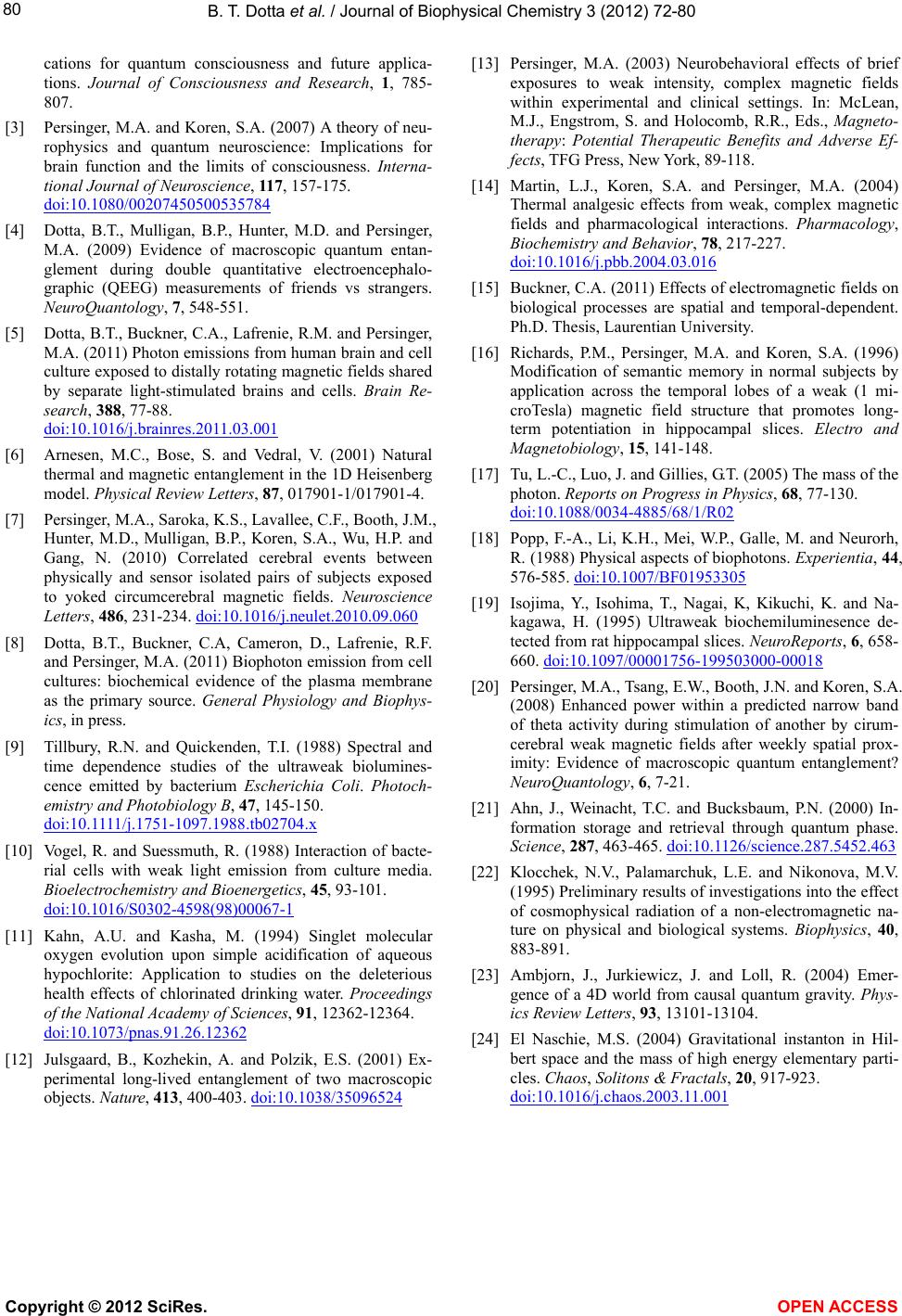
B. T. Dotta et al. / Journal of Biophysical Chemistry 3 (2012) 72-80
Copyright © 2012 SciRes. OPEN ACCESS
80
cations for quantum consciousness and future applica-
tions. Journal of Consciousness and Research, 1, 785-
807.
[3] Persinger, M.A. and Koren, S.A. (2007) A theory of neu-
rophysics and quantum neuroscience: Implications for
brain function and the limits of consciousness. Interna-
tional Journal of Neuroscience, 117, 157-175.
doi:10.1080/00207450500535784
[4] Dotta, B.T., Mulligan, B.P., Hunter, M.D. and Persinger,
M.A. (2009) Evidence of macroscopic quantum entan-
glement during double quantitative electroencephalo-
graphic (QEEG) measurements of friends vs strangers.
NeuroQuantology, 7, 548-551.
[5] Dotta, B.T., Buckner, C.A., Lafrenie, R.M. and Persinger,
M.A. (2011) Photon emissions from human brain and cell
culture exposed to distally rotating magnetic fields shared
by separate light-stimulated brains and cells. Brain Re-
search, 388, 77-88.
doi:10.1016/j.brainres.2011.03.001
[6] Arnesen, M.C., Bose, S. and Vedral, V. (2001) Natural
thermal and magnetic entanglement in the 1D Heisenberg
model. Physical Re view Letters, 87, 017901-1/017901-4.
[7] Persinger, M.A., Saroka, K.S., Lavallee, C.F., Booth, J.M.,
Hunter, M.D., Mulligan, B.P., Koren, S.A., Wu, H.P. and
Gang, N. (2010) Correlated cerebral events between
physically and sensor isolated pairs of subjects exposed
to yoked circumcerebral magnetic fields. Neuroscience
Letters, 486, 231-234. doi:10.1016/j.neulet.2010.09.060
[8] Dotta, B.T., Buckner, C.A, Cameron, D., Lafrenie, R.F.
and Persinger, M.A. (2011) Biophoton emission from cell
cultures: biochemical evidence of the plasma membrane
as the primary source. General Physiology and Biophys-
ics, in press.
[9] Tillbury, R.N. and Quickenden, T.I. (1988) Spectral and
time dependence studies of the ultraweak biolumines-
cence emitted by bacterium Escherichia Coli. Photoch-
emistry and Photobiology B, 47, 145-150.
doi:10.1111/j.1751-1097.1988.tb02704.x
[10] Vogel, R. and Suessmuth, R. (1988) Interaction of bacte-
rial cells with weak light emission from culture media.
Bioelectrochemistry and Bioenergetics, 45, 93-101.
doi:10.1016/S0302-4598(98)00067-1
[11] Kahn, A.U. and Kasha, M. (1994) Singlet molecular
oxygen evolution upon simple acidification of aqueous
hypochlorite: Application to studies on the deleterious
health effects of chlorinated drinking water. Proceedings
of the National Academy of Sciences, 91, 12362-12364.
doi:10.1073/pnas.91.26.12362
[12] Julsgaard, B., Kozhekin, A. and Polzik, E.S. (2001) Ex-
perimental long-lived entanglement of two macroscopic
objects. Nature, 413, 400-403. doi:10.1038/35096524
[13] Persinger, M.A. (2003) Neurobehavioral effects of brief
exposures to weak intensity, complex magnetic fields
within experimental and clinical settings. In: McLean,
M.J., Engstrom, S. and Holocomb, R.R., Eds., Magneto-
therapy: Potential Therapeutic Benefits and Adverse Ef-
fects, TFG Press, New York, 89-118.
[14] Martin, L.J., Koren, S.A. and Persinger, M.A. (2004)
Thermal analgesic effects from weak, complex magnetic
fields and pharmacological interactions. Pharmacology,
Biochemistry and Behavior, 78, 217-227.
doi:10.1016/j.pbb.2004.03.016
[15] Buckner, C.A. (2011) Effects of electromagnetic fields on
biological processes are spatial and temporal-dependent.
Ph.D. Thesis, Laurentian University.
[16] Richards, P.M., Persinger, M.A. and Koren, S.A. (1996)
Modification of semantic memory in normal subjects by
application across the temporal lobes of a weak (1 mi-
croTesla) magnetic field structure that promotes long-
term potentiation in hippocampal slices. Electro and
Magnetobiology, 15, 141-148.
[17] Tu, L.-C., Luo, J. and Gillies, G.T. (2005) The mass of the
photon. Reports on Progress in Physics, 68, 77-130.
doi:10.1088/0034-4885/68/1/R02
[18] Popp, F.-A., Li, K.H., Mei, W.P., Galle, M. and Neurorh,
R. (1988) Physical aspects of biophotons. Experientia, 44,
576-585. doi:10.1007/BF01953305
[19] Isojima, Y., Isohima, T., Nagai, K, Kikuchi, K. and Na-
kagawa, H. (1995) Ultraweak biochemiluminesence de-
tected from rat hippocampal slices. NeuroReports, 6, 658-
660. doi:10.1097/00001756-199503000-00018
[20] Persinger, M.A., Tsang, E.W., Booth, J.N. and Koren, S.A.
(2008) Enhanced power within a predicted narrow band
of theta activity during stimulation of another by cirum-
cerebral weak magnetic fields after weekly spatial prox-
imity: Evidence of macroscopic quantum entanglement?
NeuroQuantology, 6, 7-21.
[21] Ahn, J., Weinacht, T.C. and Bucksbaum, P.N. (2000) In-
formation storage and retrieval through quantum phase.
Science, 287, 463-465. doi:10.1126/science.287.5452.463
[22] Klocchek, N.V., Palamarchuk, L.E. and Nikonova, M.V.
(1995) Preliminary results of investigations into the effect
of cosmophysical radiation of a non-electromagnetic na-
ture on physical and biological systems. Biophysics, 40,
883-891.
[23] Ambjorn, J., Jurkiewicz, J. and Loll, R. (2004) Emer-
gence of a 4D world from causal quantum gravity. Phys-
ics Review Letters, 93, 13101-13104.
[24] El Naschie, M.S. (2004) Gravitational instanton in Hil-
bert space and the mass of high energy elementary parti-
cles. Chaos, Solitons & Fractals, 20, 917-923.
doi:10.1016/j.chaos.2003.11.001