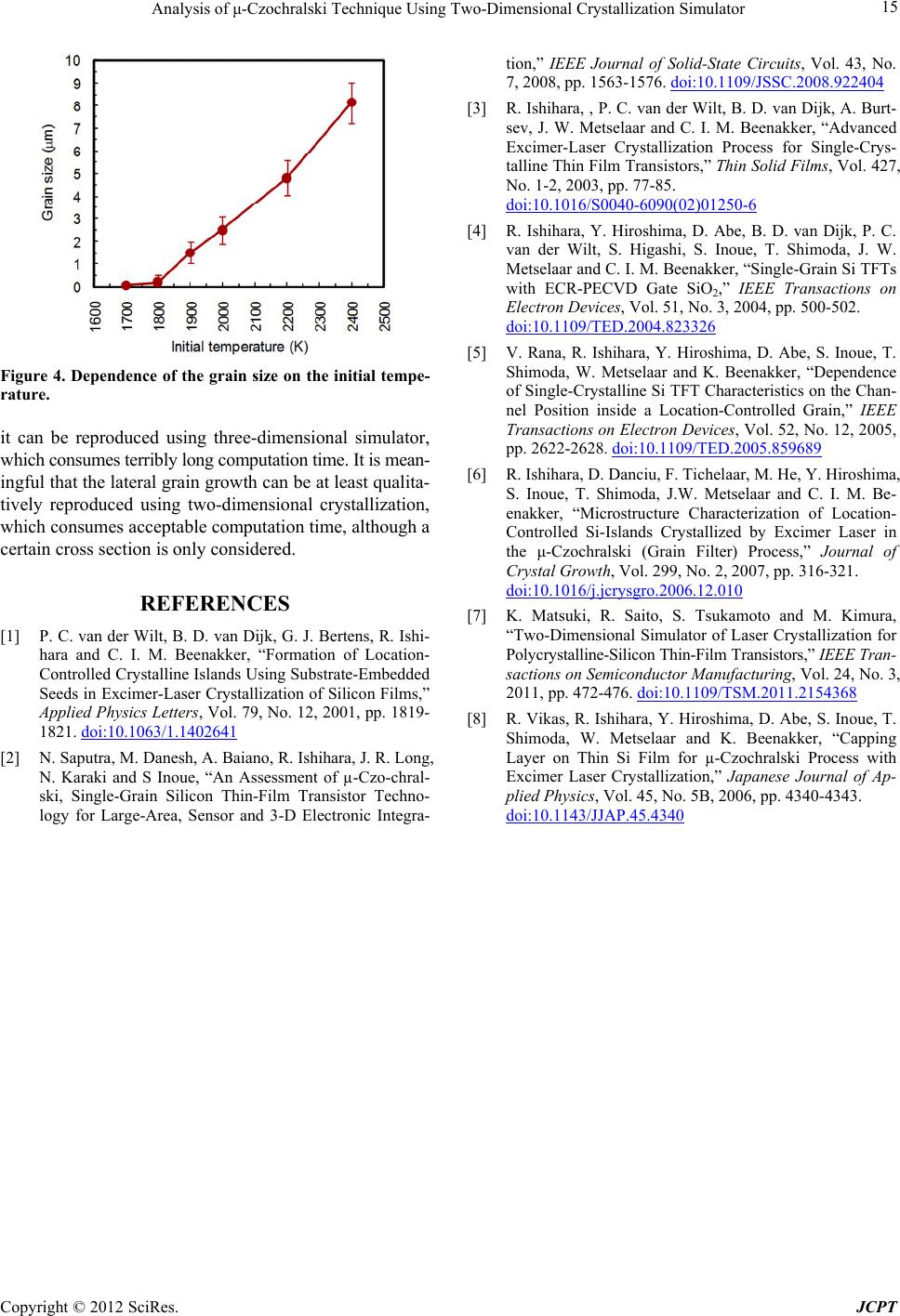
Analysis of μ-Czochralski Technique Using Two-Dimensional Crystallization Simulator 15
Figure 4. Dependence of the grain size on the initial tempe-
rature.
it can be reproduced using three-dimensional simulator,
which consumes terribly long computation time. It is mean-
ingful that the lateral grain growth can be at least qualita-
tively reproduced using two-dimensional crystallization,
which consumes acceptable computation time, although a
certain cross section is only considered.
REFERENCES
[1] P. C. van der Wilt, B. D. van Dijk, G. J. Bertens, R. Ishi-
hara and C. I. M. Beenakker, “Formation of Location-
Controlled Crystalline Islands Using Substrate-Embedded
Seeds in Excimer-Laser Crystallization of Silicon Films,”
Applied Physics Letters, Vol. 79, No. 12, 2001, pp. 1819-
1821. doi:10.1063/1.1402641
[2] N. Saputra, M. Danesh, A. Baiano, R. Ishihara, J. R. Long,
N. Karaki and S Inoue, “An Assessment of µ-Czo-chral-
ski, Single-Grain Silicon Thin-Film Transistor Techno-
logy for Large-Area, Sensor and 3-D Electronic Integra-
tion,” IEEE Journal of Solid-State Circuits, Vol. 43, No.
7, 2008, pp. 1563-1576. doi:10.1109/JSSC.2008.922404
[3] R. Ishihara, , P. C. van der Wilt, B. D. van Dijk, A. Burt-
sev, J. W. Metselaar and C. I. M. Beenakker, “Advanced
Excimer-Laser Crystallization Process for Single-Crys-
talline Thin Film Transistors,” Thin Solid Films, Vol. 427,
No. 1-2, 2003, pp. 77-85.
doi:10.1016/S0040-6090(02)01250-6
[4] R. Ishihara, Y. Hiroshima, D. Abe, B. D. van Dijk, P. C.
van der Wilt, S. Higashi, S. Inoue, T. Shimoda, J. W.
Metselaar and C. I. M. Beenakker, “Single-Grain Si TFTs
with ECR-PECVD Gate SiO2,” IEEE Transactions on
Electron Devi ces, Vol. 51, No. 3, 2004, pp. 500-502.
doi:10.1109/TED.2004.823326
[5] V. Rana, R. Ishihara, Y. Hiroshima, D. Abe, S. Inoue, T.
Shimoda, W. Metselaar and K. Beenakker, “Dependence
of Single-Crystalline Si TFT Characteristics on the Chan-
nel Position inside a Location-Controlled Grain,” IEEE
Transactions on Electron Devices, Vol. 52, No. 12, 2005,
pp. 2622-2628. doi:10.1109/TED.2005.859689
[6] R. Ishihara, D. Danciu, F. Tichelaar, M. He, Y. Hiroshima,
S. Inoue, T. Shimoda, J.W. Metselaar and C. I. M. Be-
enakker, “Microstructure Characterization of Location-
Controlled Si-Islands Crystallized by Excimer Laser in
the μ-Czochralski (Grain Filter) Process,” Journal of
Crystal Growth, Vol. 299, No. 2, 2007, pp. 316-321.
doi:10.1016/j.jcrysgro.2006.12.010
[7] K. Matsuki, R. Saito, S. Tsukamoto and M. Kimura,
“Two-Dimensional Simulator of Laser Crystallization for
Polycrystalline-Silicon Thin-Film Transistors,” IEE E Tran-
sactions on Semiconductor Manufacturing, Vol. 24, No. 3,
2011, pp. 472-476. doi:10.1109/TSM.2011.2154368
[8] R. Vikas, R. Ishihara, Y. Hiroshima, D. Abe, S. Inoue, T.
Shimoda, W. Metselaar and K. Beenakker, “Capping
Layer on Thin Si Film for µ-Czochralski Process with
Excimer Laser Crystallization,” Japanese Journal of Ap-
plied Physics, Vol. 45, No. 5B, 2006, pp. 4340-4343.
doi:10.1143/JJAP.45.4340
Copyright © 2012 SciRes. JCPT