 International Journal of Organic Chemistry, 2011, 1, 202-206 doi:10.4236/ijoc.2011.14029 Published Online December 2011 (http://www.SciRP.org/journal/ijoc) Copyright © 2011 SciRes. IJOC The Synthesis of Arylsulfonylphthalimides and Their Reactions with Several Amines in Acetonitrile Seyhan Ozturk, Halil Kutuk* Department of C hemi st ry , Faculty of Arts and Sciences, Ondokuz Mayıs University, Samsun, Turkey E-mail: *hkutuk@omu.edu.tr Received August 24, 2011; revised October 14, 2011; accepted October 23, 2011 Abstract In this study, several N-(p-substituted-arylsulfonyl)phthalimides (1a-e) were synthesized. The synthesized compounds were then examined with respect to their substitution reactions with t-butylamine, diethylamine, cyclohexylamine, and trans-1,2-diaminocyclohexane in acetonitrile. In order to determine the mechanism, substituent effect, activation entropy, and nucleophile effect were used as criteria. Keywords: Arylsulfonyl Phthalimides, Mechanism, Substituent Effect, Activation Entropy 1. Introduction N-Alkyl and N-arylsulfonyl phthalimides were prepared by Heller [1] from the reaction of potassium phthalimide and sulfonyl chlorides. Earlier attempts by Evans and Dehn to prepare several N-aryl derivatives [2-3] and later by Scott and Lutz to prepare some N-alkyl derivatives by this reaction had been unsuccessful [4]. Later, potassium phthalimide was reported to interact with p-toluenesul- fonyl chloride at 140˚C or in dimethylformamide at 5˚C to 40˚C to yield N-(p-tolylsulfonyl)phthalimide [5]. The mechanism of acid-catalyzed hydrolysis of N-(p-substi- tuted-arylsulfonyl)phthalimides was studied in detail in our laboratory [6]. We now report a complementary stu- dy of the nucleophilic substitution reactions of a series of N-(p-substituted-arylsulfonyl)phthalimides (1a-e) in ace- tonitrile (Scheme 1). 2. Results and Discussions In this study, N-(p-methoxyphenylsulfonyl)phthalimide (1a), N-(p-toluenesulfonyl)phthalimide (1b), N-(phenyl- sulfonyl)phthalimide (1c), N-(p-bromophenylsulfonyl) phthalimide (1d) and N-(p-nitrophenylsulfonyl)phtha- li- mide (1e) were synthesized. The synthesized compoun- ds were examined with respect to their substitution reac- tions with t-butylamine, diethylamine, cyclohexylamine, and trans-1,2-diaminocyclohexane. In order to determi- ne the mechanism, substituent effect, activation entropy and nucleophile effect were used as criteria. The substituent effect was investigated at 30.0˚C ± 0.1˚C in acetonitrile. Positive ρ values were obtained for the substitution of N-(p-substituted-arylsulfonyl)phthali- mides with t-butylamine, diethylamine, cyclohexylamine, and trans-1,2-diaminocyclohexane. Electron withdrawing substituents (-Br, -NO2) increased the reaction rate, while electron donating substituents (-CH3, -OCH3) led to a de- crease (Figures 1-4). A positive value indicates the SN2 mechanism or an addition-elimination mechanism. The values for the reaction of N-(p-substituted-arylsulfonyl) phthalimides in acetonitrile with t-butylamine, diethyl- amine, cyclohexylamine, and trans-1,2-diaminocyclohe- xane were 1.18, 1.12, 1.05 and 1.14 respectively. A similar behavior was observed for the alkaline hydrolysis of sulfonimidic esters and reactions of sulfinylphthalim- ides with several nucleophiles [7,8]. Scheme 1. N-(p-Substitued-arylsulfonyl)phthalimides.
 203 S. OZTURK ET AL. Figure 1. Hammett Plots of logk2/k0 versus for the reactions of 1a-e with t-butylamine at 30.0˚C 0.1 ˚C in acetonitrile. Figure 2. Hammett Plots of logk2/k0 versus for the reactions of 1a-e with diethyla mine at 30.0˚C 0.1˚C in acetonitrile. Figure 3. Hammett Plots of logk2/k0 versus for the reactions of 1a-e with cyclohexylamine at 30.0˚C 0.1˚C in acetonitrile. The activation entropy was also studied, and negative ΔS≠ values were obtained. The ΔS≠ values for the re- action of N-(phenylsulfonyl)phthalimides in acetonitrile with t-butylamine, diethylamine, cyclohexylamine, and trans-1,2-diaminocyclohexane were –148.94, –106.55, –132.02 and –48.78 J/mol·K respectively. The negative ΔS≠ values indicate that the reaction followed the SN2 mechanism or an addition-elimination mechanism. Similar Figure 4. Hammett Plots of logk2/k0 versus for the reac- tions of 1a-e with trans-1,2-diaminocyclohexane at 30.0˚C 0.1˚C in acetonitrile. behavior was observed for the aminolysis of 1-tosyl- 3-methyl imidazolium chloride as well [9]. Arrhenius pa- rameters for the reaction of N-(phenylsulfonyl)phth- ali- mides in acetonitrile with t-butylamine, diethylamine, cyclohexylamine, and trans-1,2-diaminocyclohexane are shown in Table 1. Second order kinetics, showing dependence both on the nucleophile and on the substrate, are widely observed in nucleophilic substitutions [10]. It was also observed that the reactions with cyclohexylamine, and trans-1,2-dia- minocyclohexane nucleophiles took place much faster than those with t-butylamine and diethylamine nucleo- philes as shown in Table 2. In the light of the overall evidence, we propose that the substitution reactions of a series of N-(p-substitut ed-arylsulfonyl)phthalimides with t-butylamine, diethy- lamine, cyclohexylamine and trans-1,2-diaminocyclohe- xane occur with SN2 mechanism or an addition-elimina- tion mechanism, as shown in Schemes 2 and 3 respec- tively. 3. Experimental 3.1. Materials and Methods N-(p-Substituted-arylsulfonyl)phthalimides 1a-e were pre- pared from the corresponding p-substituted-arylsulfonyl Table 1. Activation parameters for the reaction of N-(phe- nylsulfonyl) phthalimide in acetonitrile with t-butylamine, diethylamine, cyclohexylamine, and trans-1,2-diaminocy- clohexane. Nucleophile H ( kJ/mol) S (J/mol·K) R2 t-Butylamine 29.75 –148.94 0.9981 Diethylamine 39.60 –106.55 0.9978 Cyclohexylamine 20.66 –132.02 0.9928 trans-1,2-Diaminocyclohexane46.18 –48.78 0.9829 logk2/k0 logk2/k0 logk2/k0 logk2/k0 Copyright © 2011 SciRes. IJOC
 S. OZTURK ET AL. Copyright © 2011 SciRes. IJOC 204 Table 2. Values of k2 (M–1s–1) for the substitution of N-(p- substitutedarylsulfonyl) phthalimides with nucleophiles at 30.0˚C 0.1˚C in acetonitrile. chlorides with potassium phthalimides in acetonitrile as described by Heller [1]. All melting points were deter- mined using an electrothermal digital melting point ap- paratus. Nucleophile Substituent k2 (M–1s–1) 1a 0.47 1b 0.68 1c 0.87 1d 1.29 t-Butylamine 1e 3.91 1a 1.41 1b 1.91 1c 2.63 1d 6.06 Diethylamine 1e 10.51 1a 108.63 1b 147.78 1c 210.64 1d 363.74 Cyclohexylamine 1e 1093.56 1a 102.07 1b 123.40 1c 204.84 1d 276.86 trans-1,2-Diaminocyclohexane 1e 856.09 1a: m.p. 218˚C - 219˚C (Lit.11 218˚C - 219˚C). 1H NMR (400 MHz, DMSO-d6): 7.90 - 8.10 (d, 2H), 7.80 - 8.00 (s, 2H), 7.1 - 7.3 (d, 2H), 3.8 (s, 3H); 13C NMR (400 MHz, DMSO-d6): 164.61, 163.43, 136.27, 131.04, 130.92, 129.65, 124.70, 115.19, 56.40; IR (ATR, cm–1) 3067, 1739, 1593 - 1417, 1252 - 975, 1165, 1143, 1089, 866 - 663, 663. 1b: m.p. 240˚C - 241˚C (Lit.1 239˚C - 240˚C). 1H NMR (200 MHz, DMSO-d6): 8.40 - 7.60 (d, 4H), 7.50 - 7.00 (dd, J = 7.6 Hz, 4H), 2.34 (s, 3H); 13C NMR (200 MHz, DMSO-d6): 146.04, 135.43, 134.29, 130.97, 129.92, 128.44, 124.54, 123.59, 21.30; IR (KBr disk, cm–1) 3066, 2988, 1747, 1593 - 1466, 1256 - 965, 1178, 1088, 865 - 632, 657. 1c: m.p. 202˚C - 203˚C (Lit.1 202.5˚C - 203.5˚C). 1H NMR (200 MHz, DMSO-d6): 8.09 - 8.07 (d, 2H), 8.07 - 8.02 (dd, J = 3.4 Hz, 2H), 7.94 - 7.90 (d, 2H), 7.77 - 7.73 (d, 2H), 7.70 - 7.60 (d, 1H); 13C NMR (200 MHz, DMSO-d6): 162.60, 137.94, 135.82, 134.90, 130.54, 129.53, 127.75, 124.28; IR (KBr disk, cm–1) 3069, 1748, 1604 - 1448, 1255 - 999, 1138, 1087, 864 - 682, 682. Scheme 2. SN2 mechanism for N-(p-Substitued-arylsulfonyl)phthalimides with t-butylamine.
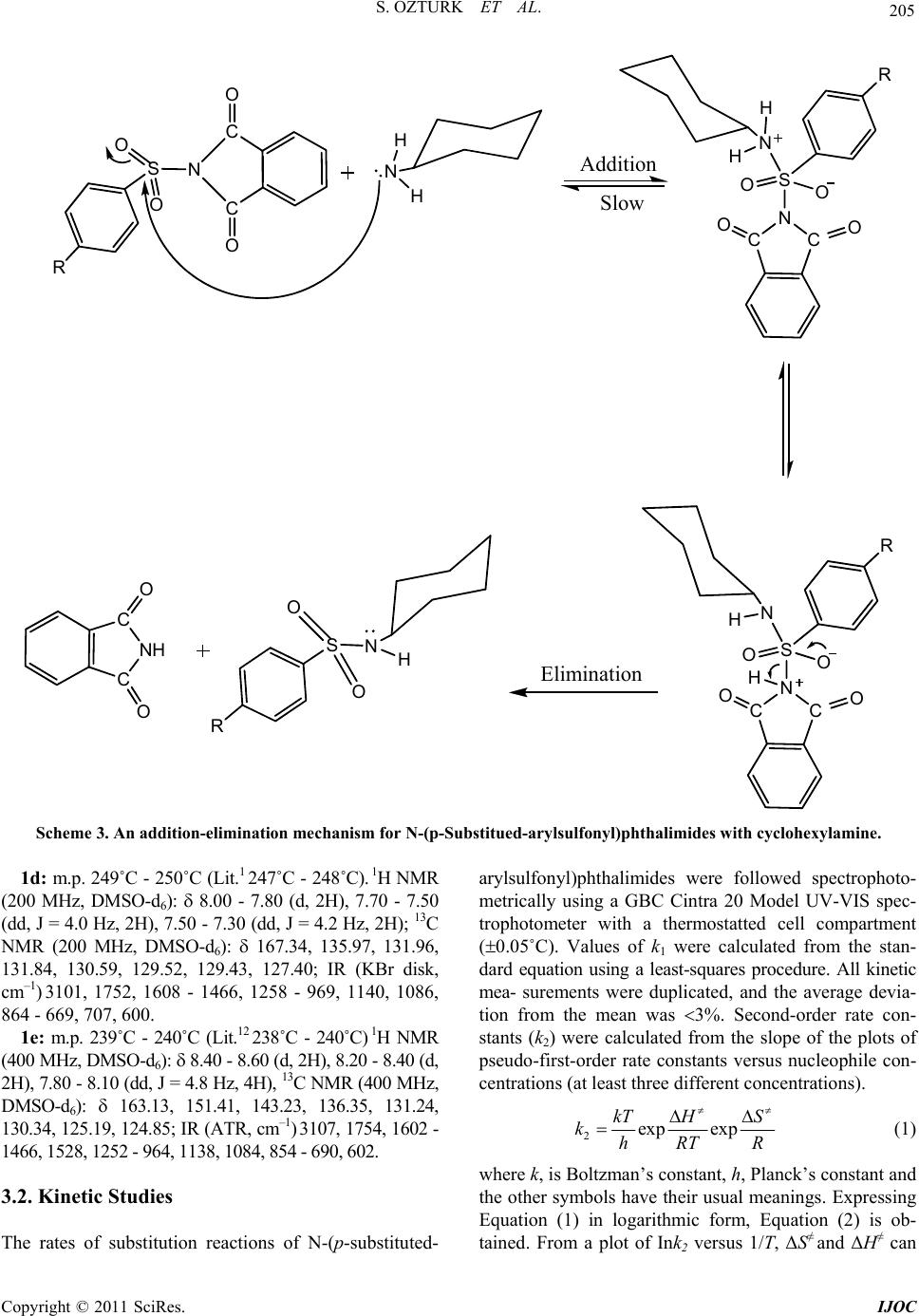 205 S. OZTURK ET AL. S O N OC C O O R Addition Slow C N C OO S OO R Elimination C NH C O OR S O O N H H C N C OO S OO R H N H H N H NH Scheme 3. An addition-elimination mechanism for N-(p-Substitued-arylsulfonyl)phthalimides with cyclohexylamine. 1d: m.p. 249˚C - 250˚C (Lit.1 247˚C - 248˚C). 1H NMR (200 MHz, DMSO-d6): 8.00 - 7.80 (d, 2H), 7.70 - 7.50 (dd, J = 4.0 Hz, 2H), 7.50 - 7.30 (dd, J = 4.2 Hz, 2H); 13C NMR (200 MHz, DMSO-d6): 167.34, 135.97, 131.96, 131.84, 130.59, 129.52, 129.43, 127.40; IR (KBr disk, cm–1) 3101, 1752, 1608 - 1466, 1258 - 969, 1140, 1086, 864 - 669, 707, 600. 1e: m.p. 239˚C - 240˚C (Lit.12 238˚C - 240˚C) 1H NMR (400 MHz, DMSO-d6): 8.40 - 8.60 (d, 2H), 8.20 - 8.40 (d, 2H), 7.80 - 8.10 (dd, J = 4.8 Hz, 4H), 13C NMR (400 MHz, DMSO-d6): 163.13, 151.41, 143.23, 136.35, 131.24, 130.34, 125.19, 124.85; IR (ATR, cm–1) 3107, 1754, 1602 - 1466, 1528, 1252 - 964, 1138, 1084, 854 - 690, 602. 3.2. Kinetic Studies The rates of substitution reactions of N-(p-substituted- arylsulfonyl)phthalimides were followed spectrophoto- metrically using a GBC Cintra 20 Model UV-VIS spec- trophotometer with a thermostatted cell compartment (0.05˚C). Values of k1 were calculated from the stan- dard equation using a least-squares procedure. All kinetic mea- surements were duplicated, and the average devia- tion from the mean was 3%. Second-order rate con- stants (k2) were calculated from the slope of the plots of pseudo-first-order rate constants versus nucleophile con- centrations (at least three different concentrations). 2exp exp kT HS khRT R (1) where k, is Boltzman’s constant, h, Planck’s constant and the other symbols have their usual meanings. Expressing Equation (1) in logarithmic form, Equation (2) is ob- tained. From a plot of Ink2 versus 1/T, ΔS≠ and ΔH≠ can Copyright © 2011 SciRes. IJOC
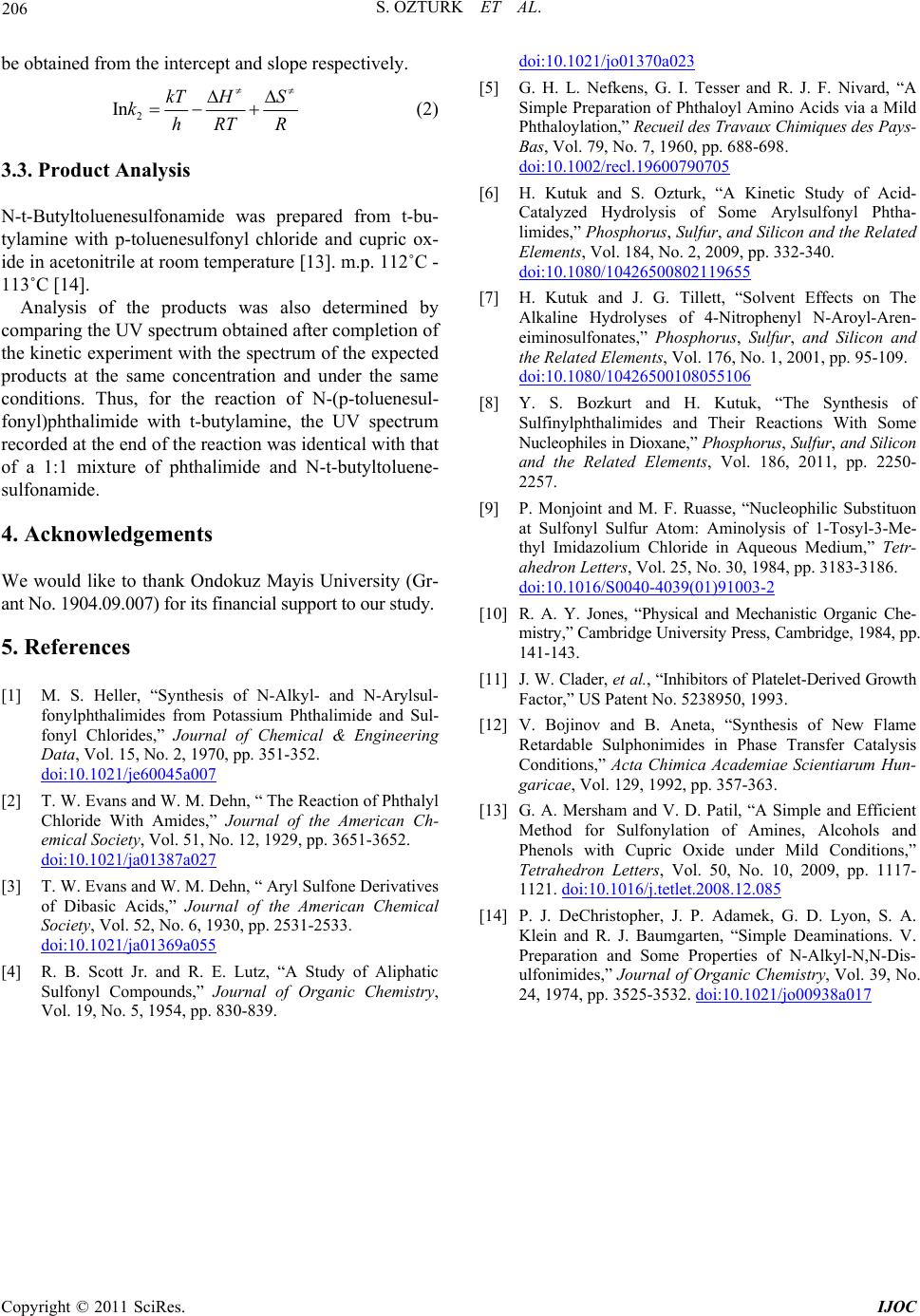 S. OZTURK ET AL. 206 be obtained from the intercept and slope respectively. 2 In kT HS khRT R (2) 3.3. Product Analysis N-t-Butyltoluenesulfonamide was prepared from t-bu- tylamine with p-toluenesulfonyl chloride and cupric ox- ide in acetonitrile at room temperature [13]. m.p. 112˚C - 113˚C [14]. Analysis of the products was also determined by comparing the UV spectrum obtained after completion of the kinetic experiment with the spectrum of the expected products at the same concentration and under the same conditions. Thus, for the reaction of N-(p-toluenesul- fonyl)phthalimide with t-butylamine, the UV spectrum recorded at the end of the reaction was identical with that of a 1:1 mixture of phthalimide and N-t-butyltoluene- sulfonamide. 4. Acknowledgements We would like to thank Ondokuz Mayis University (Gr- ant No. 1904.09.007) for its financial support to our study. 5. References [1] M. S. Heller, “Synthesis of N-Alkyl- and N-Arylsul- fonylphthalimides from Potassium Phthalimide and Sul- fonyl Chlorides,” Journal of Chemical & Engineering Data, Vol. 15, No. 2, 1970, pp. 351-352. doi:10.1021/je60045a007 [2] T. W. Evans and W. M. Dehn, “ The Reaction of Phthalyl Chloride With Amides,” Journal of the American Ch- emical Society, Vol. 51, No. 12, 1929, pp. 3651-3652. doi:10.1021/ja01387a027 [3] T. W. Evans and W. M. Dehn, “ Aryl Sulfone Derivatives of Dibasic Acids,” Journal of the American Chemical Society, Vol. 52, No. 6, 1930, pp. 2531-2533. doi:10.1021/ja01369a055 [4] R. B. Scott Jr. and R. E. Lutz, “A Study of Aliphatic Sulfonyl Compounds,” Journal of Organic Chemistry, Vol. 19, No. 5, 1954, pp. 830-839. doi:10.1021/jo01370a023 [5] G. H. L. Nefkens, G. I. Tesser and R. J. F. Nivard, “A Simple Preparation of Phthaloyl Amino Acids via a Mild Phthaloylation,” Recueil des Trav aux Chimiques de s Pays- Bas, Vol. 79, No. 7, 1960, pp. 688-698. doi:10.1002/recl.19600790705 [6] H. Kutuk and S. Ozturk, “A Kinetic Study of Acid- Catalyzed Hydrolysis of Some Arylsulfonyl Phtha- limides,” Phosphorus, Sulfur, and Silicon and the Related Elements, Vol. 184, No. 2, 2009, pp. 332-340. doi:10.1080/10426500802119655 [7] H. Kutuk and J. G. Tillett, “Solvent Effects on The Alkaline Hydrolyses of 4-Nitrophenyl N-Aroyl-Aren- eiminosulfonates,” Phosphorus, Sulfur, and Silicon and the Related Elements , Vol. 176, No. 1, 2001, pp. 95-109. doi:10.1080/10426500108055106 [8] Y. S. Bozkurt and H. Kutuk, “The Synthesis of Sulfinylphthalimides and Their Reactions With Some Nucleophiles in Dioxane,” Phosphorus, Sulfur, and Silicon and the Related Elements, Vol. 186, 2011, pp. 2250- 2257. [9] P. Monjoint and M. F. Ruasse, “Nucleophilic Substituon at Sulfonyl Sulfur Atom: Aminolysis of 1-Tosyl-3-Me- thyl Imidazolium Chloride in Aqueous Medium,” Tetr- ahedron Letters, Vol. 25, No. 30, 1984, pp. 3183-3186. doi:10.1016/S0040-4039(01)91003-2 [10] R. A. Y. Jones, “Physical and Mechanistic Organic Che- mistry,” Cambridge University Press, Cambridge, 1984, pp. 141-143. [11] J. W. Clader, et al., “Inhibitors of Platelet-Derived Growth Factor,” US Patent No. 5238950, 1993. [12] V. Bojinov and B. Aneta, “Synthesis of New Flame Retardable Sulphonimides in Phase Transfer Catalysis Conditions,” Acta Chimica Academiae Scientiarum Hun- garicae, Vol. 129, 1992, pp. 357-363. [13] G. A. Mersham and V. D. Patil, “A Simple and Efficient Method for Sulfonylation of Amines, Alcohols and Phenols with Cupric Oxide under Mild Conditions,” Tetrahedron Letters, Vol. 50, No. 10, 2009, pp. 1117- 1121. doi:10.1016/j.tetlet.2008.12.085 [14] P. J. DeChristopher, J. P. Adamek, G. D. Lyon, S. A. Klein and R. J. Baumgarten, “Simple Deaminations. V. Preparation and Some Properties of N-Alkyl-N,N-Dis- ulfonimides,” Journal of Organic Chemistry, Vol. 39, No. 24, 1974, pp. 3525-3532. doi:10.1021/jo00938a017 Copyright © 2011 SciRes. IJOC
|