 Journal of Minerals & Materials Characterization & Engineering, Vol. 11, No.4, pp.335-352, 201 2 jmmce.org Printed in the USA. All rights reserved 335 Effect of Copper and Silicon Carbide C ontent on the Corr osion Resistance of Al-Mg Alloys in Acidic and Alkaline Solution s Ahmad T. Mayyas1, , Mohammad M. Hamasha2, Abdalla Alrashdan2, Adel M. Hassan2, Mohammed T. Hayajneh2 1 Department of Automotive Engineering, Clemson University-International Center for Automotive Research (CU-ICAR), 4 Research Drive, Greenville, South Carolina 29607 USA. 2 Center for Autonomous Solar Power, State University of New York at Binghamton, Binghamton, NY 13850, USA 3 Department of Industrial Engineering, Faculty of Engineering, Jordan University of Science and Technology, P.O. Box 3030, Irbid, 22110, Jordan ∗ Corresponding Author: ahmadm@g.clemson.edu ABSTRACT In this study, corrosion resistance of cast aluminum based alloys and composites reinforced with silicon carbide has been investigated. Different Al-Mg-Cu alloys and Al-4wt.%Mg-Cu/SiC composites reinforced with 5 or 10 vol.%SiC were subjected to corrosive media (acidic: 1.0M HCl and alkaline: 1.0M NaOH) using weight loss method to evaluate their corrosion resistance. The results show that introducing copper and silicon carbide have a negative effect on the corrosion resistance of monolithic aluminum; however, Al-based composites still being attractive materials to replace steel parts in automotive and aircraft industries and hence
 336 A.T. Mayyas, M.M. Hamasha, A. Alra shd a n , A.M. Hassan, M.T. Hayajneh Vol.11, No.4 studying their corrosion resistance is of high importance. Scanning electron microscopy (SEM) and Energy-dispersive X-ray (EDS) were used to show the degree of attack and of acidic and alkaline solution on the surface of the investigated materials. Keywor d s: Casting; Corrosion Testing; Metal Matrix Composites; Surface Engineering. 1. INTRODUCTIO N Aluminum matrix composites (AMCs) refer to the class of light weight high performance aluminum centric material systems. The reinforcement in AMCs could be in the form of continuous/discontinuous fibers, whisker or particulates [1], in volume fractions ranging from a few percent to 60%. Studying corrosion resistance of Al-based materials is important especially for automotive and aircraft applications where the parts are prone to corrosive media like salt water solutions, acidic and alkaline media. Aluminum based composites are usually reinforced b y Al2O3, SiC, and C. The major advantages of AMCs compared to unreinforced materials are as follows: greater strength, improved stiffness, reduced density, good corrosion resistance, improved high temperature properties, controlled thermal expansion coefficient, thermal/heat management, improved wear resistance and improved damping capabilities [1-6] . Nowadays, the increased demand for light weight components, primarily driven by the need to reduce energy consumption in a variety of societal and structural components, has led to an increase in the use of aluminum. Among the various methods to fabricate metal matrix composites, stir casting method has drawn keen attraction among the researchers due to its industrial feasibility. The major limitation in many cases in fabricating metal matrix composites by liquid phase route resides upon the incompatibility of the reinforcement and the matrix [ 1-3]. This problem in case of Al-based metal matrix composite is due to the formation of stable tenacious oxide film, resulting in poor wettability with the ceramic particle. One of the common practices to improve wettability of an Al melt is through addition of small amount of reactive metals like magnesium and titanium prior to the incorporation of ceramic particle [1,3]. However, in the present work, 4 wt% Mg was added to Al to improve wettability and argon flux was used during melting and pouring tasks to reduce oxidation effect.
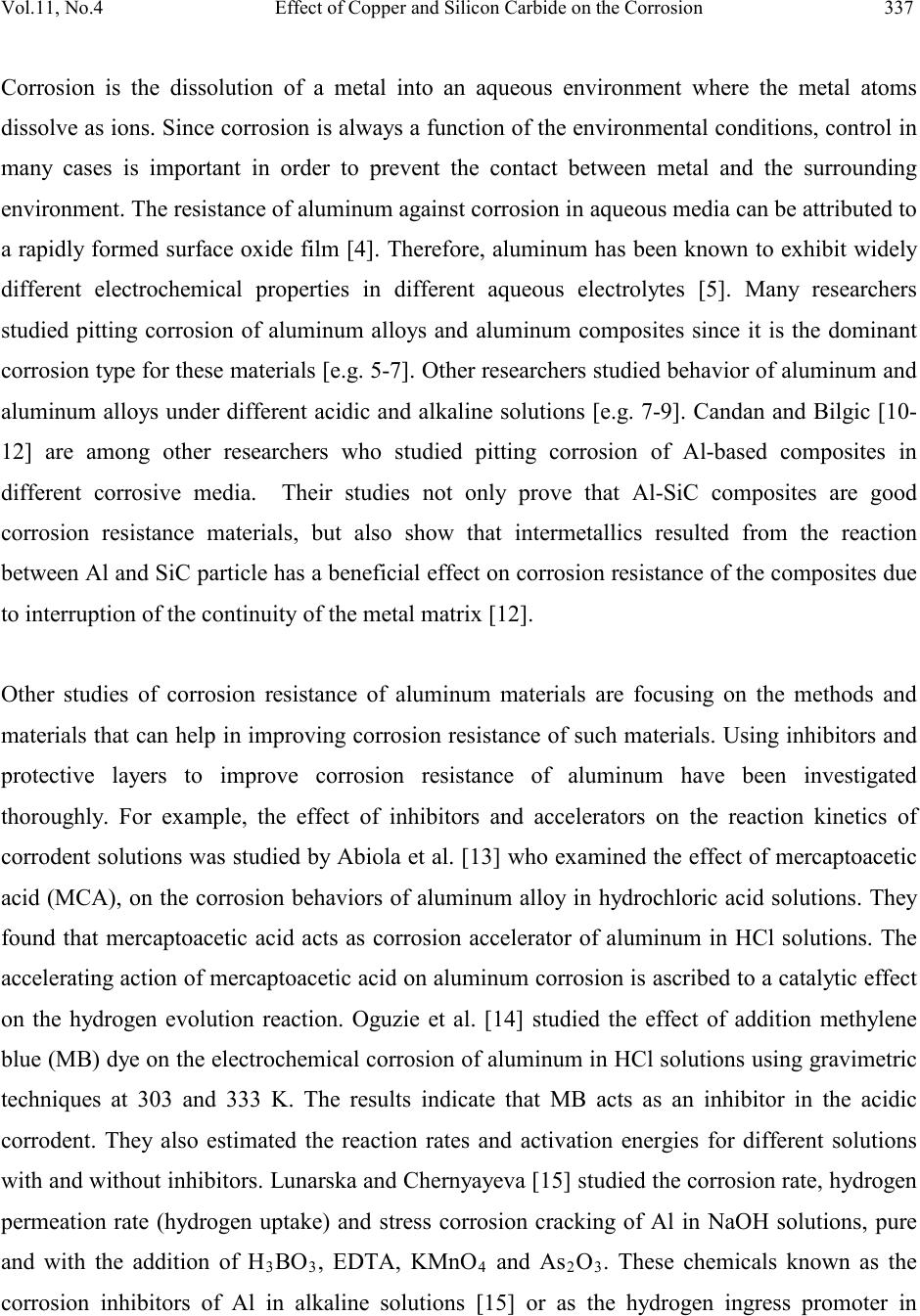 Vol.11, No.4 Effect of Copper and Silicon Carbide o n the Corrosion 337 Corrosion is the dissolution of a metal into an aqueous environment where the metal atoms dissolve as ions. Since corrosion is always a function of the environmental conditions, control in many cases is important in order to prevent the contact between metal and the surrounding environment. The resistance of aluminum against corrosion in aqueous media can be attri but ed t o a rapidly formed surface oxide film [4]. Therefore, aluminum has been known to exhibit widely different electrochemical properties in different aqueous electrolytes [5]. Many researchers studied pitting corrosion of aluminum alloys and aluminum composites since it is the dominant corrosion type for these materials [e.g. 5-7]. Other researchers studied behavior of aluminum and aluminum alloys under different acidic and alkaline solutions [e.g. 7-9]. Candan and Bilgic [10- 12] are among other researchers who studied pitting corrosion of Al-based composites in different corrosive media. Their studies not only prove that Al-SiC composites are good corrosion resistance materials, but also show that intermetallics resulted from the reaction between Al and Si C particl e has a ben eficial effect o n corros ion res istan ce of t he comp osit es d ue to interruption of the continuity of the metal matrix [12]. Other studies of corrosion resistance of aluminum materials are focusing on the methods and materials that can help in improving corrosion resistance of such materials. Using inhibitors and protective layers to improve corrosion resistance of aluminum have been investigated thoroughly. For example, the effect of inhibitors and accelerators on the reaction kinetics of corrodent solutions was studied by Abiola et al. [13] who examined the effect of mercaptoacetic acid (MCA), on the corrosion behaviors of aluminum alloy in hydrochloric acid solutions. They found that mercaptoacetic acid acts as corrosion accelerator of aluminum in HCl solutions. The acceleratin g action o f mercaptoacetic aci d on alumin um corrosi on is ascribed to a catalytic effect on the hydrogen evolution reaction. Oguzie et al. [14] studied the effect of addition methylene blue (MB) dye on the electrochemical corrosion of aluminum in HCl solutions using gravimetric techniques at 303 and 333 K. The results indicate that MB acts as an inhibitor in the acidic corrodent. They also estimated the reaction rates and activation energies for different solutions with and without inhibitors. Lunarska and Chernyayeva [15] s tudied the corrosion rate, hydrogen permeation rate (hydrogen uptake) and stress corrosion cracking of Al in NaOH solutions, pure and with the addition of H3BO3, EDTA, KMnO4 and As2O3. These chemicals known as the corrosion inhibitors of Al in alkaline solutions [15] or as the hydrogen ingress promoter in
 338 A.T. Mayyas, M.M. Hamasha, A. Alra shd a n , A.M. Hassan, M.T. Hayajneh Vol.11, No.4 electrolyte and the implantation of Al surface with B+ ions inhibited corrosion. Hydrogen uptake was found to be promoted or inhibited by means of studied species, depending on the method of their introduction into the base solution and on the applied polarization. The different studies dealt with the effect of alloying elements on the corrosion resistance of aluminum showed that magnesium tends to enhance corrosion resistance of aluminum while copper has adverse effect on the corrosion resistance of aluminum [16-18]. It was proposed that the concentrations of alloying elements, particularly copper, in Al solid solution are the key factors which could influence the pitting corrosion. Such increase of copper in the aluminum matrix increases the corrosion potential of aluminum matrix [16]. The present study is an attempt to investigate the corrosion behavior of Al-based alloys and composites reinforced with SiC under chemical attacks of acidic and alkaline aqueous media. In this study, two different aqueous solutions were used; namely: 1.0M HCl as acidic environment and 1.0M NaOH as alkaline environment. Such kind of corrosive media might be similar to what automotive and airplane parts face in their service lifetime. 2. EXPERIMENTAL SETUP AND PROCEDURE 2.1 Materials The test materials studied in this work were a mixture of aluminum (commercial grade Al, ~99% purity) and copper granules with an average particle size of 0.425 mm and ~97% purity as a metal matrix. Silicon carbide particles ware added as reinforcement to the metal matrix. About 1000 g of commercial grade Al ingots and different weight percentages of copper powder (0, 1, 2, 3, 4, and 5 wt.%) were used to prepare the base metal matrix by stir casting method. Specific quantities of silicon carbide powder with an average particle size of 75μm and purity exceeds 99.5% of 5 and 10 vol.% were added to the matrix alloy. Magnesium ingots (~99% purity) was added in small quantities (fixed weight percentage 4wt.%) to promote wettability between metal matrix and reinforcement particles [2, 10].
 Vol.11, No.4 Effect of Copper and Silicon Carbide o n the Corrosion 339 2.2 Processing The synthesis of the particulate metal matrix composites used in the present study was carried out by the stir casting method (compocasting method). Aluminum ingots and copper granules melted together at 850 °C. The amount of SiC powder pre-oxidized at 900°C for about 30 minutes to form a layer of SiO 2 on their surface in order to improve their wettability with molten aluminum were incorporated into the melt [1]. Magnesium was added to the melt in the final stage prior to pouring. The pouring temperature was maintained at 580-600 °C in semisolid state. Then the mould was left in air to cool down to room temperature. Finally the produced cast bars were turned to small disks to get the required specimens to be used in corrosion test (specimen dimensions were: 25 mm in diameter and 10 mm in thickness and each specimen has a hole of 8.5 mm in diameter.). The total surface area for each sample was 1920 mm2. 2.3. Weight Loss Measurements For full immersion method the specimens were suspended in glass jar contains the corrodent solution (1.0M solution of HCl or 1.0M solution of NaOH) using plastic string. The corrodent solution was exposed to atmospheric air and its temperature was maintained at 23–25 °C. The weight loss rates were determined by removing specimens from test solutions at 30 minutes intervals with total testing time of 240 minutes. To avoid local crevice corrosion, the specimens were firstly ground with #600 SiC abrasive paper, the specimens were then doubly rinsed under running water, cleaned in ethanol to remove any remaining debris, and to ensure complete dryness of the specimens, they were dried in oven at 70 °C for 20 minutes and then cooled to room temperature prior to weighing using electronic balance having 0.1 mg accuracy. The specimens were subsequently returned to the testing solutions and the procedure repeated progressively for 240 minutes. Cumulative weight loss was established based on the original weight of each specimen. New similar specimens were used in 1.0M NaOH solution to get a relative comparison of the corrosion resistance of the specimens in both acidic and alkaline solutions. The corroded surfaces were then examined using scanning electron microscope (SEM) (Quanta 200) equipped with Energy dispersive X-ray spectroscopy (EDS) (EDAX).
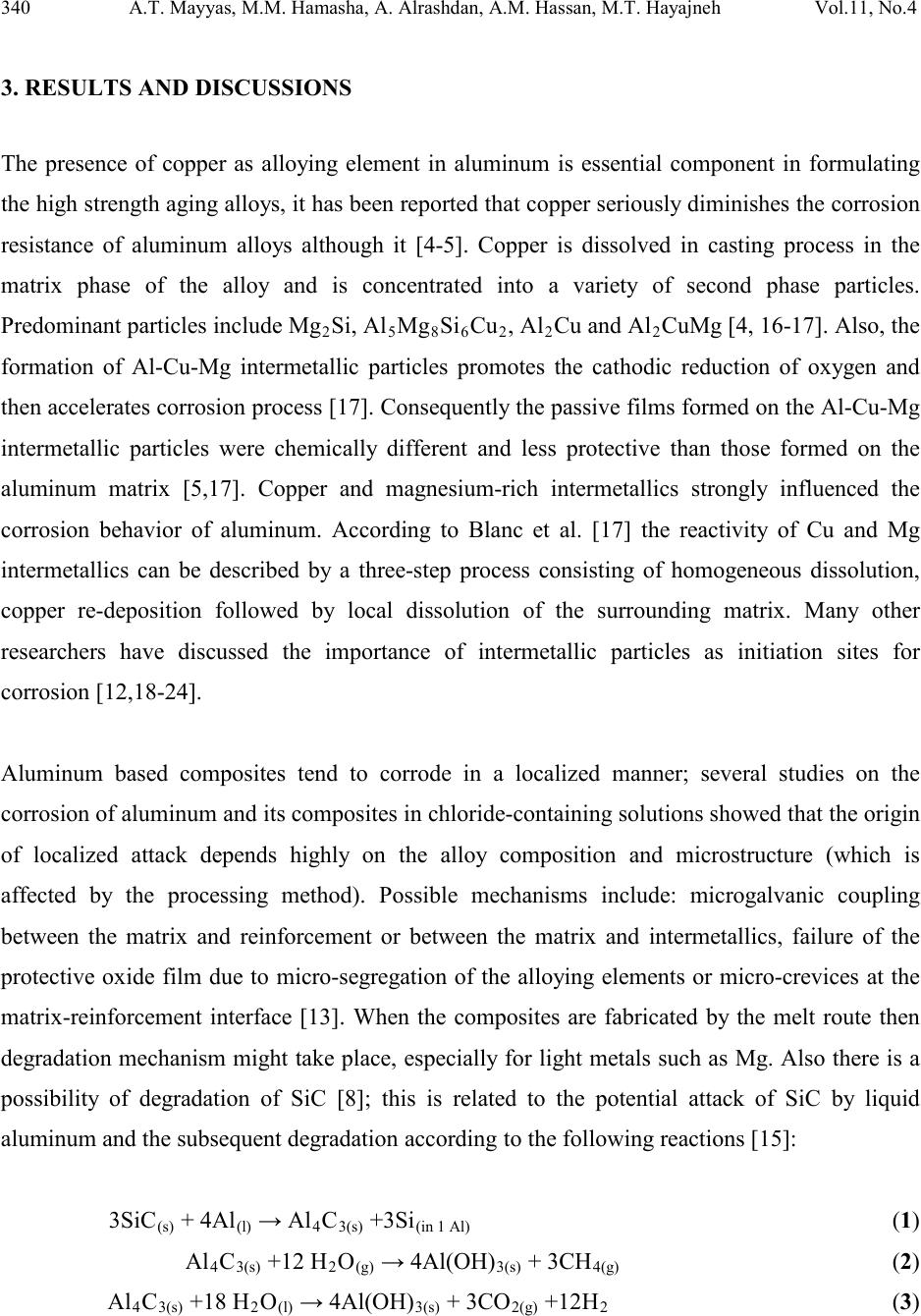 340 A.T. Mayyas, M.M. Hamasha, A. Alra shd a n , A.M. Hassan, M.T. Hayajneh Vol.11, No.4 3. RESULTS AND DISCUSSIONS The presence of copper as alloying element in aluminum is essential component in formulating the high strength aging alloys, it has been reported that copper seriously diminishes the corrosion resistance of aluminum alloys although it [4-5]. Copper is dissolved in casting process in the matrix phase of the alloy and is concentrated into a variety of second phase particles. Predominant particles include Mg2S i, Al5Mg8Si6Cu2, Al2Cu and Al2C uMg [4, 16-17]. Also, the formation of Al-Cu-Mg intermetallic particles promotes the cathodic reduction of oxygen and then accelerates corrosion process [17]. Consequently the passive films formed on the Al-Cu-Mg intermetallic particles were chemically different and less protective than those formed on the aluminum matrix [5,17]. Copper and magnesium-rich intermetallics strongly influenced the corrosion behavior of aluminum. According to Blanc et al. [17] the reactivity of Cu and Mg intermetallics can be described by a three-step process consisting of homogeneous dissolution, copper re-deposition followed by local dissolution of the surrounding matrix. Many other researchers have discussed the importance of intermetallic particles as initiation sites for corrosion [12,18-24]. Aluminum based composites tend to corrode in a localized manner; several studies on the corrosion of aluminum and its composites in chloride-containing solutions showed that the origin of localized attack depends highly on the alloy composition and microstructure (which is affected by the processing method). Possible mechanisms include: microgalvanic coupling between the matrix and reinforcement or between the matrix and intermetallics, failure of the protective oxide film due to micro-segregation of the alloying elements or micro-crevices at the matrix-reinforcement interface [13]. When the composites are fabricated by the melt route then degradation mechanism might take place, especially for light metals such as Mg. Also there is a possibility of degradation of SiC [8]; this is related to the potential attack of SiC by liquid aluminum and the subsequent degradation according to the following reactions [15]: 3SiC(s) + 4Al(l) → Al4C3(s) +3Si(in 1 Al) (1) Al4C3(s) +12 H2O(g) → 4Al(OH)3(s) + 3CH4(g) (2) Al4C3(s) +18 H2O(l) → 4Al(OH)3(s) + 3CO2(g) +12 H2 (3)
 Vol.11, No.4 Effect of Copper and Silicon Carbide o n the Corrosion 341 The intermetallic precipitates that may found during casting process are Al 4C3 and free Si- type intermetallic, the contribution of such hard intermetallic precipitates is to increase corrosion rate in monolithic aluminum alloys and matrix composites [2, 8]. The chemical reaction of Al4C3 with water (even with moisture in the atmosphere at room temperature) and other environments such as HCl and NaOH is thermodynamically favorable and could be detrimental to the composites properties [8,13]. Silicon dioxide (SiO2) as it formed from reaction between aluminum and oxidized silicon carbide particles in Al/SiC composites would have various beneficial effects [8]: i) abating processing costs, ii) preventing the formation of Al4C3 and iii) promoting in situ growth of thermodynamically stable phases (MgO, MgAl 2O4) that may act as reinforcem ents, according to [8] the following reactions may take place: SiO2(s) + 2Mg(l) → 2MgO(s) + Si(in 1 Al ) (4) Mg(l) +2Al(l) +2 SiO2(s) → MgAl2O4 (s) + 2Si (in l Al) (5) 4Al6Si2O13(s) +13 Mg(l) +2Al(l) → 13MgAl2O4 (s) + 8Si (in l Al) (6) In addition to these reactions, the following chemical reaction between molten aluminum and SiO2 is also thermodynamically possible [8]: SiO2(s) + 2Al(l) → 2Al2O3(s) + Si(in 1 Al) (7) Although the rejection of a certain amount of silicon into the alloy matrix is useful to prevent the formation of Al4C3, it may represent a potential problem. Reaction of Si in the alloy (or that formed as a by-product) with magnesium might produce Mg 2S i during solidification of the alloy matrix in the composites. The intermetallic Mg2Si has been reported to be electrochemically active and may enhance localized corrosion [8]. On the other hand, high dislocation densities have been ob served at the Al -SiC matrix interfaces as a result of differential thermal contraction between SiC p arti cles and the Al mat rix [9] . A ls o t he prot ruded S iC part i cles increase t he s urface area which becomes in contact with corroding solution and hence increase the susceptibility of the surface to be corroded.
 342 A.T. Mayyas, M.M. Hamasha, A. Alra shd a n , A.M. Hassan, M.T. Hayajneh Vol.11, No.4 Figures 1 and 2 show the cumulative mass loss of different specimens in 1.0M HCl solution and 1.0M NaOH solution, respectively. The non-uniformity and non-linearity of the plots suggest that the Al corrosion by HCl and NaOH environments is heterogeneous processes. In acidic and alkaline environments the heterogeneous process involving several phases: in the first phase the hard oxide film is damaged by corrodent and in the second phase with increased exposu re time a steady state will be reached as the corrodent reaches the oxide-free matrix; hence it allows equilibrium in the kinetics of dissolution of the outer porous layer and build-up of an inner compact l ayer to be reached aft er an i nt erval of time. Pitting is the predominant form of localized corrosion in this step [6, 9, and 16]. Similar observations have been also noticed in the present study for corrosion of Al in NaOH solution. Fig. 1 Cumulative mass loss of: a) Al-4wt.%Mg-Cu alloys, and b) Al-4wt.%Mg-SiC composites in 1.0M HCl Fig. 2 Cumulative mass loss of: a) Al-4wt.%Mg-Cu alloys, and b) Al-4wt.%Mg-SiC composites in 1.0M NaOH
 Vol.11, No.4 Effect of Copper and Silicon Carbide o n the Corrosion 343 3.1. Thermodynamics Of Corrosion The dissolution of Al- based alloys and composites have shown stronger affection by acidic solution of HCl compared to alkaline solution of NaOH. To compare the effect of dissolution kinetics of Al-based alloys/composites in alkaline and acidic solutions the first order Arrhenius equation: (8) Where Ea is the apparent activation energy which depends on type of material, corrodent and temperature, R is the ideal gas constant (8.314 J.mol-1.K-1), and T is absolute temperature. In its original form the pre-exponential factor A and the activation energy Ea are considered to be temperat u r e-independent. The rate constants can be calculated according to the following equation [13]: (9) wi represents the initial weight of specimen and w f represents t he final weight o f specimen aft er immersion in corrodent solution for 240 minutes and t is time in minutes (i.e. 240 minutes). The half-time (t1/2) was calculated from the first order Arrhenius equation [13]: (10) When ln(wi –Δw) is plotted against time a linear plot is obtained which confirms first order Arrhenius equation as shown in Fig. 3. Arrhenius first order equation was used to estimate the kinetic data like rate constant (K) and half time (t1/2) as shown in Table 1. Fig. 4 has been plotted to manifest the relative corrosion thermodynamics data in Table 1. Fig.4 shows the rate constant and half-time (t1/2) for different alloys and composites in 1.0M HCl and 1.0M NaOH solutions, respectively. The first observation from these figures indicates that the average corrosion rates of Al-based materials in alkaline environment is lower than corrosion rate in acidic environment, however, severe damaged is resulted from the acidic environments. Also, from these figures one can observe that the rate constant varies considerably for Al-based specimens used in 1.0M HCl solution compared to the specimens used in 1.0M NaOH ( in 1.0M HCl rate constant range from 95.98X10-4 for Al-4wt.%Mg to 250.63X10-4 (min-1) for Al- 4wt.%Mg-5wt.%Cu-10vol%SiC which can be compared to the corresponding values in 1.0M
 344 A.T. Mayyas, M.M. Hamasha, A. Alra shd a n , A.M. Hassan, M.T. Hayajneh Vol.11, No.4 NaOH as they ranged from 91.63X10-4 for Al-4wt.%Mg to 120.65X10-4 for Al-4wt.%Mg- 5wt.%Cu-10vol%SiC). Hence it is clear that the acidic solution has stronger effect on the Al- based surfaces when compared to NaOH solutions. Fig. 3 Variation of log (wi -Δw) with time for different samples in: a) 1.0M HCl solution, and b) 1.0M NaOH solution. Fig. 4 Rate constant (K) and half time (t 1/2) for different alloys and composites in: a) 1.0M HCl solution, and b) 1.0M NaOH solution
 Vol.11, No.4 Effect of Copper and Silicon Carbide o n the Corrosion 345 Table 1 Thermodynamic data of different alloys and composite specimens used in this study -6 -6 3.2. Surface Characterization The specimens were exposed to corrodent solutions for 240 minutes before they examined using scanning electron microscope (SEM) and energy dispersive X-ray (EDS). Fig. 5 shows photographs of the surface of some corroded specimens investigated in this work. Visual inspection with the aid of this figure shows that the HCl solution has stronger effect on the surface of Al-based materials than the NaOH solution. However, such kind of subjective judgment is rather important, but at least it gives us a feeling of what is happening in the corrosion test. During the testing period and especially in the initial periods, pitting corrosion is the dominant type of corrosion followed by formation of white and light-gray powders on the specimen surfaces. The amount of that powder was observed significantly in the 1.0M NaOH solution. However, polished surfaces were obtained after the termination of the corrosion test in 1.0M NaOH as shown in Fig. 5. In 1.0M HCl solution it was observed continuous pitting corrosion of surface with decreasing corrosion rate due to the etching effect of acidic solutions.
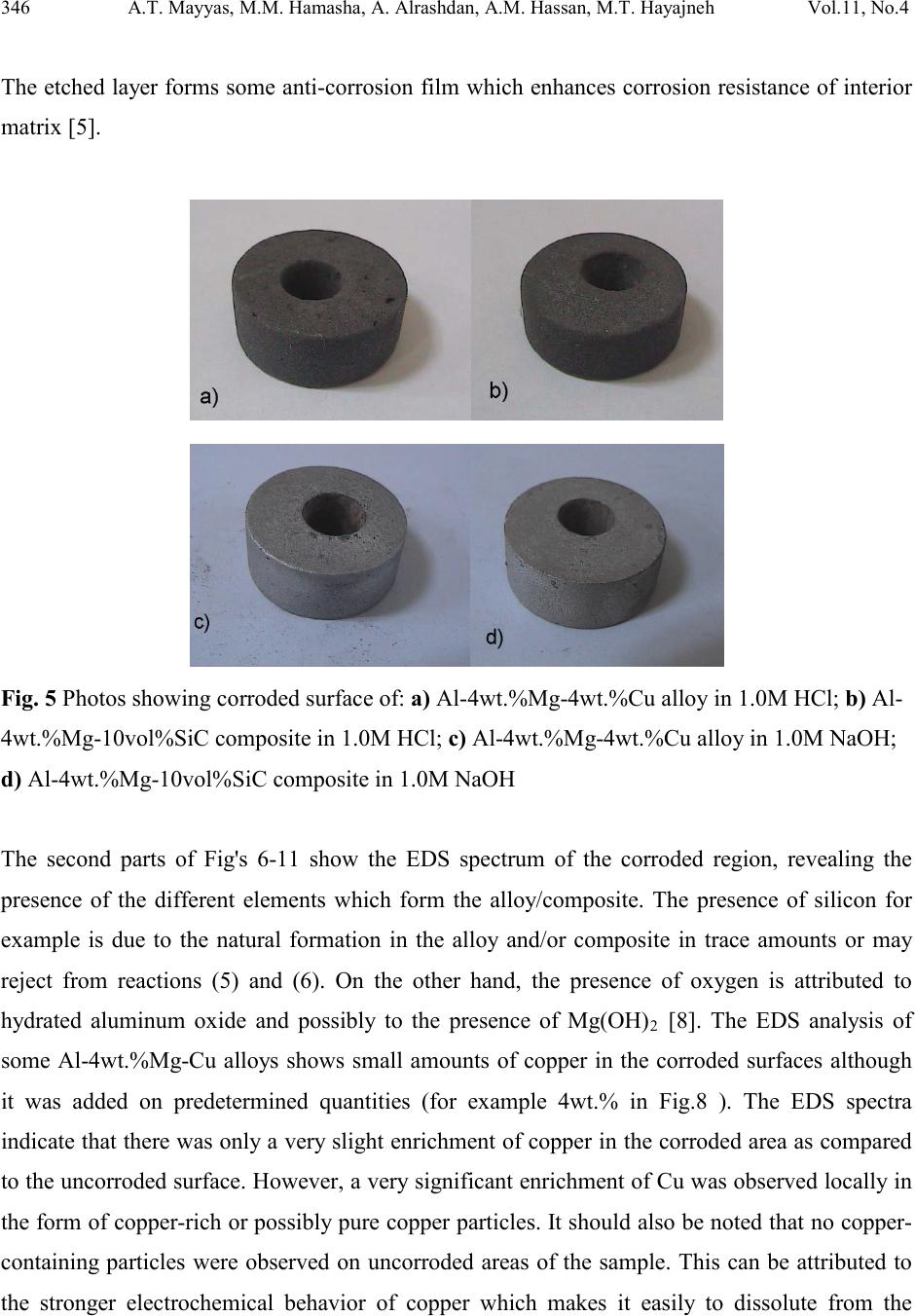 346 A.T. Mayyas, M.M. Hamasha, A. Alra shd a n , A.M. Hassan, M.T. Hayajneh Vol.11, No.4 The etched layer forms some anti-corrosion film which enhances corrosion resistance of interior matrix [5]. Fig . 5 Photos showing corroded surface of: a) Al-4wt.%Mg-4wt.%Cu alloy in 1.0M HCl; b) Al- 4wt.%Mg-10vol%SiC composite in 1.0M HCl; c) Al-4wt.%Mg-4wt.%Cu alloy in 1.0M NaOH; d) Al-4wt.%Mg-10vol%SiC composite in 1.0M NaOH The second parts of Fig's 6-11 show the EDS spectrum of the corroded region, revealing the presence of the different elements which form the alloy/composite. The presence of silicon for example is due to the natural formation in the alloy and/or composite in trace amounts or may reject from reactions (5) and (6). On the other hand, the presence of oxygen is attributed to hydrated aluminum oxide and possibly to the presence of Mg(OH)2 [8]. The EDS analysis of some Al-4wt.%Mg-Cu alloys shows small amounts of copper in the corroded surfaces although it was added on predetermined quantities (for example 4wt.% in Fig.8 ). The EDS spectra indicat e that there was o nly a very slight enrichm ent of copper i n the corroded area as compared to the uncorroded surface. However, a very significant enrichment of Cu was observed locally in the form of copper-rich or possibly pure copper particles. It should also be noted that no copper- containing particles were observed on uncorroded areas of the sample. This can be attributed to the stronger electrochemical behavior of copper which makes it easily to dissolute from the
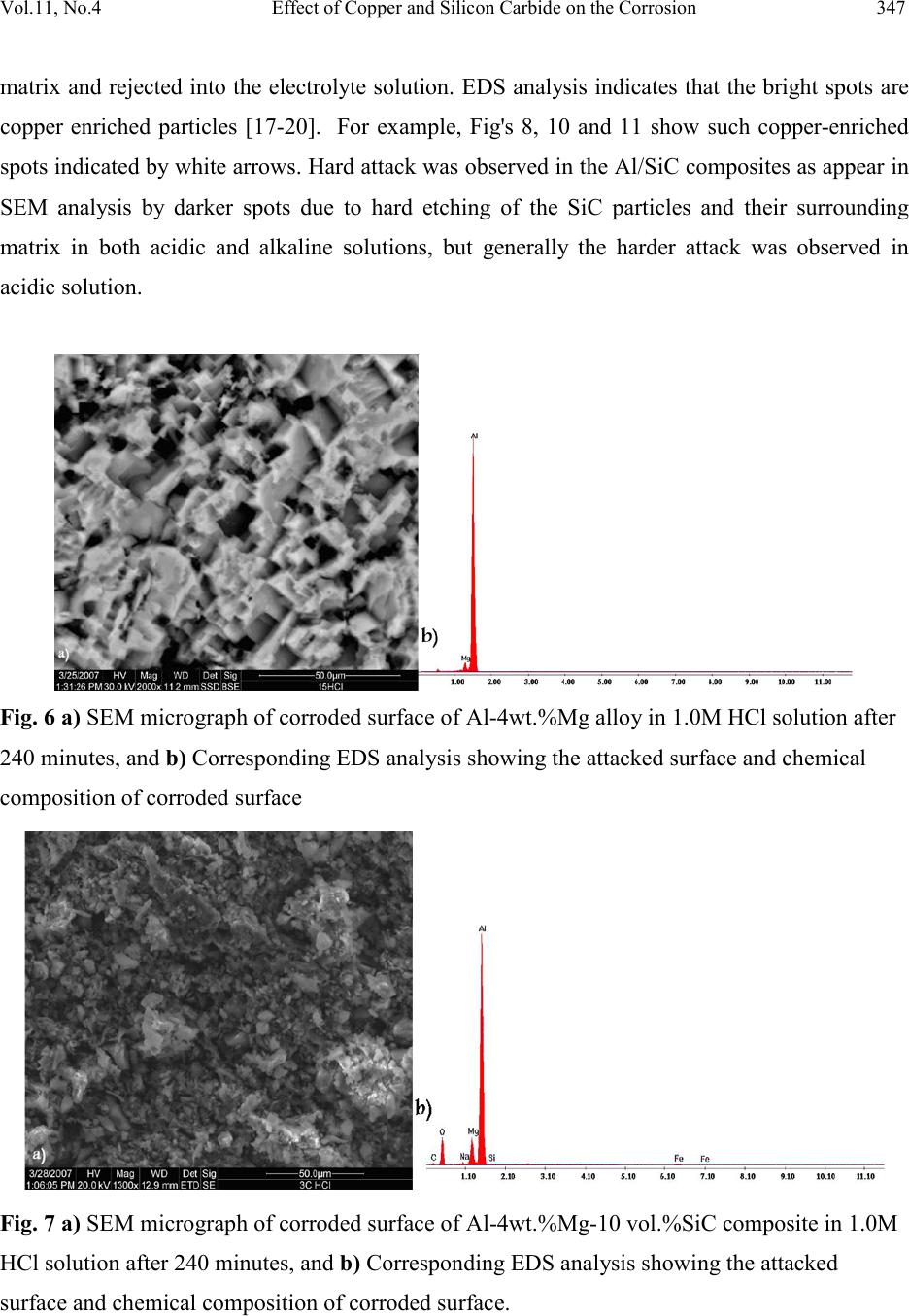 Vol.11, No.4 Effect of Copper and Silicon Carbide o n the Corrosion 347 matrix and rejected into the electrolyte solution. EDS analysis indicates that the bright spots are copper enriched particles [17-20]. For example, Fig's 8, 10 and 11 show such copper-enriched spots indicated by white arrows. Hard attack was observed in the Al/SiC composites as appear in SEM analysis by darker spots due to hard etching of the SiC particles and their surrounding matrix in both acidic and alkaline solutions, but generally the harder attack was observed in acidic solution. Fig. 6 a) SEM micrograph of corroded surface of Al-4wt.%Mg alloy in 1.0M HCl solution after 240 minutes, and b) Corresponding EDS analysis showing the attacked surface and chemical composition of corroded surface Fig. 7 a) SEM micrograph of corroded surface of Al-4wt.%Mg-10 vol.%SiC composite in 1.0M HCl solution after 240 minutes, and b) Corresponding EDS analysis showing the attacked surface and chemical composition of corroded surface.
 348 A.T. Mayyas, M.M. Hamasha, A. Alra shd a n , A.M. Hassan, M.T. Hayajneh Vol.11, No.4 Fig. 8 a) SEM micrograph of corroded surface of Al-4wt.%Mg-4 wt.%Cu alloy in 1.0M HCl solution after 240 minutes, and b) Corresponding EDS analysis showing the attacked surface and chemical composition of corroded surface. Fig. 9 a) SEM micrograph of corroded surface of Al-4wt.%Mg alloy in 1.0M NaOH solution after 240 minutes, and b) Corresponding EDS analysis showing the attacked surface and chemical composition of corroded surface.
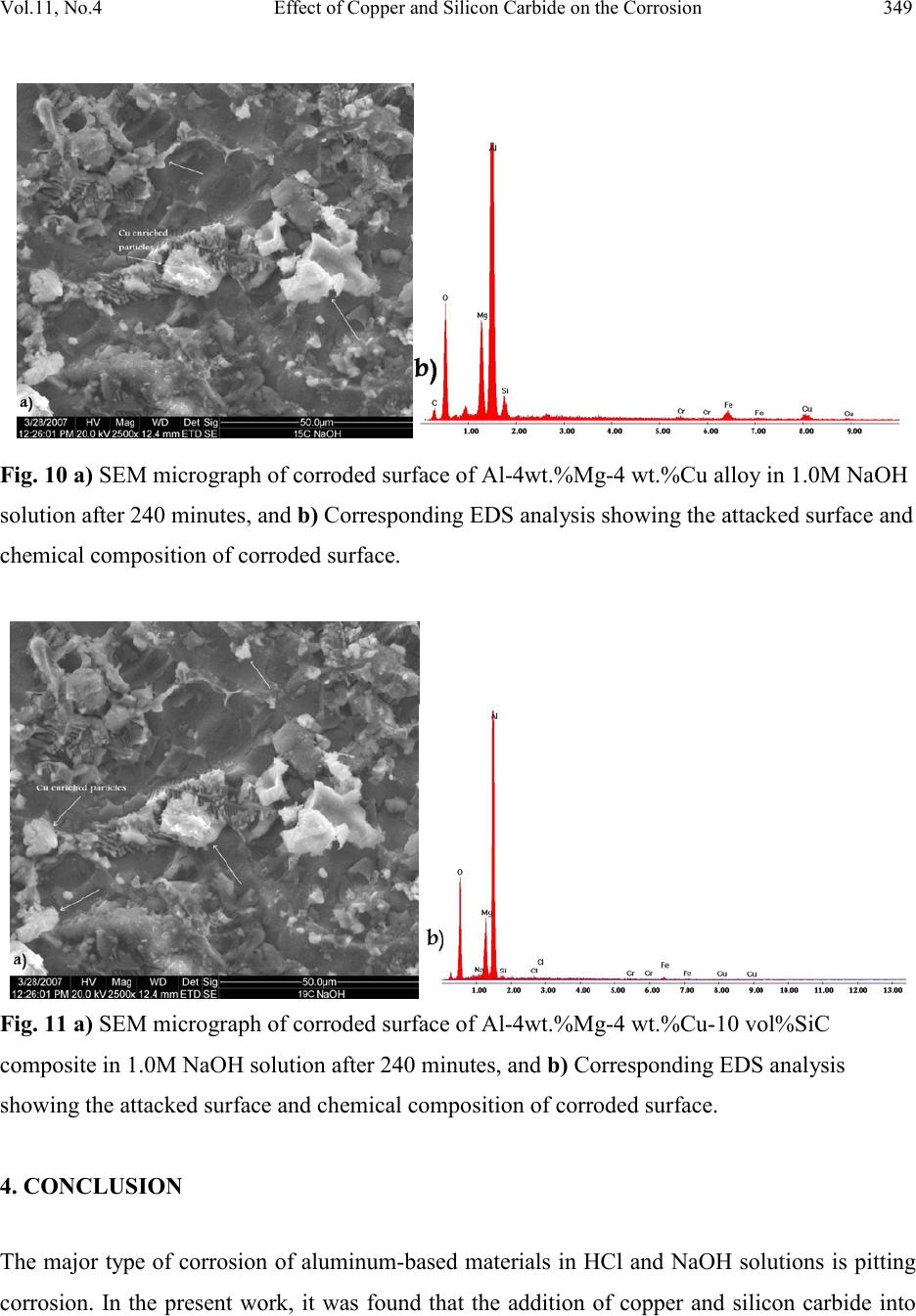 Vol.11, No.4 Effect of Copper and Silicon Carbide o n the Corrosion 349 Fig. 10 a) SEM micrograph of corroded surface of Al-4wt.%Mg-4 wt.%Cu alloy in 1.0M NaOH solution after 240 minutes, and b) Corresponding EDS analysis showing the attacked surface and chemical composition of corroded surface. Fig. 11 a) SEM micrograph of corroded surface of Al-4wt.%Mg-4 wt.%Cu-10 vol%SiC composite in 1.0M NaOH solution after 240 minutes, and b) Corresponding EDS analysis showing the attacked surface and chemical composition of corroded surface. 4. CONCLUSIO N The major type of corrosion of aluminum-based materials in HCl and NaOH solutions is pitting corrosion. In the present work, it was found that the addition of copper and silicon carbide into
 350 A.T. Mayyas, M.M. Hamasha, A. Alra shd a n , A.M. Hassan, M.T. Hayajneh Vol.11, No.4 Al-4 wt.%Mg alloy tend to diminish the ex cellent corrosion resistance of pure aluminum in both acidic and alkaline media. The addition of SiC particles will also diminish the corrosion resistance of Al-4wt.%Mg alloy since that it is difficult to achieve very good bond-ability between ceramic and metal. On the other hand, during corrosion tests in extremely alkaline or acid solutions, Cu surface enrichment likely occurs by particle dissolution and de-alloying and possible de-alloying of the matrix phase. It was found that both Al-based alloys and their associated composites have fewer tendencies to corrode in NaOH compared to HCl environment. SEM analysis shows that the corrosion effect on the silicon carbide containing composites is more sever compared to corresponding Al-4wt.%Mg-Cu alloys. When conducting EDS analysis the absence of copper on the corroded surfaces is attributed to the high chemical activity of copper eit her by formation of the hard intermetallic particles which are usually very active or by dissolution in corrodent. ACKNOWLEDGEMENTS The authors gratefully acknowledge the assis tance of t he commit tee of scien tific r esearch/ J ord an University of Science and Technology for its support of this research (grant No. 29/2007). The authors would like also to gratefully acknowledge the use of Machine shop and the laboratory facilities at Jordan University of Science and Technology, Irbid, Jordan REFERENCES 1. Ahlatci H, Kocer T, Candan E, Cimenoglu H. Wear behaviour of Al/(AlR2ROR3PR +SiCRPR) hybrid composites. Tribology international 2006; 39: 213-220. 2. Hassan AM, Tashtoush GM, Alkhalil JA. The effect of graphite and/or silicon carbide addition on the hardness and surface roughness of Al- 4 wt.%Mg alloy. Composite materials 2007; 41(4): 453-465. 3. Gouveia-Caridade C, Pereira MIS. Brett CMA. Electrochemical noise and impedance study of aluminum in weakly acid chloride solution. Electrochimica Acta 2004; 49: 785– 793. 4. Kiourtsidis GE, Skolianos SM. Pitting corrosion of artificially aged T6 AA2024/SiCp
 Vol.11, No.4 Effect of Copper and Silicon Carbide o n the Corrosion 351 composites in 3.5wt.% NaCl aqueous solution. Corrosion Science. Corrosion Science 2007; 49: 2711–2725. 5. Szklarska-Smialowska Z. Pitting corrosion of aluminum. Corrosion Science 1999; 41 : 1743-1767. 6. Rushing JC, Edwards M. Effect of aluminum solids and chlorine on cold water pitting of copper. Corrosion Science 2004; 46: 3069–3088. 7. Escalera-Lozano R, Gutiérrez CA, Pech-Canul MA, Pech-Canul MI. Corrosion characteristics of hybrid Al/SiCp/MgAl2O4 composites fabricated with fly ash and recycled aluminum. Materials Characterization 2006; 58(10): 953-960. 8. Ahmad Z, Abdul Aleem BJ. Degradation of aluminum metal matrix composites in salt water and its control. Materials and Design 2002; 23: 173-180 9. Ramachandra M, Radhakrishna K. Sliding wear, slurry erosive wear, and corrosive wear of aluminum/SiC composite. Materials science- Poland 2006; 24 (2/1):334-349. 10. Candan S. and Bilgic E. Corrosion behavior of Al-60vol.%SiCP composites in NaCl, Materials letters 2004; 58: 2787-2790. 11. Sennur Candan. An investigation on corrosion behaviour of pressure infiltrated Al–Mg alloy/SiCp composites. Corrosion Science 2009, 5 1 (6): 1392-1398. 12. Sennur Candan. Effect of SiC particle size on corrosion behavior of pressure infiltrated Al matrix composites in a NaCl solution. Materials Letters 58 (2004) 3601 – 3605. 13. Abiola OK, Oforka NC, Angaye SS. Corrosion behavior of aluminum in hydrochloric acid (HCl) solution containing mercaptoacetic acid. Materials Letters 2004; 58: 3461–3466 14. Oguzie EE, Okolue BN, Ebenso EE, Onuoha GN, Onuchukwu AI. Evaluation of the inhibitory effect of methylene blue dye on the corrosion of aluminum in hydrochloric acid. Material s C hemis try and Physics 2004; 87:394–401. 15. Lunarska E, Chernyayeva O. Effect of corrosion inhibitors on hydrogen uptake by Al from NaOH solution. International Journal of Hydrogen Energy 2006; 31: 285 – 293. 16. Liu Z, Chong PH, Butt AN, Skeldon P, Thompson GE. Corrosion mechanism of laser- melted AA 2014 and AA 2024 alloys. Applied Surface Science 2005; 247: 294-299. 17. Blanc C, Freulon A, Lafont MC, Kihn Y, Mankowski G. Modelling the corrosion behavior of Al 2C uMg coarse particl es in copper-rich aluminum alloys. Corrosion Science 2006; 48: 3838–3851.
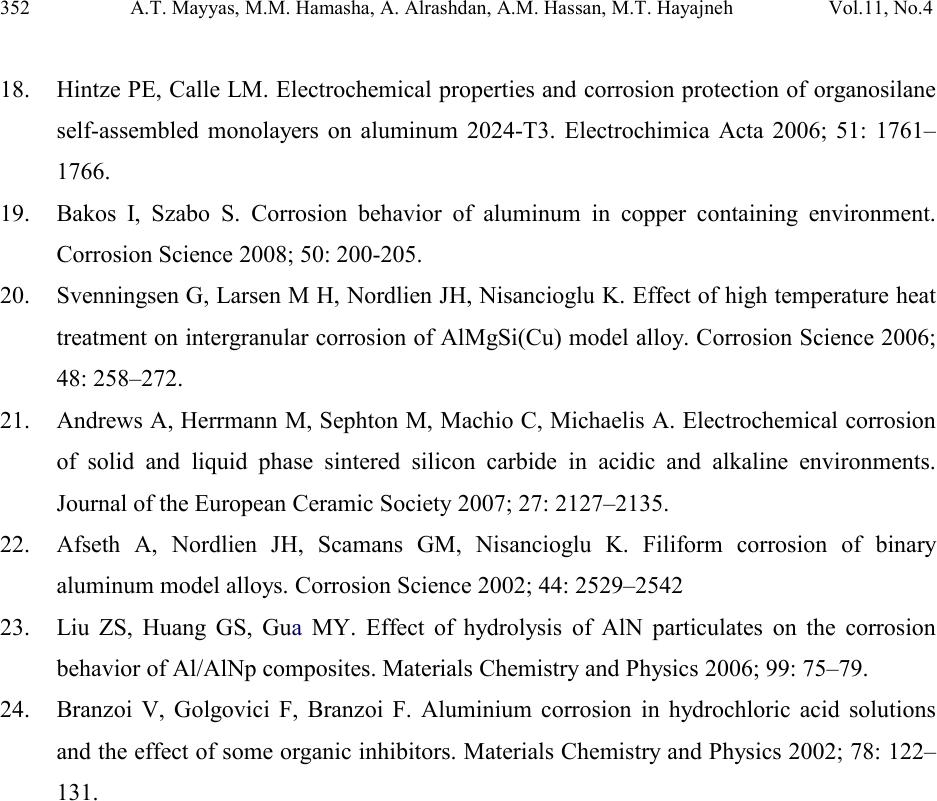 352 A.T. Mayyas, M.M. Hamasha, A. Alra shd a n , A.M. Hassan, M.T. Hayajneh Vol.11, No.4 18. Hintze PE, Calle LM. Electrochemical properties and corrosion protection of organosilane self-assembled monolayers on aluminum 2024-T3. Electrochimica Acta 2006; 51: 1761– 1766. 19. Bakos I, Szabo S. Corrosion behavior of aluminum in copper containing environment. Corrosion Science 2008; 50: 200-205. 20. Svenningsen G, Larsen M H, Nordlien JH, Nisancioglu K. Effect of high temperature heat treatment on intergranular corrosion of AlMgSi(Cu) model alloy. Corrosion Science 2006; 48: 258–272. 21. Andrews A, Herrmann M, Sephton M, Machio C, Michaelis A. Electrochemical corrosion of solid and liquid phase sintered silicon carbide in acidic and alkaline environments. Journal of the European Ceramic Society 2007; 27: 2127–2135. 22. Afseth A, Nordlien JH, Scamans GM, Nisancioglu K. Filiform corrosion of binary aluminum model alloys. Corrosion Science 2002; 44: 2529–2542 23. Liu ZS, Huang GS, Gua MY. Effect of hydrolysis of AlN particulates on the corrosion behavior of Al/AlNp composites. Material s C hem istry and Physics 2006; 99: 75–79. 24. Branzoi V, Golgovici F, Branzoi F. Aluminium corrosion in hydrochloric acid solutions and the effect of some organic inhibitors. Materials Chemistry and Physics 2002; 78: 122– 131.
|