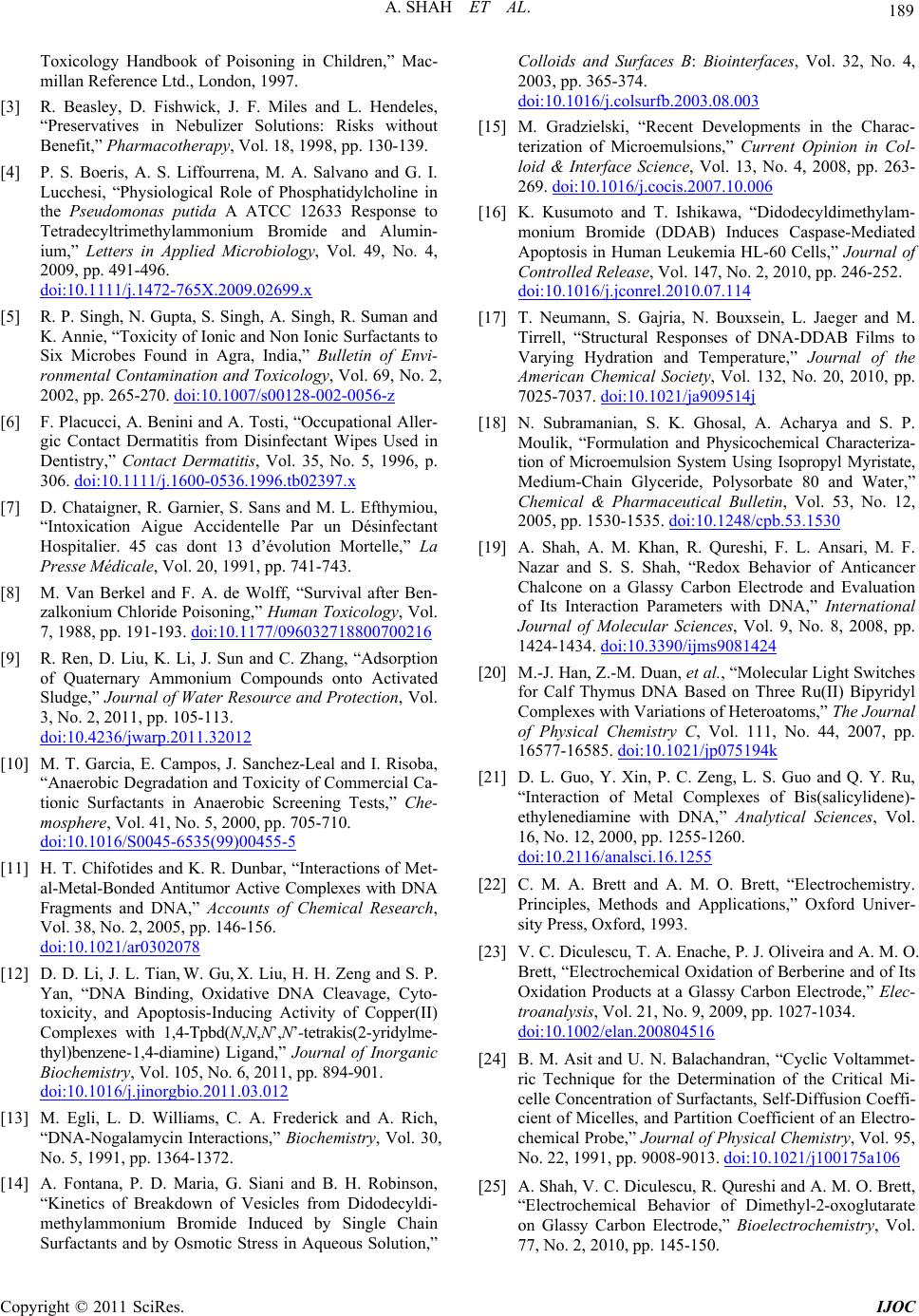
189
A. SHAH ET AL.
Toxicology Handbook of Poisoning in Children,” Mac-
millan Reference Ltd., London, 1997.
[3] R. Beasley, D. Fishwick, J. F. Miles and L. Hendeles,
“Preservatives in Nebulizer Solutions: Risks without
Benefit,” Pharmacotherapy, Vol. 18, 1998, pp. 130-139.
[4] P. S. Boeris, A. S. Liffourrena, M. A. Salvano and G. I.
Lucchesi, “Physiological Role of Phosphatidylcholine in
the Pseudomonas putida A ATCC 12633 Response to
Tetradecyltrimethylammonium Bromide and Alumin-
ium,” Letters in Applied Microbiology, Vol. 49, No. 4,
2009, pp. 491-496.
doi:10.1111/j.1472-765X.2009.02699.x
[5] R. P. Singh, N. Gupta, S. Singh, A. Singh, R. Suman and
K. Annie, “Toxicity of Ionic and Non Ionic Surfactants to
Six Microbes Found in Agra, India,” Bulletin of Envi-
ronmental Contamination and Toxicology, Vol. 69, No. 2,
2002, pp. 265-270. doi:10.1007/s00128-002-0056-z
[6] F. Placucci, A. Benini and A. Tosti, “Occupational Aller-
gic Contact Dermatitis from Disinfectant Wipes Used in
Dentistry,” Contact Dermatitis, Vol. 35, No. 5, 1996, p.
306. doi:10.1111/j.1600-0536.1996.tb02397.x
[7] D. Chataigner, R. Garnier, S. Sans and M. L. Efthymiou,
“Intoxication Aigue Accidentelle Par un Désinfectant
Hospitalier. 45 cas dont 13 d’évolution Mortelle,” La
Presse Médicale, Vol. 20, 1991, pp. 741-743.
[8] M. Van Berkel and F. A. de Wolff, “Survival after Ben-
zalkonium Chloride Poisoning,” Human Toxicology, Vol.
7, 1988, pp. 191-193. doi:10.1177/096032718800700216
[9] R. Ren, D. Liu, K. Li, J. Sun and C. Zhang, “Adsorption
of Quaternary Ammonium Compounds onto Activated
Sludge,” Journal of Water Resource and Protection, Vol.
3, No. 2, 2011, pp. 105-113.
doi:10.4236/jwarp.2011.32012
[10] M. T. Garcia, E. Campos, J. Sanchez-Leal and I. Risoba,
“Anaerobic Degradation and Toxicity of Commercial Ca-
tionic Surfactants in Anaerobic Screening Tests,” Che-
mosphere, Vol. 41, No. 5, 2000, pp. 705-710.
doi:10.1016/S0045-6535(99)00455-5
[11] H. T. Chifotides and K. R. Dunbar, “Interactions of Met-
al-Metal-Bonded Antitumor Active Complexes with DNA
Fragments and DNA,” Accounts of Chemical Research,
Vol. 38, No. 2, 2005, pp. 146-156.
doi:10.1021/ar0302078
[12] D. D. Li, J. L. Tian, W. Gu, X. Liu, H. H. Zeng and S. P.
Yan, “DNA Binding, Oxidative DNA Cleavage, Cyto-
toxicity, and Apoptosis-Inducing Activity of Copper(II)
Complexes with 1,4-Tpbd(N,N,N’,N’-tetrakis(2-yridylme-
thyl)benzene-1,4-diamine) Ligand,” Journal of Inorganic
Biochemistry, Vol. 105, No. 6, 2011, pp. 894-901.
doi:10.1016/j.jinorgbio.2011.03.012
[13] M. Egli, L. D. Williams, C. A. Frederick and A. Rich,
“DNA-Nogalamycin Interactions,” Biochemistry, Vol. 30,
No. 5, 1991, pp. 1364-1372.
[14] A. Fontana, P. D. Maria, G. Siani and B. H. Robinson,
“Kinetics of Breakdown of Vesicles from Didodecyldi-
methylammonium Bromide Induced by Single Chain
Surfactants and by Osmotic Stress in Aqueous Solution,”
Colloids and Surfaces B: Biointerfaces, Vol. 32, No. 4,
2003, pp. 365-374.
doi:10.1016/j.colsurfb.2003.08.003
[15] M. Gradzielski, “Recent Developments in the Charac-
terization of Microemulsions,” Current Opinion in Col-
loid & Interface Science, Vol. 13, No. 4, 2008, pp. 263-
269. doi:10.1016/j.cocis.2007.10.006
[16] K. Kusumoto and T. Ishikawa, “Didodecyldimethylam-
monium Bromide (DDAB) Induces Caspase-Mediated
Apoptosis in Human Leukemia HL-60 Cells,” Journal of
Controlled Release, Vol. 147, No. 2, 2010, pp. 246-252.
doi:10.1016/j.jconrel.2010.07.114
[17] T. Neumann, S. Gajria, N. Bouxsein, L. Jaeger and M.
Tirrell, “Structural Responses of DNA-DDAB Films to
Varying Hydration and Temperature,” Journal of the
American Chemical Society, Vol. 132, No. 20, 2010, pp.
7025-7037. doi:10.1021/ja909514j
[18] N. Subramanian, S. K. Ghosal, A. Acharya and S. P.
Moulik, “Formulation and Physicochemical Characteriza-
tion of Microemulsion System Using Isopropyl Myristate,
Medium-Chain Glyceride, Polysorbate 80 and Water,”
Chemical & Pharmaceutical Bulletin, Vol. 53, No. 12,
2005, pp. 1530-1535. doi:10.1248/cpb.53.1530
[19] A. Shah, A. M. Khan, R. Qureshi, F. L. Ansari, M. F.
Nazar and S. S. Shah, “Redox Behavior of Anticancer
Chalcone on a Glassy Carbon Electrode and Evaluation
of Its Interaction Parameters with DNA,” International
Journal of Molecular Sciences, Vol. 9, No. 8, 2008, pp.
1424-1434. doi:10.3390/ijms9081424
[20] M.-J. Han, Z.-M. Duan, et al., “Molecular Light Switches
for Calf Thymus DNA Based on Three Ru(II) Bipyridyl
Complexes with Variations of Heteroatoms,” The Journal
of Physical Chemistry C, Vol. 111, No. 44, 2007, pp.
16577-16585. doi:10.1021/jp075194k
[21] D. L. Guo, Y. Xin, P. C. Zeng, L. S. Guo and Q. Y. Ru,
“Interaction of Metal Complexes of Bis(salicylidene)-
ethylenediamine with DNA,” Analytical Sciences, Vol.
16, No. 12, 2000, pp. 1255-1260.
doi:10.2116/analsci.16.1255
[22] C. M. A. Brett and A. M. O. Brett, “Electrochemistry.
Principles, Methods and Applications,” Oxford Univer-
sity Press, Oxford, 1993.
[23] V. C. Diculescu, T. A. Enache, P. J. Oliveira and A. M. O.
Brett, “Electrochemical Oxidation of Berberine and of Its
Oxidation Products at a Glassy Carbon Electrode,” Elec-
troanalysis, Vol. 21, No. 9, 2009, pp. 1027-1034.
doi:10.1002/elan.200804516
[24] B. M. Asit and U. N. Balachandran, “Cyclic Voltammet-
ric Technique for the Determination of the Critical Mi-
celle Concentration of Surfactants, Self-Diffusion Coeffi-
cient of Micelles, and Partition Coefficient of an Electro-
chemical Probe,” Journal of Physical Chemistry, Vol. 95,
No. 22, 1991, pp. 9008-9013. doi:10.1021/j100175a106
[25] A. Shah, V. C. Diculescu, R. Qureshi and A. M. O. Brett,
“Electrochemical Behavior of Dimethyl-2-oxoglutarate
on Glassy Carbon Electrode,” Bioelectrochemistry, Vol.
77, No. 2, 2010, pp. 145-150.
Copyright © 2011 SciRes. IJOC