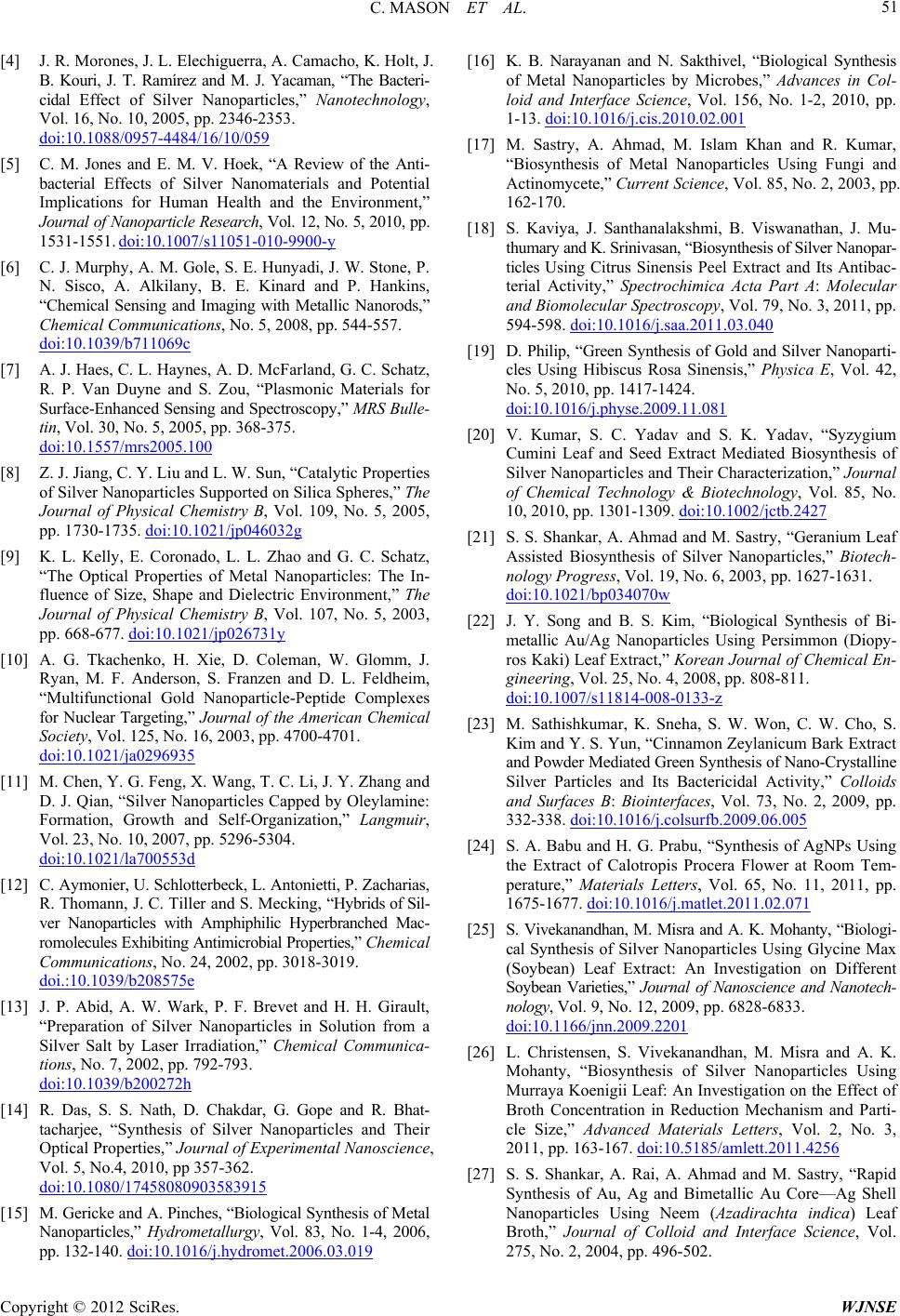
C. MASON ET AL. 51
[4] J. R. Morones, J. L. Elechiguerra, A. Camacho, K. Holt, J.
B. Kouri, J. T. Ramírez and M. J. Yacaman, “The Bacteri-
cidal Effect of Silver Nanoparticles,” Nanotechnology,
Vol. 16, No. 10, 2005, pp. 2346-2353.
doi:10.1088/0957-4484/16/10/059
[5] C. M. Jones and E. M. V. Hoek, “A Review of the Anti-
bacterial Effects of Silver Nanomaterials and Potential
Implications for Human Health and the Environment,”
Journal of Nanoparticle Research, Vol. 12, No. 5, 2010, pp.
1531-1551. doi:10.1007/s11051-010-9900-y
[6] C. J. Murphy, A. M. Gole, S. E. Huny adi, J. W. Stone, P.
N. Sisco, A. Alkilany, B. E. Kinard and P. Hankins,
“Chemical Sensing and Imaging with Metallic Nanorods,”
Chemical Communications, No. 5, 2008, pp. 544-557.
doi:10.1039/b711069c
[7] A. J. Haes, C. L. Hay nes, A. D. McFarland, G. C. Schatz,
R. P. Van Duyne and S. Zou, “Plasmonic Materials for
Surface-Enhanced Sensing and Spectroscopy,” MRS Bulle-
tin, Vol. 30, No. 5, 2005, pp. 368-375.
doi:10.1557/mrs2005.100
[8] Z. J. Jiang, C. Y. Liu and L. W. Sun, “Catalytic Properties
of Silver Nanoparticles Supported on Silica Spheres,” The
Journal of Physical Chemistry B, Vol. 109, No. 5, 2005,
pp. 1730-1735. doi:10.1021/jp046032g
[9] K. L. Kelly, E. Coronado, L. L. Zhao and G. C. Schatz,
“The Optical Properties of Metal Nanoparticles: The In-
fluence of Size, Shape and Dielectric Environment,” The
Journal of Physical Chemistry B, Vol. 107, No. 5, 2003,
pp. 668-677. doi:10.1021/jp026731y
[10] A. G. Tkachenko, H. Xie, D. Coleman, W. Glomm, J.
Ryan, M. F. Anderson, S. Franzen and D. L. Feldheim,
“Multifunctional Gold Nanoparticle-Peptide Complexes
for Nuclear Targeting,” Journal of the American Chemical
Society, Vol. 125, No. 16, 2003, pp. 4700-4701.
doi:10.1021/ja0296935
[11] M. Chen, Y. G. Feng, X. Wang, T. C. Li, J. Y. Zhang and
D. J. Qian, “Silver Nanoparticles Capped by Oleylamine:
Formation, Growth and Self-Organization,” Langmuir,
Vol. 23, No. 10, 2007, pp. 5296-5304.
doi:10.1021/la700553d
[12] C. Aymonier, U. Schlotterbeck, L. Antonietti, P. Zacharias,
R. Thomann, J. C. Tiller and S. Mecking, “Hybrids of Sil-
ver Nanoparticles with Amphiphilic Hyperbranched Mac-
romolecules Exhibiting Antimicrobial Properties,” Chemical
Communications, No. 24, 2002, pp. 3018-3019.
doi.:10.1039/b208575e
[13] J. P. Abid, A. W. Wark, P. F. Brevet and H. H. Girault,
“Preparation of Silver Nanoparticles in Solution from a
Silver Salt by Laser Irradiation,” Chemical Communica-
tions, No. 7, 2002, pp. 792-793.
doi:10.1039/b200272h
[14] R. Das, S. S. Nath, D. Chakdar, G. Gope and R. Bhat-
tacharjee, “Synthesis of Silver Nanoparticles and Their
Optical Properties,” Journal of Experimental Nanoscience,
Vol. 5, No.4, 2010, pp 357-362.
doi:10.1080/17458080903583915
[15] M. Gericke and A. Pinches, “Biological Synthesis of Metal
Nanoparticles,” Hydrometallurgy, Vol. 83, No. 1-4, 2006,
pp. 132-140. doi:10.1016/j.hydromet.2006.03.019
[16] K. B. Narayanan and N. Sakthivel, “Biological Synthesis
of Metal Nanoparticles by Microbes,” Advances in Col-
loid and Interface Science, Vol. 156, No. 1-2, 2010, pp.
1-13. doi:10.1016/j.cis.2010.02.001
[17] M. Sastry, A. Ahmad, M. Islam Khan and R. Kumar,
“Biosynthesis of Metal Nanoparticles Using Fungi and
Actinomycete,” Current Science, Vol. 85, No. 2, 2003, pp.
162-170.
[18] S. Kaviya, J. Santhanalakshmi, B. Viswanathan, J. Mu-
thumary and K. Srinivasan, “Biosynthesis of Silver Nanopar-
ticles Using Citrus Sinensis Peel Extract and Its Antibac-
terial Activity,” Spectrochimica Acta Part A: Molecular
and Biomolecular Spectroscopy, Vol. 79, No. 3, 2011, pp.
594-598. doi:10.1016/j.saa.2011.03.040
[19] D. Philip, “Green Synthesis of Gold and Silver Nanoparti-
cles Using Hibiscus Rosa Sinensis,” Physica E, Vol. 42,
No. 5, 2010, pp. 1417-1424.
doi:10.1016/j.physe.2009.11.081
[20] V. Kumar, S. C. Yadav and S. K. Yadav, “Syzygium
Cumini Leaf and Seed Extract Mediated Biosynthesis of
Silver Nanoparticles and Their Characterization,” Journal
of Chemical Technology & Biotechnology, Vol. 85, No.
10, 2010, pp. 1301-1309. doi:10.1002/jctb.2427
[21] S. S. Shankar, A. Ahmad and M. Sastry, “Geranium Leaf
Assisted Biosynthesis of Silver Nanoparticles,” Biotech-
nology Progress, Vol. 19, No. 6, 2003, pp. 1627-1631.
doi:10.1021/bp034070w
[22] J. Y. Song and B. S. Kim, “Biological Synthesis of Bi-
metallic Au/Ag Nanoparticles Using Persimmon (Diopy-
ros Kaki) Leaf Extract,” Korean Journal of Chemical En-
gineering, Vol. 25, No. 4, 2008, pp. 808-811.
doi:10.1007/s11814-008-0133-z
[23] M. Sathishkumar, K. Sneha, S. W. Won, C. W. Cho, S.
Kim and Y. S. Yun, “Cinnamon Zeylanicum Bark Extract
and Powder Mediated Green Synthesi s of Nano-Cry stalline
Silver Particles and Its Bactericidal Activity,” Colloids
and Surfaces B: Biointerfaces, Vol. 73, No. 2, 2009, pp.
332-338. doi:10.1016/j.colsurfb.2009.06.005
[24] S. A. Babu and H. G. Prabu, “Synthesis of AgNPs Using
the Extract of Calotropis Procera Flower at Room Tem-
perature,” Materials Letters, Vol. 65, No. 11, 2011, pp.
1675-1677. doi:10.1016/j.matlet.2011.02.071
[25] S. Vivekanandhan, M. Misra and A. K. Mohanty, “Biologi-
cal Synthesis of Silver Nanoparticles Using Glycine Max
(Soybean) Leaf Extract: An Investigation on Different
Soybean Varieties,” Journal of Nanoscience and Nanotech-
nology, Vol. 9, No. 12, 2009, pp. 6828-6833.
doi:10.1166/jnn.2009.2201
[26] L. Christensen, S. Vivekanandhan, M. Misra and A. K.
Mohanty, “Biosynthesis of Silver Nanoparticles Using
Murraya Koenigii Leaf: An Investigation on the Effect of
Broth Concentration in Reduction Mechanism and Parti-
cle Size,” Advanced Materials Letters, Vol. 2, No. 3,
2011, pp. 163-167. doi:10.5185/amlett.2011.4256
[27] S. S. Shankar, A. Rai, A. Ahmad and M. Sastry, “Rapid
Synthesis of Au, Ag and Bimetallic Au Core—Ag Shell
Nanoparticles Using Neem (Azadirachta indica) Leaf
Broth,” Journal of Colloid and Interface Science, Vol.
275, No. 2, 2004, pp. 496-502.
Copyright © 2012 SciRes. WJNSE