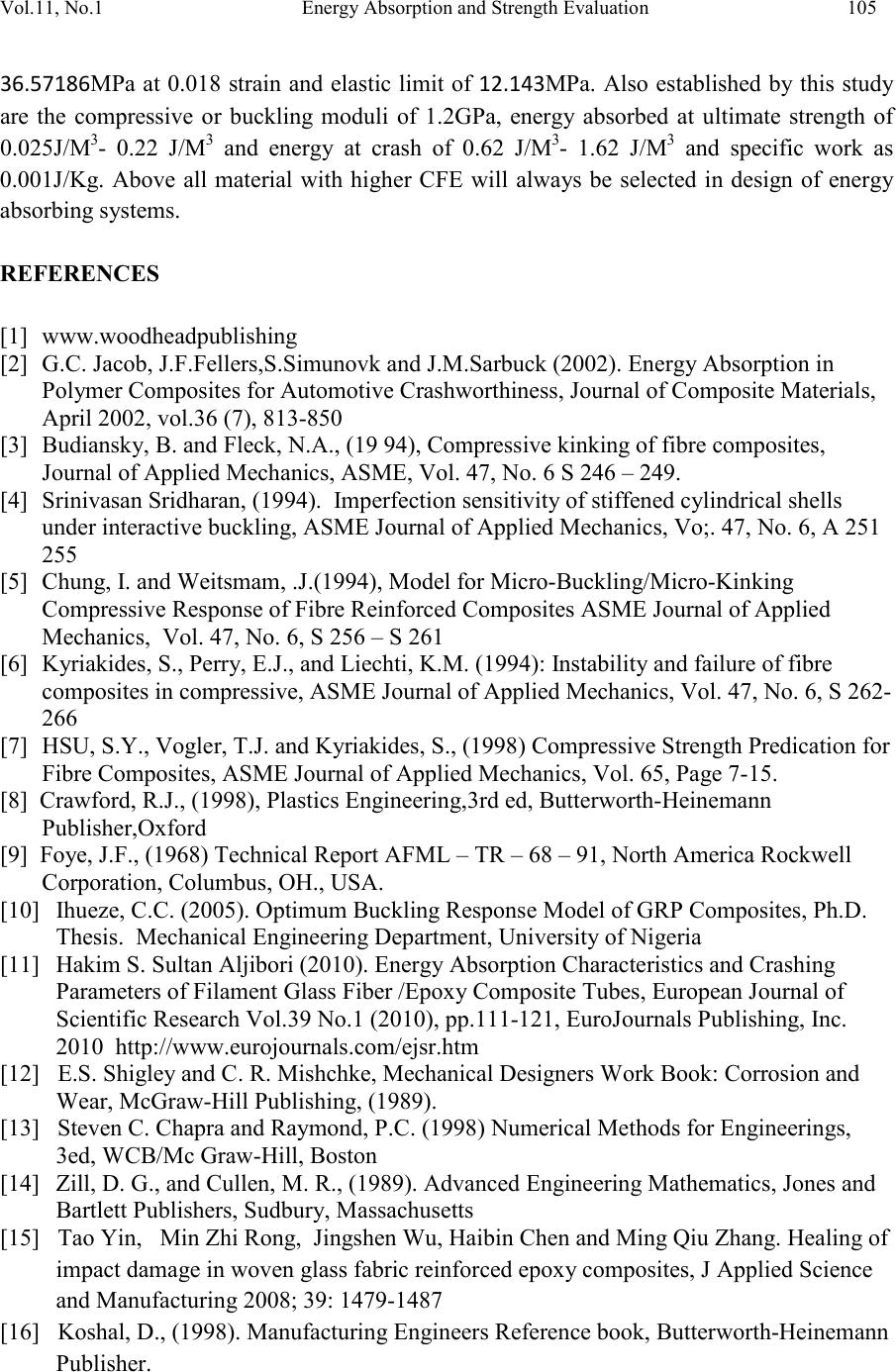
Vol.11, No.1 Energy Abs orption and S trength Evaluati on 105
36.57186MPa at 0.018 strain and elastic limit of 12.143MPa. Also established by this study
are the compressive or buckling moduli of 1.2GPa, energy absorbed at ultimate strength of
0.025J/M3- 0.22 J/M3 and energy at crash of 0.62 J/M3- 1.62 J/M3 and specific work as
0.001J/Kg. Above all material with higher CFE will always be selected in design of energy
absorbing systems.
REFERENCES
[1] www.woodheadpublishing
[2] G.C. Jacob, J.F.Fellers,S.Simunovk and J.M.Sarbuck (2002). Energy Absorption in
Polymer Composites for Automotive Crashworthiness, Journal of Composite Materials,
April 2002, vol.36 (7), 813-850
[3] Budiansky, B. and Fleck, N.A., (19 94), Compressive kinking of fibre composites,
Journal of Applied Mechanics, ASME, Vol. 47, No. 6 S 246 – 249.
[4] Srinivasan Sridharan, (1994). Imperfection sensitivity of stiffened cylindrical shells
under interactive buckling, ASME Journal of Applied Mechanics, Vo;. 47, No. 6, A 251
255
[5] Chung, I. and Weitsmam, .J.(1994), Model for Micro-Buckling/Micro-Kinking
Compressive Response of Fibre Reinforced Composites ASME Journal of Applied
Mechanics, Vol. 47, No. 6, S 256 – S 261
[6] Kyriakides, S., Perry, E.J., and Liechti, K.M. (1994): Instability and failure of fibre
composites in compressive, ASME Journal of Applied Mechanics, Vol. 47, No. 6, S 262-
266
[7] HSU, S.Y., Vogler, T.J. and Kyriakides, S., (1998) Compressive Strength Predication for
Fibre Composites, ASME Journal of Applied Mechanics, Vol. 65, Page 7-15.
[8] Crawford, R.J., (1998), Plastics Engineering,3rd ed, Butterworth-Heinemann
Publisher,Oxford
[9] Foye, J.F., (1968) Technical Report AFML – TR – 68 – 91, North America Rockwell
Corporation, Columbus, OH., USA.
[10] Ihueze, C.C. (2005). Optimum Buckling Response Model of GRP Composites, Ph.D.
Thesis. Mechanical Engineering Department, University of Nigeria
[11] Hakim S. Sultan Aljibori (2010). Energy Absorption Characteristics and Crashing
Parameters of Filament Glass Fiber /Epoxy Composite Tubes, European Journal of
Scientific Research Vol.39 No.1 (2010), pp.111-121, EuroJournals Publishing, Inc.
2010 http://www.eurojournals.com/ejsr.htm
[12] E.S. Shigley and C. R. Mishchke, Mechanical Designers Work Book: Corrosion and
Wear, McGraw-Hill Publishing, (1989).
[13] Steven C. Chapra and Raymond, P.C. (1998) Numerical Methods for Engineerings,
3ed, WCB/Mc Graw-Hill, Boston
[14] Zill, D. G., and Cullen, M. R., (1989). Advanced Engineering Mathematics, Jones and
Bartlett Publishers, Sudbury, Massachusetts
[15] Tao Yin, Min Zhi Rong, Jingshen Wu, Haibin Chen and Ming Qiu Zhang. Healing of
impact damage in woven glass fabric reinforced epoxy composites, J Applied Science
and Manufacturing 2008; 39: 1479-1487
[16] Koshal, D., (1998). Manufacturing Engineers Reference book, Butterworth-Heinemann
Publisher.