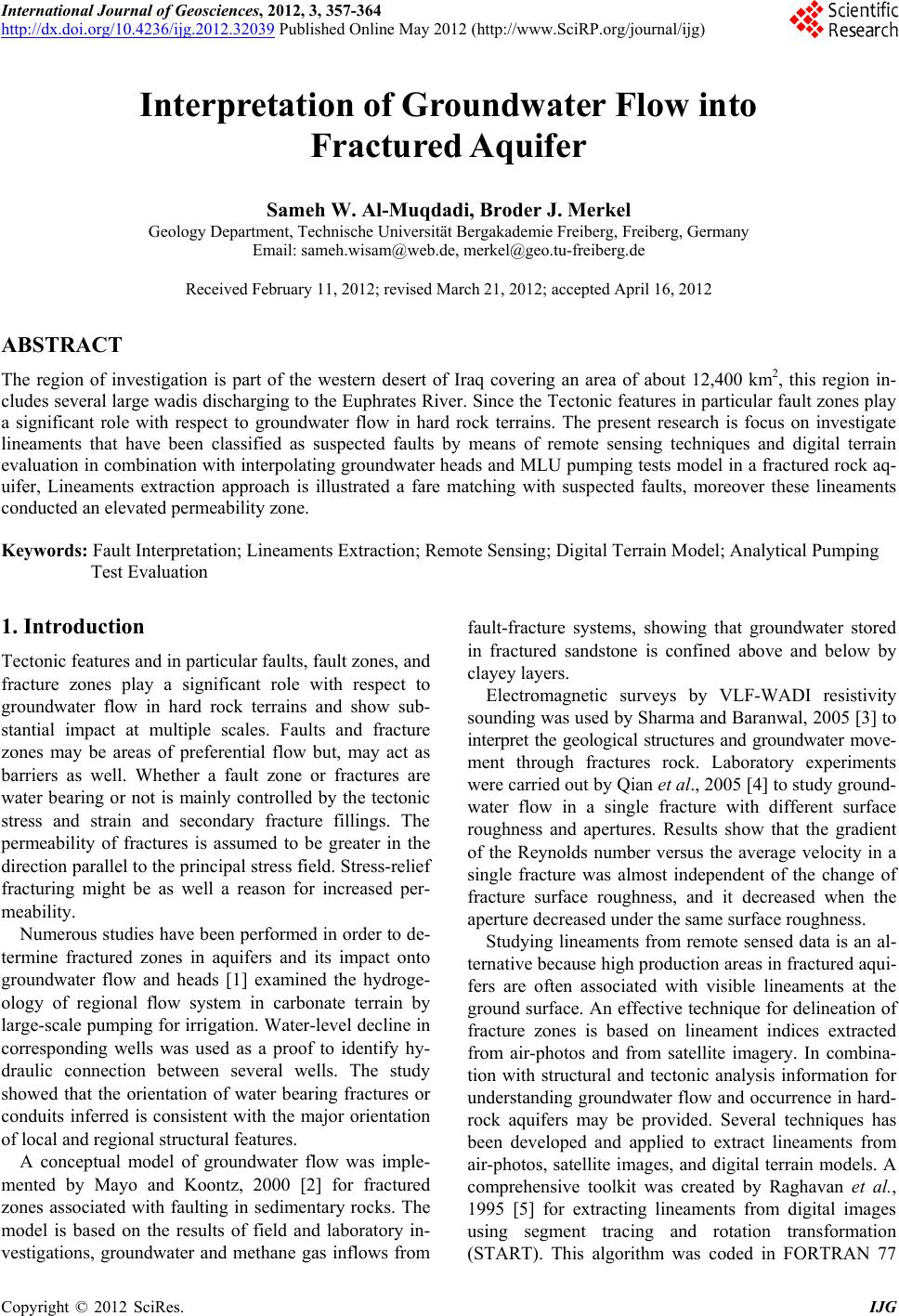
International Journal of Geosciences, 2012, 3, 357-364
http://dx.doi.org/10.4236/ijg.2012.32039 Published Online May 2012 (http://www.SciRP.org/journal/ijg)
Interpretation of Groundwater Flow into
Fractured Aquifer
Sameh W. Al-Muqdadi, Broder J. Merkel
Geology Department, Technische Universität Bergakademie Freiberg, Freiberg, Germany
Email: sameh.wisam@web.de, merkel@geo.tu-freiberg.de
Received February 11, 2012; revised March 21, 2012; accepted April 16, 2012
ABSTRACT
The region of investigation is part of the western desert of Iraq covering an area of about 12,400 km2, this region in-
cludes several large wadis discharging to the Euphrates River. Since the Tectonic features in particular fault zones play
a significant role with respect to groundwater flow in hard rock terrains. The present research is focus on investigate
lineaments that have been classified as suspected faults by means of remote sensing techniques and digital terrain
evaluation in combination with interpolating groundwater heads and MLU pumping tests model in a fractured rock aq-
uifer, Lineaments extraction approach is illustrated a fare matching with suspected faults, moreover these lineaments
conducted an elevated permeability zone.
Keywords: Fault Interpretation; Lineaments Extraction; Remote Sensing; Digital Terrain Model; Analytical Pumping
Test Evaluation
1. Introduction
Tectonic features and in particular faults, fault zones, and
fracture zones play a significant role with respect to
groundwater flow in hard rock terrains and show sub-
stantial impact at multiple scales. Faults and fracture
zones may be areas of preferential flow but, may act as
barriers as well. Whether a fault zone or fractures are
water bearing or not is mainly controlled by the tectonic
stress and strain and secondary fracture fillings. The
permeability of fractures is assumed to be greater in the
direction parallel to the principal stress field. Stress-relief
fracturing might be as well a reason for increased per-
meability.
Numerous studies have been performed in order to de-
termine fractured zones in aquifers and its impact onto
groundwater flow and heads [1] examined the hydroge-
ology of regional flow system in carbonate terrain by
large-scale pumping for irrigation. Water-level decline in
corresponding wells was used as a proof to identify hy-
draulic connection between several wells. The study
showed that the orientation of water bearing fractures or
conduits inferred is consistent with the major orientation
of local and regional structural features.
A conceptual model of groundwater flow was imple-
mented by Mayo and Koontz, 2000 [2] for fractured
zones associated with faulting in sedimentary rocks. The
model is based on the results of field and laboratory in-
vestigations, groundwater and methane gas inflows from
fault-fracture systems, showing that groundwater stored
in fractured sandstone is confined above and below by
clayey layers.
Electromagnetic surveys by VLF-WADI resistivity
sounding was used by Sharma and Baranwal, 2005 [3] to
interpret the geological structures and groundwater move-
ment through fractures rock. Laboratory experiments
were carried out by Qian et al., 2005 [4] to study ground-
water flow in a single fracture with different surface
roughness and apertures. Results show that the gradient
of the Reynolds number versus the average velocity in a
single fracture was almost independent of the change of
fracture surface roughness, and it decreased when the
aperture decreased under the same surface roughness.
Studying lineaments from remote sensed data is an al-
ternative because high production areas in fractured aqui-
fers are often associated with visible lineaments at the
ground surface. An effective technique for delineation of
fracture zones is based on lineament indices extracted
from air-photos and from satellite imagery. In combina-
tion with structural and tectonic analysis information for
understanding groundwater flow and occurrence in hard-
rock aquifers may be provided. Several techniques has
been developed and applied to extract lineaments from
air-photos, satellite images, and digital terrain models. A
comprehensive toolkit was created by Raghavan et al.,
1995 [5] for extracting lineaments from digital images
using segment tracing and rotation transformation
(START). This algorithm was coded in FORTRAN 77
C
opyright © 2012 SciRes. IJG