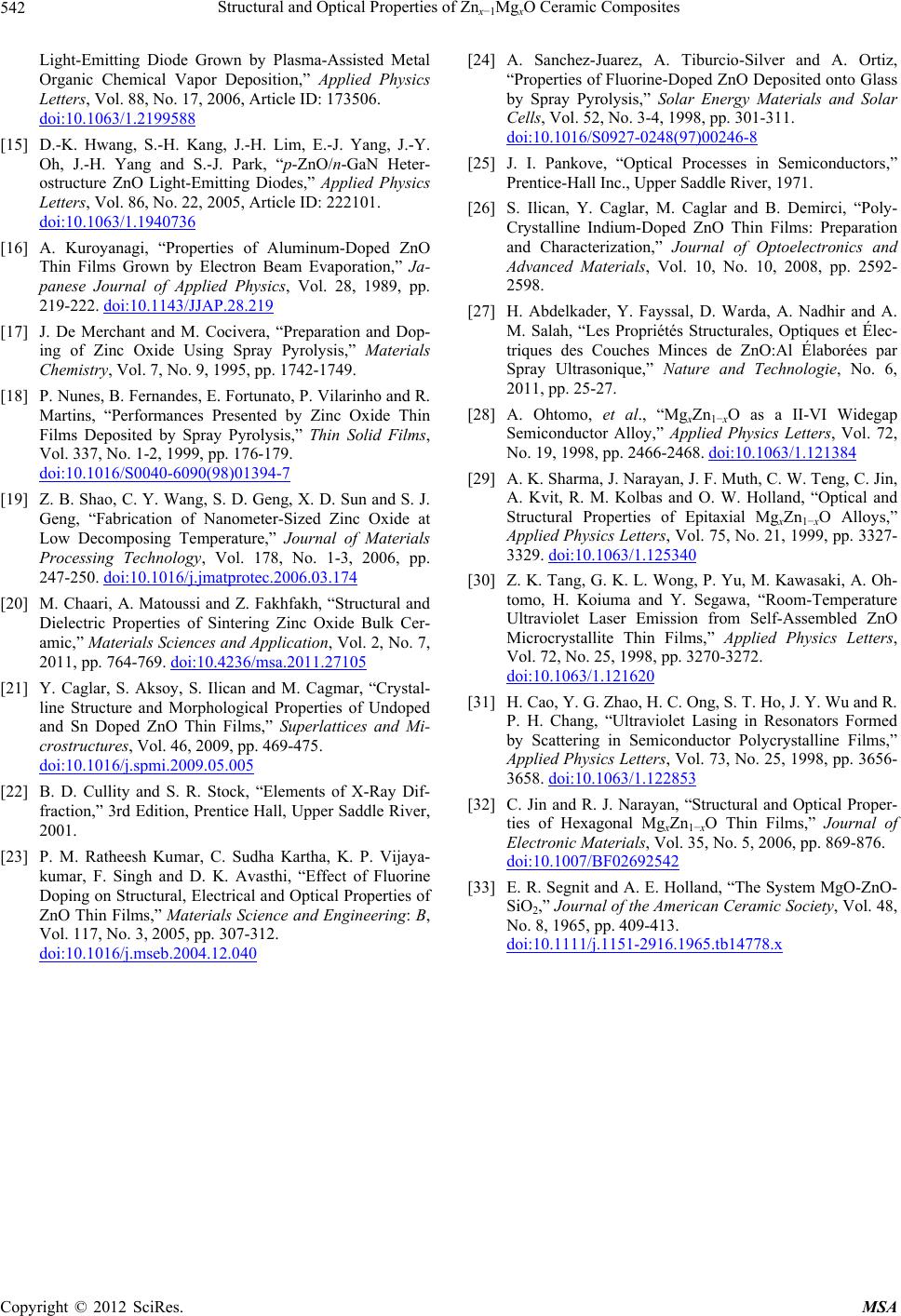
Structural and Optical Properties of Znx−1MgxO Ceramic Composites
Copyright © 2012 SciRes. MSA
542
Light-Emitting Diode Grown by Plasma-Assisted Metal
Organic Chemical Vapor Deposition,” Applied Physics
Letters, Vol. 88, No. 17, 2006, Article ID: 173506.
doi:10.1063/1.2199588
[15] D.-K. Hwang, S.-H. Kang, J.-H. Lim, E.-J. Yang, J.-Y.
Oh, J.-H. Yang and S.-J. Park, “p-ZnO/n-GaN Heter-
ostructure ZnO Light-Emitting Diodes,” Applied Physics
Letters, Vol. 86, No. 22, 2005, Article ID: 222101.
doi:10.1063/1.1940736
[16] A. Kuroyanagi, “Properties of Aluminum-Doped ZnO
Thin Films Grown by Electron Beam Evaporation,” Ja-
panese Journal of Applied Physics, Vol. 28, 1989, pp.
219-222. doi:10.1143/JJAP.28.219
[17] J. De Merchant and M. Cocivera, “Preparation and Dop-
ing of Zinc Oxide Using Spray Pyrolysis,” Materials
Chemistry, Vol. 7, No. 9, 1995, pp. 1742-1749.
[18] P. Nunes, B. Fernandes, E. Fortunato, P. Vilarinho and R.
Martins, “Performances Presented by Zinc Oxide Thin
Films Deposited by Spray Pyrolysis,” Thin Solid Films,
Vol. 337, No. 1-2, 1999, pp. 176-179.
doi:10.1016/S0040-6090(98)01394-7
[19] Z. B. Shao, C. Y. Wang, S. D. Geng, X. D. Sun and S. J.
Geng, “Fabrication of Nanometer-Sized Zinc Oxide at
Low Decomposing Temperature,” Journal of Materials
Processing Technology, Vol. 178, No. 1-3, 2006, pp.
247-250. doi:10.1016/j.jmatprotec.2006.03.174
[20] M. Chaari, A. Matoussi and Z. Fakhfakh, “Structural and
Dielectric Properties of Sintering Zinc Oxide Bulk Cer-
amic,” Materials Sciences and Application, Vol. 2, No. 7,
2011, pp. 764-769. doi:10.4236/msa.2011.27105
[21] Y. Caglar, S. Aksoy, S. Ilican and M. Cagmar, “Crystal-
line Structure and Morphological Properties of Undoped
and Sn Doped ZnO Thin Films,” Superlattices and Mi-
crostructures, Vol. 46, 2009, pp. 469-475.
doi:10.1016/j.spmi.2009.05.005
[22] B. D. Cullity and S. R. Stock, “Elements of X-Ray Dif-
fraction,” 3rd Edition, Prentice Hall, Upper Saddle River,
2001.
[23] P. M. Ratheesh Kumar, C. Sudha Kartha, K. P. Vijaya-
kumar, F. Singh and D. K. Avasthi, “Effect of Fluorine
Doping on Structural, Electrical and Optical Properties of
ZnO Thin Films,” Materials Science and Engineering: B,
Vol. 117, No. 3, 2005, pp. 307-312.
doi:10.1016/j.mseb.2004.12.040
[24] A. Sanchez-Juarez, A. Tiburcio-Silver and A. Ortiz,
“Properties of Fluorine-Doped ZnO Deposited onto Glass
by Spray Pyrolysis,” Solar Energy Materials and Solar
Cells, Vol. 52, No. 3-4, 1998, pp. 301-311.
doi:10.1016/S0927-0248(97)00246-8
[25] J. I. Pankove, “Optical Processes in Semiconductors,”
Prentice-Hall Inc., Upper Saddle River, 1971.
[26] S. Ilican, Y. Caglar, M. Caglar and B. Demirci, “Poly-
Crystalline Indium-Doped ZnO Thin Films: Preparation
and Characterization,” Journal of Optoelectronics and
Advanced Materials, Vol. 10, No. 10, 2008, pp. 2592-
2598.
[27] H. Abdelkader, Y. Fayssal, D. Warda, A. Nadhir and A.
M. Salah, “Les Propriétés Structurales, Optiques et Élec-
triques des Couches Minces de ZnO:Al Élaborées par
Spray Ultrasonique,” Nature and Technologie, No. 6,
2011, pp. 25-27.
[28] A. Ohtomo, et al., “MgxZn1−xO as a II-VI Widegap
Semiconductor Alloy,” Applied Physics Letters, Vol. 72,
No. 19, 1998, pp. 2466-2468. doi:10.1063/1.121384
[29] A. K. Sharma, J. Narayan, J. F. Muth, C. W. Teng, C. Jin,
A. Kvit, R. M. Kolbas and O. W. Holland, “Optical and
Structural Properties of Epitaxial MgxZn1−xO Alloys,”
Applied Physics Letters, Vol. 75, No. 21, 1999, pp. 3327-
3329. doi:10.1063/1.125340
[30] Z. K. Tang, G. K. L. Wong, P. Yu, M. Kawasaki, A. Oh-
tomo, H. Koiuma and Y. Segawa, “Room-Temperature
Ultraviolet Laser Emission from Self-Assembled ZnO
Microcrystallite Thin Films,” Applied Physics Letters,
Vol. 72, No. 25, 1998, pp. 3270-3272.
doi:10.1063/1.121620
[31] H. Cao, Y. G. Zhao, H. C. Ong, S. T. Ho, J. Y. Wu and R.
P. H. Chang, “Ultraviolet Lasing in Resonators Formed
by Scattering in Semiconductor Polycrystalline Films,”
Applied Physics Letters, Vol. 73, No. 25, 1998, pp. 3656-
3658. doi:10.1063/1.122853
[32] C. Jin and R. J. Narayan, “Structural and Optical Proper-
ties of Hexagonal MgxZn1−xO Thin Films,” Journal of
Electronic Materials, Vol. 35, No. 5, 2006, pp. 869-876.
doi:10.1007/BF02692542
[33] E. R. Segnit and A. E. Holland, “The System MgO-ZnO-
SiO2,” Journal of the American Ceramic Society, Vol. 48,
No. 8, 1965, pp. 409-413.
doi:10.1111/j.1151-2916.1965.tb14778.x