 Journal of Behavioral and Brain Science, 2012, 2, 156-161 http://dx.doi.org/10.4236/jbbs.2012.22018 Published Online May 2012 (http://www.SciRP.org/journal/jbbs) Dissociation between Performances in Water Maze and Spontaneous Alternation in BALB/c versus A/J Mice Julien Celestine1*, Arnaud Tanti2, Arnaud Aubert3 1INSERM U930 E2 Neurogénétique et Neurométabolomique CHRU de Tours, Hôpital Bretonneau, 2 Boulevard Tonnellé, Bât B1A, 1er Etage, 37044 Tours Cedex 9, France 2Faculté des Sciences et Techniques, Université François Rabelais, Tours, France 3EA 2114, Psychologie des Ages de la Vie, Université de Tours, 3 Rue des Tanneurs , 37041 Tours Cedex 1, France Email: *julien.celestine@etu.univ-tours.fr Received March 7, 2011; revised April 15, 2011; accepted May 7, 2011 ABSTRACT Learning processes are extensively studied in behavioral neuroscience. As experimental models, Morris Water Maze (MWM) and Spontaneous Alternation (SA) represent two of the most frequently used laboratory tests to respectively address spatial vs non-spatial tasks. Several factors have been shown to impact on those learning, including strain, gen- der, apparatus, conditioning, vision, lighting conditions and stress level. In order to focus on the later, we compared the acquisition of two learning tasks (MWM and SA) in BALB/c and A/J mice, which are known as fearful and stress-sensitive strains. Here, we report that BALB/c mice exhibited higher performances than A/J mice in the MWM (i.e. spatial reference memory task), whereas A/J mice performed better in the SA (i.e. spatial working memory task). These results indicate dissociated processes in the acquisition of spatial vs non-spatial tasks, and emphasize a varying influence of emotional reactivity on different forms of cognition. Keywords: Morris Water Maze; Spontaneous Alternation; Behavior; BALB/c; A/J; Learning 1. Introduction Emotions are co mmonly descr ibed as the processes wh ere- by brain could evaluate stimuli, basically as pleasant or unpleasant [1-3], promoting either approach or avoidance behaviors, thus supporting individual adaptation and sur- vival. Such a key role in behavioral guidance and deci- sion making is growingly been emphasized [1,2], and it emotions are now commonly regarded as adaptive proc- esses signaling relevant cues about environmental changes. However, assessment of emotions remains a difficult task [4], and especially in non-human animals where verbal information is unavailable. Nevertheless, human and non- human darwinian emotions share many biobehavioral features [5]. This evolutionary common ground allows the investigation of emotional state by using animal models [4]. It has been argued that emotions represent a warning system and a way to optimize action [6]. That is the rea- son why animals could exhibit very different behaviors under normal or stressful conditions. Throughout the evolution of species, some behavioral mechanisms were selected on the basis of their significant survival benefit. Such behaviors like fear-induced freezing or fleeing are considered are widespread evolutionary stable strategies [7,8]. The selection of the final response (i.e. freezing or fleeing) is largely dependent on actual emotional arousal triggered by environmental cues. Emotions act as potent factors for rapid adaptive decision making processes. Therefore, they represent modulatory tools for cognition rather than inhibitory factors. Advances in psychology and neurosciences have also shown that core cognitive functio ns such as learning and memory share many complex interactions with emotional processes. For example, patients suffering from various mood disorders also express memory impairments, and corresponding animal models were developed [4,5] to better understand the mechanisms underlying these rela- tions. Among the diverse experimental tests designed to assess learning and memory in animals, two main cate- gories of tests consist in reference versus working mem- ory tasks [9,10], especially in rodent studies [11-16]. Morris Water Maze (MWM) [17] and Spontaneous Al- ternation (SA) [18] for example became standard tasks to explore such functions. Indeed, they are versatile and allow many variations to assess specific processes and/or factors (e.g. pharmacological agents, gen etic background and neurological preparation). While those behavioral models were generally design e d for rats, mice are nowadays the main animal model in *Corresponding a uthor. C opyright © 2012 SciRes. JBBS
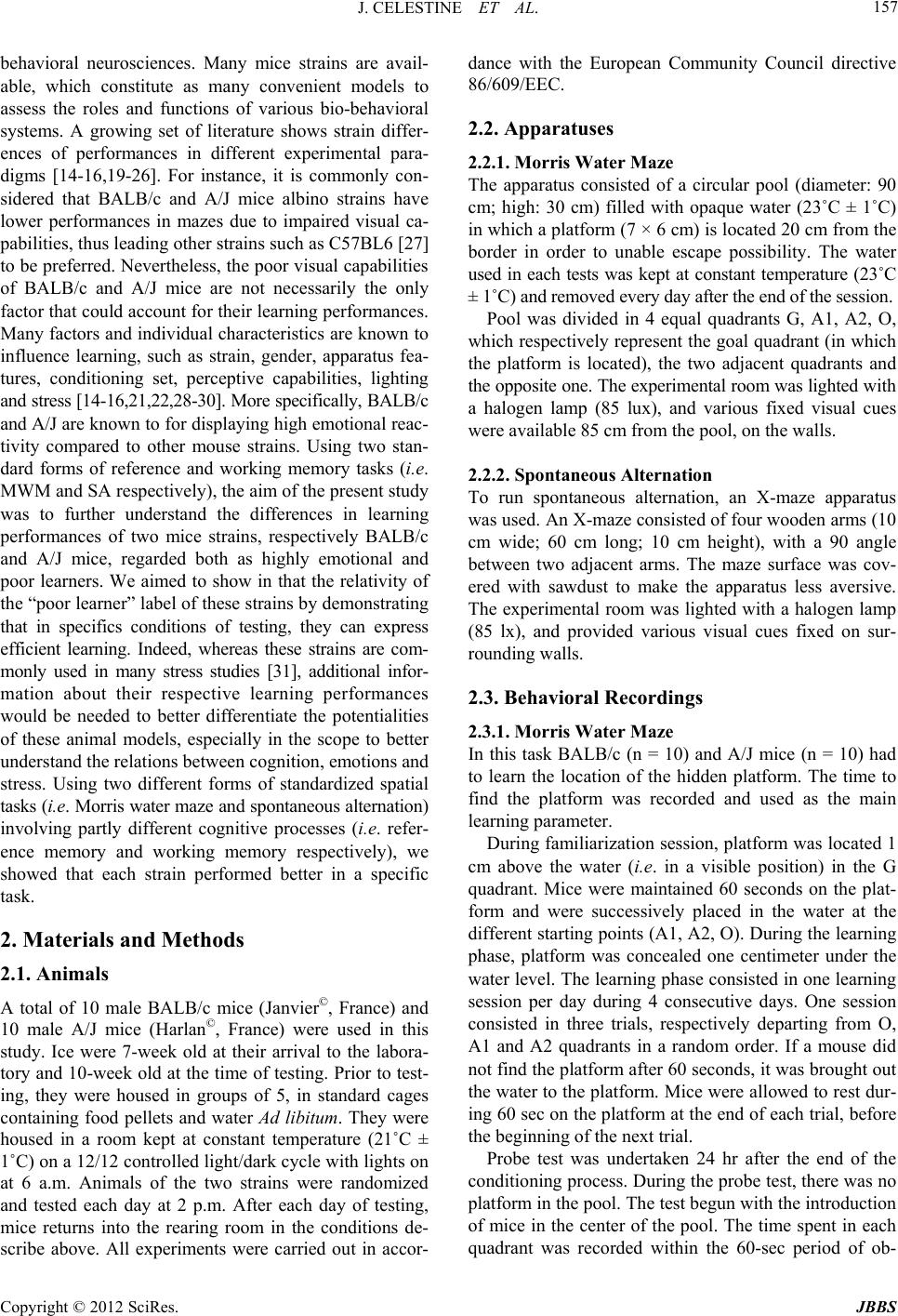 J. CELESTINE ET AL. 157 behavioral neurosciences. Many mice strains are avail- able, which constitute as many convenient models to assess the roles and functions of various bio-behavioral systems. A growing set of literature shows strain differ- ences of performances in different experimental para- digms [14-16,19-26]. For instance, it is commonly con- sidered that BALB/c and A/J mice albino strains have lower performances in mazes due to impaired visual ca- pabilities, thus leadin g oth er strains su ch as C57 BL6 [27 ] to be preferred. Nevertheless, th e poor visual cap abilities of BALB/c and A/J mice are not necessarily the only factor that could account for their learning performances. Many factors and individual characteristics are known to influence learning, such as strain, gender, apparatus fea- tures, conditioning set, perceptive capabilities, lighting and stress [14-16,21,22,28-30]. More specifically, BALB/c and A/J are known to for displaying high emotional reac- tivity compared to other mouse strains. Using two stan- dard forms of reference and working memory tasks (i.e. MWM and SA respectively), the aim of the present study was to further understand the differences in learning performances of two mice strains, respectively BALB/c and A/J mice, regarded both as highly emotional and poor learners. We aimed to show in that the relativity of the “poor learner” label of these strains by demonstrating that in specifics conditions of testing, they can express efficient learning. Indeed, whereas these strains are com- monly used in many stress studies [31], additional infor- mation about their respective learning performances would be needed to better differentiate the potentialities of these animal models, especially in the scope to better understand the relations between cognition, emotions and stress. Using two different forms of standardized spatial tasks (i.e. Morris water maze and spontaneous alternation) involving partly different cognitive processes (i.e. refer- ence memory and working memory respectively), we showed that each strain performed better in a specific task. 2. Materials and Methods 2.1. Animals A total of 10 male BALB/c mice (Janvier©, France) and 10 male A/J mice (Harlan©, France) were used in this study. Ice were 7-week old at their arrival to the labora- tory and 10-week old at the ti me of testing. Prior to test- ing, they were housed in groups of 5, in standard cages containing food pellets and water Ad libitum. They were housed in a room kept at constant temperature (21˚C ± 1˚C) on a 12/12 controlled light/dark cycle with lights on at 6 a.m. Animals of the two strains were randomized and tested each day at 2 p.m. After each day of testing, mice returns into the rearing room in the conditions de- scribe above. All experiments were carried out in accor- dance with the European Community Council directive 86/609/EEC. 2.2. Apparatuses 2.2.1. Morris Water M a ze The apparatus consisted of a circular pool (diameter: 90 cm; high: 30 cm) filled with opaque water (23˚C ± 1˚C) in which a platform (7 × 6 cm) is located 20 cm from the border in order to unable escape possibility. The water used in each tests was kept at constant temperature (23˚C ± 1˚C) and removed every day aft er the end of the session. Pool was divided in 4 equal quadrants G, A1, A2, O, which respectively represent the goal quadrant (in which the platform is located), the two adjacent quadrants and the opposite one. The experimental room was lighted with a halogen lamp (85 lux), and various fixed visual cues were available 85 cm from the pool, on the walls. 2.2.2. Spontaneous Alternation To run spontaneous alternation, an X-maze apparatus was used. An X-maze consisted of four wooden arms (10 cm wide; 60 cm long; 10 cm height), with a 90 angle between two adjacent arms. The maze surface was cov- ered with sawdust to make the apparatus less aversive. The experimental room was lighted with a halogen lamp (85 lx), and provided various visual cues fixed on sur- rounding walls. 2.3. Behavioral Recordings 2.3.1. Morris Water M a ze In this task BALB/c (n = 10) and A/J mice (n = 10) had to learn the location of the hidden platform. The time to find the platform was recorded and used as the main learning parame t er. During familiarization session, platform was located 1 cm above the water (i.e. in a visible position) in the G quadrant. Mice were maintained 60 seconds on the plat- form and were successively placed in the water at the different starting points (A1, A2, O). During the learning phase, platform was concealed one centimeter under the water level. The learning phase consisted in one learning session per day during 4 consecutive days. One session consisted in three trials, respectively departing from O, A1 and A2 quadrants in a random order. If a mouse did not find the platform after 60 seconds, it was brough t out the water to the platform. Mice were allowed to rest dur- ing 60 sec on the platform at the end of each trial, before the beginning of the next trial. Probe test was undertaken 24 hr after the end of the conditioning pr ocess. During th e prob e test, there wa s no platform in the pool. The test begun with the in trodu ction of mice in the center of the pool. The time spent in each quadrant was recorded within the 60-sec period of ob- Copyright © 2012 SciRes. JBBS
 J. CELESTINE ET AL. 158 servation. Samples for probe test, samples sizes of each group of mice were decreased to n = 8 due to accidental mortality. 2.3.2. Spontaneous Alternation This paradigm uses the spontaneous tendency of mice to move from one arm of the maze to another. At the begin- ning of the test, BALB/c (n = 8) and A/J (n = 8) mice were placed at the center of the maze and the sequence of entries into the three arms was recorded ov er a period of 10 min, an arm entry being determined as the four paws within that arm. The total number of arm entries was recorded and the spontaneous alternation score was cal- culated as the number of alternations (i.e . entries in three different arms consecutively) divi ded by the total num ber of possible alternations (i.e. total number of arm entries- two) and multiplied by 100. 2.4. Statistical Analyses To allow a valid use of parametric statistical tests, nor- mality and homoscedasticity of data was checked before each test, using Shapiro-Wilk’s and Bartlett’s tests re- spectively. When parametric assumptions were not satis- fied, data were submitted to a Log10 transform to reach the criteria. Learning performances of mice in the MWM were analyzed with a two-way repeated ANOVA (strain × training session). When main analysis showed a sig- nificant effect of one of the main factors, post-hoc pair- wise analyses were done using the Holm-Sidak proce- dure. The differences between strains in the probe test were analyzed with unpaired Student’s t-test. Student’s t-test was also used to analyze spontaneous alternation scores, number of arm entries and alternation percentage in the SA (within and between subjects’ comparisons re- spectively). Comparisons between strains were done using unpaired t-tests while comparisons of performances of each strain with random scores were done using one-sam- ple t-tests. 3. Results 3.1. Morris Water Maze Figure 1 shows the mean latency to reach the hidden plat- form during acquisition sessions in BALB/c (n = 10) and A/J mice (n = 10). A two-way repeated ANOVA showed a significant difference in latency to find the hidden platform between the two strains (F1,54 = 5.365; p = 0.033), revealing a globally shorter latency in BALB/c mice compared to A/J (mean latencies: 24.97 sec vs 35.15 sec respectively). It also showed a significant dif- ference for latency to find the hidden platform between training day (F3,54 = 5.093; p = 0.004). Post-hoc com- parisons showed that latency to find the platform was significantly smaller from session 4 relative to session 1 in BALB/c (Holm-Sidak’ s p = 0.004) but not in A /J mice (Holm-Sidak’s p = 0.178). Moreover, test showed that latency to find the platform was not significantly differ- ent between the two strains on session 1 (Holm-Sidak’s p = 0.382), but was significantly shorter in BALB/c mice for session 4 compared to A/J mice (Holm-Sidak’s p = 0.034). Figure 2 illustrates performances of BALB/c (n = 8) and A/J mice (n = 8) in the probe test. Independent Stu- dent’s t-test showed that BALB/c express significantly longer swimming time in the goal quadrant (G) com- pared to A/J (t = 2.274; df = 14; p = 0.0392). Figure 1. Mean (±SEM) escape latencies (in seconds) on successive sessions. Each point represents the average score over three trials. (a) Indicates significant difference inside A/J group (n = 10); (b), (c) Indicate significant difference inside BALB/c group (n = 10); **: p < 0.01: significant dif- ference between the two strains in session 4. Figure 2. Mean (±SEM) time spent in each quadrant (sec- onds) during probe test. G is the goal quadrant, A1 and A2 are the adjacent quadrants, and O is the opposite quadrant. (a)-(c) Indicate significant difference between quadrant in- side BALB/c group (n = 8); (d) Indicate significant differ- ence between quadrants inside A/J group (n = 8); ***: p < 0.001: significant difference between the two strains in quadrant G and O. Copyright © 2012 SciRes. JBBS
 J. CELESTINE ET AL. 159 3.2. Spontaneous Alternation BALB/c mice (n = 8) showed an alternation rate (mean ± SEM: 54.4% ± 3%) not significantly different from a ran- dom arm entries sequence (one-sample t-test: t = 1.4667; df = 7; p = 0.1859). On the contrary, A/J mice (n = 8) expressed a significantly higher alternation rate (mean ± SEM: 70.8% ± 4.2%) than BALB/c mice (unpaired t-test: t = 3.177; df = 14; p = 0.0067), and significantly differ- ent from a random arm entries sequence (one-sample t-test: t = 4.9524; df = 7; p = 0.0017). Finally, the total number of arm-entries was however higher in BALB/c mice compared to A/J mice (unpaired t-test: t = 5.168; df = 14; p = 0.0001). 4. Discussion In this study, we compared lear ning performances of two strains of mice (BALB/c and A/J) known for their high emotional reactivity and poor learning skills. Each strain was submitted to a spatial memory task (MWM) and a spatial working memory learning task (SA). Results showed that contrary to A/J mice, BALB/c learned the location of the MWM hidden platform in 4 days (Figure 1). Probe test has shown that BALB/c ex- press better abilities to restore the learning information than A/J strain. Moreover, results of spontaneo us alterna- tion test were the opposite, and A/Js’ performances in this test were significantly better than BALB/c. Stress studies involve a limited choice of mice strains (including BALB/c and A/J mice), which are selected on the basis of their sensitivity to stressors [31 ]. In addition, throughout the literature BALB/c and A/J strains are commonly considered as unable or very inefficient in spa- tial task resolution [21]. This work shows that in th e con- trary, these strains are able to efficiently learn a task, but with opposite potentialities, BALB/c performing better than A/J in spatial reference task while A/J mice were better than BALB/c in spatial working task. Since the strains tested in this study are commonly used for their high emotional reactivity, our results can be discussed in terms of relations between emotion and learning [32]. Indeed, our results point out a relation between strain (BALB/c vs A/J), and learning type (spatial vs non-spa- tial). BALB/c and A/J are known to exhibit low lo como- tor activity and high level of emotional reactivity com- pared to other mouse strains [21]. Such an emotional reactivity is generally expressed in mazes as wall hug- ing, floating [5,14,16,19-21,29,33-38] or anxiety-like be- haviors [15,27]. However, supporting the differences showed in our results, few studies reveal that BALB/c have better learning performances than A/J throughout spatial learning [36]. Interestingly, it has been shown that A/J mice exhibit a higher emotional reactivity than BALB/c. It was indirectly reviewed by several authors which showed a differentiation between those two strains for example in the open field test or anxiety like behavior [19,21,37,39,40]. Hence, the less emotionally reactive strain tested in our study (i.e. BALB/c) expressed better performances in the spatial MWM task, thus further sup- porting the known inv erse relation between sensitivity to stress and spatial cognition . As suggested by the germinal works of Donald Hebb [41], performances in many tasks (hence cognitive proc- esses), are a function of the degree of emotional arousal. Indeed, a general rule was described in which emotional arousal influences performances with an inverted U-shape relation, where lowest and highest emotional loads are associated with lowest performances (i.e. poor motiva- tion to solve the task and inhibitory emotional over-load respectively). However, our results support previous sug- gestions that such emotion-cognition relation would not be uni-dimensional, but woul d be ta sk-dependent. In conclusion, our study shows that whereas consid- ered as poor learners, BALB/c and A/J mice can perform efficiently in different learning tasks. More specifically, our results revealed that BALB/c mice performed better than A/J mice in a spatial learning task (MWM) while A/J mice acquired faster a non-spatial task (SA). Consid- ering the fact that BALB/c and less emotionally reactive than A/J mice, this suggests that the influence of emo- tional arousal on cognition (inhibition or facilitation) de- pends on th e natur e of th e task (ref er ence vs work ing me m- ory) and involv ed neural substr ates (e.g. hippocam pus). Indeed MWM and SA as spatial and non-spatial tasks are respectively hippocampus-dependent and non-de- pendent. Conveniently, influence of emotion and stress on hippocampus has been extensively studied [13,32-35, 42,43]. Indeed many studies have investigated and dem- onstrated so far the impairment of hippocampus spatial learning in response to stress procedure such as learned helplessness or chronic mild stress [13,44]. Furthermore, limbic system is largely implicated in emotional response. Particularly the amygdala and also the hippocampus in fear context [45-48]. This link is very consistent with th e behavioral differentiation observed between those two strains. In fact our results are in line with an inverse rela- tionship between hippocampus processing efficiency and HPA axis activity. If conflicting hippocampal activations between emo- tional and spatial p rocesses could constitute a subs tantial hypothesis to understand why a lower emotional arousal would allow better performances in spatial tasks, further studies would be then needed to better understand why high emotional arousal would be beneficial to a dis- criminant task. 5. Acknowledgements Authors thank Catherine Belzung for providing logistic support (i.e. animals and experimental facilities). J. Céles- tine and A. Tanti designed the experimental protocol and Copyright © 2012 SciRes. JBBS
 J. CELESTINE ET AL. 160 collected data. J. Célestine and A. Aubert undertook sta- tistical analysis. All authors revised draft of the manu- scripts and have approved the final manuscript. Finally, authors thank Helen Morrison for language revision. REFERENCES [1] J. LeDoux, “The Emotional Brain: The Mysterious Un- derpinnings of Emotional Life,” Simon & Schuster, New York, 1996. [2] J. E. Ledoux, “Emotion Circuits in the Brain,” Annual Review of Neurosciences, Vol. 23, 2000, pp. 155-184. doi:10.1146/annurev.neuro.23.1.155 [3] J. Panksepp, “Evolution Constructed the Potential for Subjective Experience within the Neurodynamics of the Mammalian Brain,” In: P. Ekman and R. J. David son, E ds . , The Nature of Emotion: Fundamental Questions, 1994, pp. 396-399. [4] E. S. Paul, E. J. Harding and M. Mendi, “Measuring Emotional Processes in Animals: The Utility of a Cogni- tive Approach,” Neuroscience and Biobehavioral Revi ew s, Vol. 29, No. 3, 2005, pp. 469-491. doi:10.1016/j.neubiorev.2005.01.002 [5] A. Cabanac and M. Cabanac, “Heart Rate Response to Gentle Handling of Frog and Lizard,” Behavioural Proc- esses, Vol. 52, No. 2-3, 2000, pp. 89-95. doi:10.1016/S0376-6357(00)00108-X [6] M. Cabanac, “Emotion and Phylogeny,” Japanese Jour- nal of Physiology, Vol. 49, No. 1, 1999, pp. 1-10. doi:10.2170/jjphysiol.49.1 [7] A. Fischer, M. Radulovic, C. Schrick, F. Sananbenesi, J. Godovac-Zimmermann and J. Radulovic, “Hippocampal Mek/Erk Signaling Mediates Extinction of Contextual Freezing Behavior,” Neurobiology of Learning and Mem- ory, Vol. 87, No. 1, 2007, pp. 149-158. doi:10.1016/j.nlm.2006.08.003 [8] M. R. Holahan and N. M. White, “Amygdala c-Fos In- ducting Corresponds to Unconditioned and Conditioned Aversive Stimuli but Not to Freezing,” Behavioural Brain Research, Vol. 152, 2004, pp. 109-120. [9] N. Graindorge, C. Alves, A. S. Darmaillacq, R. Chichery, L. Dickel and C. Bellanger, “Effects of Dorsal and Ven- tral Vertical Lobe Electrolytic Lesions on Spatial Learn- ing and Locomotor Activity in Sepia Officinalis,” Be- havioral Neuroscience, Vol. 120, No. 5, 2006, pp. 1151- 1158. doi:10.1037/0735-7044.120.5.1151 [10] C. Alves, J. G. Boal, R. Chichery and L. Dickel, “Orien- tation in the Cuttlefish Sepia Officinalis: Response versus Place Learning,” Animal Cognition, Vol. 10, No. 1, 2007, pp. 29-36. doi:10.1007/s10071-006-0027-6 [11] E.-T. Ang, G. S. Dawe, P. T. H. Wong, S. Moochhala and Y.-K. Ng, “Alterations in Spatial Learning and Memory after Forced Exercise,” Brain Research, Vol. 1113, No. 1, 2006, pp. 186-193. doi:10.1016/j.brainres.2006.07.023 [12] R. A. Countryman, N. L. Kaban and P. J. Colombo, “Hippocampal c-Fos Is Necessary for Long-Term Mem- ory of a Socially Transmitted Food Preference,” Neuro- biology of Learning and Memory, Vol. 84, No. 3, 2005, p. 175. doi:10.1016/j.nlm.2005.07.005 [13] L. Song, W. Che, W. Min-Wei, Y. Murakami and K. Ma- tsumoto, “Impairment of the Spatial Learning and Mem- ory Induced by Learned Helplessness and Chronic Mild Stress,” Pharmacology, Biochemistry, and Behavior, Vol. 83, No. 2, 2006, pp. 186-193. doi:10.1016/j.pbb.2006.01.004 [14] J. N. Crawley, J. K. Belknap, A. Collins, J. C. Crabbe, W. Frankel, N. Henderson, et al., “Behavioral Phenotypes of Inbred Mouse Strains: Implications and Recommenda- tions for Molecular Studies,” Psychopharmacology, Vol. 132, No. 2, 1997, pp. 107-124. doi:10.1007/s002130050327 [15] K. Klapdor and F. J. van der Staay, “The Morris Water- Escape Task in Mice: Strain Differences and Effects of Intra-Maze Contrast and Brightness,” Physiology & Be- havior, Vol. 60, No. 5, 1996, pp. 1247-1254. doi:10.1016/S0031-9384(96)00224-7 [16] P. Carlier and M. Jamon, “Observational Learning in C57BL/6j Mice,” Behavioral Brain Research, Vol. 174, No. 1, 2006, pp. 125-131. doi:10.1016/j.bbr.2006.07.014 [17] R. G. M. Morris, P. Garrud, J. N. P. Rawlins and J. O’Keefe, “Place Navigation Impaired in Rats with Hip- pocampal Lesions,” Nature, Vol. 297, 1982, pp. 681-683. doi:10.1038/297681a0 [18] R. Lalonde, “The Neurobiological Basis of Spontaneous Alternation,” Neuroscience & Biobehavioral Reviews, Vol. 26, No. 1, 2002, pp. 91-104. doi:10.1016/S0149-7634(01)00041-0 [19] D. Wahlsten, S. F. Cooper and J. C. Crabbe, “Different Rankings of Inbred Mouse Strains on the Morris Maze and a Refined 4-Arm Water Escape Task,” Behavioral Brain Research, Vol. 165, No. 1, 2005, pp. 36-51. doi:10.1016/j.bbr.2005.06.047 [20] D. Wahlsten, N. R. Rustay, P. Metten and J. C. Crabbe, “In Search of a Better Mouse Test,” Trends in Neurosci- ences, Vol. 26, No. 3, 2003, pp. 132-136. doi:10.1016/S0166-2236(03)00033-X [21] V. Carola, F. D’Olimpio, E. Brunamonti, A. Bevilacqua, P. Renzi and F. Mangia, “Anxiety-Related Behaviour in C57BL/6-BALB/c Chimeric Mice,” Behavioral Brain Re- search, Vol. 150, No. 1-2, 2004, pp. 25-32. [22] V. Kazlauckas, J. Schuh, O. P. Dall’Igna, G. S. Pereira, C. D. Bonan and D. R. Lara, “Behavioral and Cognitive Pro- file of Mice with High a nd Low Exploratory Phenoty pe s,” Behavioural Brain Research, Vol. 162, No. 2, 2005, p. 272. doi:10.1016/j.bbr.2005.03.021 [23] S. J. Clapcote and J. C. Roder, “Survey of Embryonic Stem Cell Line Source Strains in the Water Maze Reveals Superior Reversal Learning of 129S6/SvEvTac Mice,” Behavioural Brain Research, Vol. 152, 2004, pp. 35-48. [24] E. H. Owen, S. F. Logue, D. L. Rasmussen and J. M. Wehner, “Assessment of Learning by the Morris Water Task and Fear Conditioning in Inbred Mouse Strains and F1 Hybrids: Implications of Genetic Background for Sin- gle Genemutations and Quantitative Trai Loci Analyses,” Neuroscience, Vol. 80, No. 4, 1997, pp. 1087-1099. doi:10.1016/S0306-4522(97)00165-6 Copyright © 2012 SciRes. JBBS
 J. CELESTINE ET AL. Copyright © 2012 SciRes. JBBS 161 [25] V. Voikar, S. Koks, E. Vasar and H. Rauvala, “Strain and Gender Differences in the Behavior of Mouse Lines Com- monly Used in Transgenic Studies,” Physiology & Be- havior, Vol. 72, No. 1-2, 2001, pp. 271-281. doi:10.1016/S0031-9384(00)00405-4 [26] M. Yoshida, K. Goto and S. Watanabe, “Task-Dependent Strain Difference of Spatial Learning in C57BL/6N and BALB/c Mice,” Physiology and Behavior, Vol. 73, No. 1-2, 2001, pp. 37-42. doi:10.1016/S0031-9384(01)00419-X [27] K. Klapdor and F. J. Van Der Staay, “Repeated Acquisi- tion of a Spatial Navigation Task in Mice: Effects of Spacing of Trials and of Unilateral Middle Cerebral Ar- tery Occlusion,” Physiology & Behavior, Vol. 63, No. 5, 1998, pp. 903-909. doi:10.1016/S0031-9384(98)00003-1 [28] D. Van Dam, G. Lenders and P. P. De Deyn, “Effect of Morris Water Maze Diameter on Visual-Spatial Learning in Different Mouse Strains,” Neurobiology of Learning and Memory, Vol. 85, No. 2, 2006, pp. 164-172. doi:10.1016/j.nlm.2005.09.006 [29] R. D’Hooge and P. P. De Deyn, “Applications of the Morris Water Maze in the Study of Learning and Mem- ory,” Brain Research Reviews, Vol. 36, No. 1, 2001, pp. 60-90. doi:10.1016/S0165-0173(01)00067-4 [30] P. Chapillon and A. Debouzie, “BALB/c Mice Are Not So Bad in the Morris Water Maze,” Behavioral Brain Research, Vol. 117, No. 1-2, 2000, pp. 115-118. doi:10.1016/S0166-4328(00)00292-8 [31] Y. Ibarguen-Vargas, A. Surget, C. Touma, R. Palme and C. Belzung, “Multifaceted Strain-Specific Effects in a Mouse Model of Depression and of Antidepressant Re- versal,” Psychoneuroe ndocrinolo gy, Vol. 33, No . 10, 2008, pp. 1357-1368. doi:10.1016/j.psyneuen.2008.07.010 [32] K. Yamada, Y. Santo-Yamada and K. Wada, “Stress- Induced Impairment of Inhibitory Avoidance Learning in Female Neuromedin B Receptor-Deficient Mice,” Physi- ology & Behavior, Vol. 78, No. 2, 2003, p. 303. doi:10.1016/S0031-9384(02)00979-4 [33] D. D. Francis, D. M. Zaharia, N. Shanks and H. Anisman, “Stress-Induced Disturbances in Morris Water-Maze Per- formance: Interstain Variability,” Physiology and Beh avior, Vol. 58, No. 1, 1995, pp. 57-65. doi:10.1016/0031-9384(95)00009-8 [34] C. Pittenger and R. S. Duman, “Stress, Depression, and Neuroplasticity: A Convergence of Mechanisms,” Neu- ropsychopharmacology, Vol. 33, No. 1, 2007, pp. 88-109. doi:10.1038/sj.npp.1301574 [35] L. Santarelli, M. Saxe, C. Gross, A. Surget, F. Battaglia, S. Dulawa, et al., “Requirement of Hippocampal Neuro- genesis for the Behavioral Effects of Antidepressants,” Science, Vol. 301, No. 5634, 2003, pp. 805-809. doi:10.1126/science.1083328 [36] R. E. Brown and A. A. Wong, “The Influence of Visual Ability on Learning and Memory Performance in 13 Strains of Mice,” Learning & Memory, Vol. 14, 2007, pp. 134-144. doi:10.1101/lm.473907 [37] S. S. Moy, J. J. Nadler, N. B. Young, A. Perez, L. P. Hol- loway, R. P. Barbaro, et al., “Mouse Behavioral Tasks Relevant to Autism: Phenotypes of 10 Inbred Strains,” Behavioural Brain Research, Vol. 176, No. 1, 2007, pp. 4-20. doi:10.1016/j.bbr.2006.07.030 [38] M. Upchurch and J. M. Wehner, “Differences between Inbred Strains of Mice in Morris Water Maze Perform- ance,” Behavior Genetics, Vol. 18, No. 1, 1988, pp. 55- 68. doi:10.1007/BF01067075 [39] J. C. Crabbe, D. Wahlsten and B. C. Dudek, “Genetics of Mouse Behavior: Interactions with Laboratory Environ- ment,” Science, Vol. 284, No. 5420, 1999, pp. 1670-1672. doi:10.1126/science.284.54 20.1670 [40] V. Carola, F. D’Olimpio, E. Brunamonti, F. Mangia and P. Renzi, “Evaluation of the Elevated Plus-Maze and Open-Field Tests for the Assessment of Anxiety-Related Behaviour in Inbred Mice,” Behavioral Brain Research, Vol. 134, No. 1-2, 2002, pp. 49-57. doi:10.1016/S0166-4328(01)00452-1 [41] D. O. Hebb, “Drives and the C.N.S. (Conceptual Nervous System),” Psychological Review, Vol. 62, 1955, pp. 243- 254. doi:10.1037/h0041823 [42] A. Briones-Aranda, L. Rocha and O. Picazo, “Influence of Forced Swimming Stress on 5-HT1A Receptors and Serotonin Levels in Mouse Brain,” Progress in Neuro- Psychopharmacology & Biological Psychiatry, Vol. 29, No. 2, 2005, pp. 275-281. doi:10.1016/j.pnpbp.2004.11.011 [43] Y. Xu, B. Ku, L. Tie, H. Yao, W. Jiang, X. Ma, et al., “Curcumin Reverses the Effects of Chronic Stress on Behavior, the HPA Axis, BDNF Expression and Phos- phorylation of CREB,” Brain Research, Vol. 1122, No. 1, 2006, pp. 56-64. doi:10.1016/j.brainres.2006.09.009 [44] A. Olariu, M. H. Tran, K. Yamada, M. Mizuno, V. Hefco and T. Nabeshima, “Memory Deficits and Increased Emotionality Induced by Beta-Amyloid (25-35) Are Cor- related with the Reduced Acetylcholine Release and Al- tered Phorbol Dibutyrate Binding in the Hippocampus,” Journal of Neural Transmission, Vol. 108, No. 8-9, 2001, pp. 1065-1079. doi:10.1007/s007020170025 [45] N. M. Conejo, M. Lopez, R. Cantora, H. Gonzalez-Pardo, L. Lopez, A. Begega, et al., “Effects of Pavlovian Fear Conditioning on Septohippocampal Metabolism in Rats,” Neuroscience Letters, Vol. 373, No. 2, 2005, pp. 94-98. doi:10.1016/j.neulet.2004.09.066 [46] M. Fujisaki, K. Hashimoto, M. Iyo and T. Chiba, “Role of the Amygdalo-Hippocampal Transition Area in the Fear Expression: Evaluation by Behavior and Immediate Early Gene Expression,” Neuroscience, Vol. 124, No. 1, 2004, pp. 247-260. doi:10.1016/j.neuroscience.2003.11.022 [47] C.-H. Lin, S.-H. Yeh, C.-H. Lin, K.-T. Lu, T.-H. Leu, W.-C. Chang, et al., “A role of PI-3 Kinase Signaling Pathway in Fear Conditioning and Synaptic Plasticity in the Amygdala,” Neuron, Vol. 31, No. 5, 2001, pp. 841- 851. doi:10.1016/S0896-6273(01)00433-0 [48] P. Barnes and M. Good, “Impaired Pavlovian Cued Fear Conditioning in Tg2576 Mice Expressing a Human Mu- tant Amyloid Precursor Protein Gene,” Behavioral Brain Research, Vol. 157, No. 1, 2005, pp. 107-117. doi:10.1016/j.bbr.2004.06.014
|