O2/CO2 instead of N2 and O2/N2. The present study investigates the impact of biomass percentage on the thermal behav- ior of coal and biomass blends in inert (N2) and oxidizing gases (CO2 and 10% O2/CO2). The PRB sub-bituminous coal, yellow pine wood pellets, and blends of coal with Copyright © 2012 SciRes. IJCCE
 P. WANG ET AL. 41 0 20 40 60 80 100 100 300 500 700 900 Weight ( %) Tempe rature ( o C) 0 20 40 60 80 100 100 300 500 700 900 Weight (%) Tempe rature ( o C) (a) (b) Legends: cal. 10 % wood in blend exp. 10 % wood in blend cal. 20 % wood in blen ex . 20 % wood in blen Figure 4. Comparison of TG thermograms of coal and wood blends from experiments and calculated based on parent fuel thermograms and ratio of blends, in CO2 (a) and in 10% O2/CO2 (b). up to 20 weight percent of this wood are being studied using thermogravimetry for temperatures up to 1000˚C. Different thermal events of pyrolysis, gasification and combustion takes place in different temperature ranges in these three gaseous environments. In the presence of oxy- gen, devolatilization rates are faster than in N2 and CO2 environments due to the volatiles combustion. The total fuel weight losses at 1000˚C for coal, wood and blends were higher in CO2 than in N2 due to CO2 char gasifica- tion in addition to the devolatilization. Char combustion in 10% O2 in CO2 takes place at lower temperature than char gasification in CO2. The blending of wood increases fuel weight loss rate in the lower temperature range in all gases due to the higher volatile matter content and nar- rower temperature range of devolatilization for wood com- pared to coal. Thermal processes for coal/wood blends can be divided into two reaction regions by temperature. In the lower temperature range, the blend thermal behavior is more like that of the biomass, and in the higher tempera- ture range, it is similar to coal. There appear to be no sig- nificant interactions between coal and biomass observable by thermal degradation weight loss profiles for the blends with low wood percentage in N2, CO2 and 10% O2/CO2. REFERENCES [1] L. Baxter, “Biomass-Coal Co-Combustion: Opportunity for Affordable Renewable Energy,” Fuel, Vol. 84, No. 10, 2005, pp. 1295-1302. doi:10.1016/j.fuel.2004.09.023 [2] A. Demirbaş, “Sustainable Cofiring of Biomass with Coal,” Energy Conversion and Management, Vol. 44, No. 9, 2003, pp. 1465-1479. doi:10.1016/S0196-8904(02)00144-9 [3] K. R. G. Hein and J. M. Bemtgen, “EU Clean Coal Tech- nology—Co-Combustion of Coal and Biomass,” Fuel Processing Technology, Vol. 54, No. 1-3, 1998, pp. 159- 169. doi:10.1016/S0378-3820(97)00067-2 [4] E. E. Hughes and D. A. Tillman, “Biomass Cofiring: Status and Prospects 1996,” Fuel Processing Technology, Vol. 54, No. 1-3, 1998, pp. 127-142. doi:10.1016/S0378-3820(97)00064-7 [5] P. J. Hus and D. A. Tillman, “Cofiring Multiple Opportu- nity Fuels with Coal at Bailly Generating Station,” Bio- mass and Bioenergy, Vol. 19, No. 6, 2000, pp. 385-394. doi:10.1016/S0961-9534(00)00050-7 [6] D. A. Tillman, “Annual Report on Biomass Cofiring Pro- gram 2001,” EPRI, Report 1004601, Palo Alto. [7] D. A. Tillman, E. Hughes and S. Plasynski, “Commer- cializing Biomass-Coal Cofiring: The Process, Status, and Prospect,” 16th Annual International Pittsburgh Coal Conference, Pittsburgh, 1999, pp. 1-11. [8] P. Wang, E. Shuster, et al., “Selection of Biomass Type for Co-Gasification Studies,” Proceeding of the 35th In- ternational Technical Conference on Clean Coal and Fuel systems, Clearwater, 6-10 June 2010. [9] M. B. Toftegaard, J. Brix, P. A. Jensen, P. Glarborg and A. D. Jensen, “Oxy-Fuel Combustion of Solid Fuels,” Pro- gress in Energy and Combustion Science, Vol. 36, No. 5, 2010, pp. 581-625. doi:10.1016/j.pecs.2010.02.001 [10] DOE/NETL (Department of Energy/National Energy Te- chnology Laboratory), “Cost and Performance Baseline for Fossil Energy Power Plants Study, Volume 1: Bitumi- nous Coal and Natural Gas to Electricity,” DOE/2010/ 1397, 2010. [11] T. J. Falcke, A. F. A. Hoadley, D. J. Brennan and S. E. Sinclair, “The Sustainability of Clean Coal Technology: IGCC with/without CCS,” Process Safety and Environ- mental Protectio n, Vol. 89, No. 1, 2011, pp. 41-52. doi:10.1016/j.psep.2010.08.002 [12] T. Wall, Y. Liu, C. Spero, L. Elliott, S. Khare, R. Rathnam, Copyright © 2012 SciRes. IJCCE
 P. WANG ET AL. 42 F. Zeenathal, B. Moghtaderi, B. Buhre, C. Sheng, et al., “An Overview on Oxyfuel Coal Combustion-State of the Art Research and Technology Development,” Chemical Engineering Research and Design, Vol. 87, No. 8, 2009, pp. 1003-1016. doi:10.1016/j.cherd.2009.02.005 [13] L. M. Romeo, L. I. Díez, I. Guedea, et al., “Design and Operation Assessment of an Oxyfuel Fluidized Bed Com- bustior,” Experimental Thermal and Fluid Science, Vol. 35, No. 3, 2011, pp. 477-484. doi:10.1016/j.expthermflusci.2010.11.011 [14] B. Arias, C. Pevida, F. Rubiera and J. J. Pis, “Effect of Biomass Blending on Coal Ignition and Burnout during Oxy-Fuel Combustion,” Fuel, Vol. 87, No. 12, 2008, pp. 2753-2759. doi:10.1016/j.fuel.2008.01.020 [15] L. D. Smoot and P. J. Smith, “Coal Combustion and Gasi- fication,” Plenum Publishing Corporation, New York, 1985. [16] R. K. Rathnam, L. K. Elliott, et al., “Differences in reac- tivity of pulverised coal in air (O2/N2) and oxy-fuel (O2/CO2) conditions,” Fuel Processing Technology, Vol. 90, No. 6, 2009, pp. 797-802. doi:10.1016/j.fuproc.2009.02.009 [17] L. Al-Markhadmeh, J. Maier and G. Scheffknecht, “Coal Pyrolysis and Char Combustion under Oxy-Fuel Condi- tions,” Proceeding of the 34th International Technical Conference on Clean Coal and Fuel System, Clearwater, 31 May-4 June 2009. [18] A. G. Borrego and D. Alvarez, “Comparison of Chars Obtained under Oxy-Fuel and Conventional Pulverized Coal Combustion Atmospheres,” Energy & Fuels, Vol. 21, No. 6, 2007, pp. 3171-3179. doi:10.1021/ef700353n [19] A. G. Borrego, L. Garavaglia and W. D. Kalkreuth, “Characteristics of High Heating Rate Biomass Chars Prepared under N2 and CO2 Atmospheres,” International Journal of Coal Geology, Vol. 77, No. 3-4, 2009, pp. 409- 415. doi:10.1016/j.coal.2008.06.004 [20] E. Biagini, F. Lippi, L. Petarca and L. Tognotti, “Devola- tilization Rate of Biomasses and Coal-Biomass Blends: An Experimental Investigation,” Fuel, Vol. 81, No. 8, 2002, pp. 1041-1050. doi:10.1016/S0016-2361(01)00204-6 [21] H. B. Vuthaluru, “Thermal Behavior of Coal/Biomass Blends during Co-Pyrolysis,” Fuel Processing Technol- ogy, Vol. 85, No. 2-3, 2003, pp. 141-155. doi:10.1016/S0378-3820(03)00112-7 [22] B. Moghtaderi, C. Meesri and T. F. Wall, “Pyrolysis Char- acteristics of Blended Coal and Woody Biomass,” Fuel, Vol. 83, No. 6, 2004, pp. 745-750. doi:10.1016/j.fuel.2003.05.003 [23] M. V. Gil, D. Casal, C. Pevida, J. J. Pis and F. Rubiera, “Thermal Behaviour and Kinetics of Coal/Biomass Blends during Co-Combustion,” Bioresource Technology, Vol. 101, No. 14, 2010, pp. 5601-5608. doi:10.1016/j.biortech.2010.02.008 Copyright © 2012 SciRes. IJCCE
|