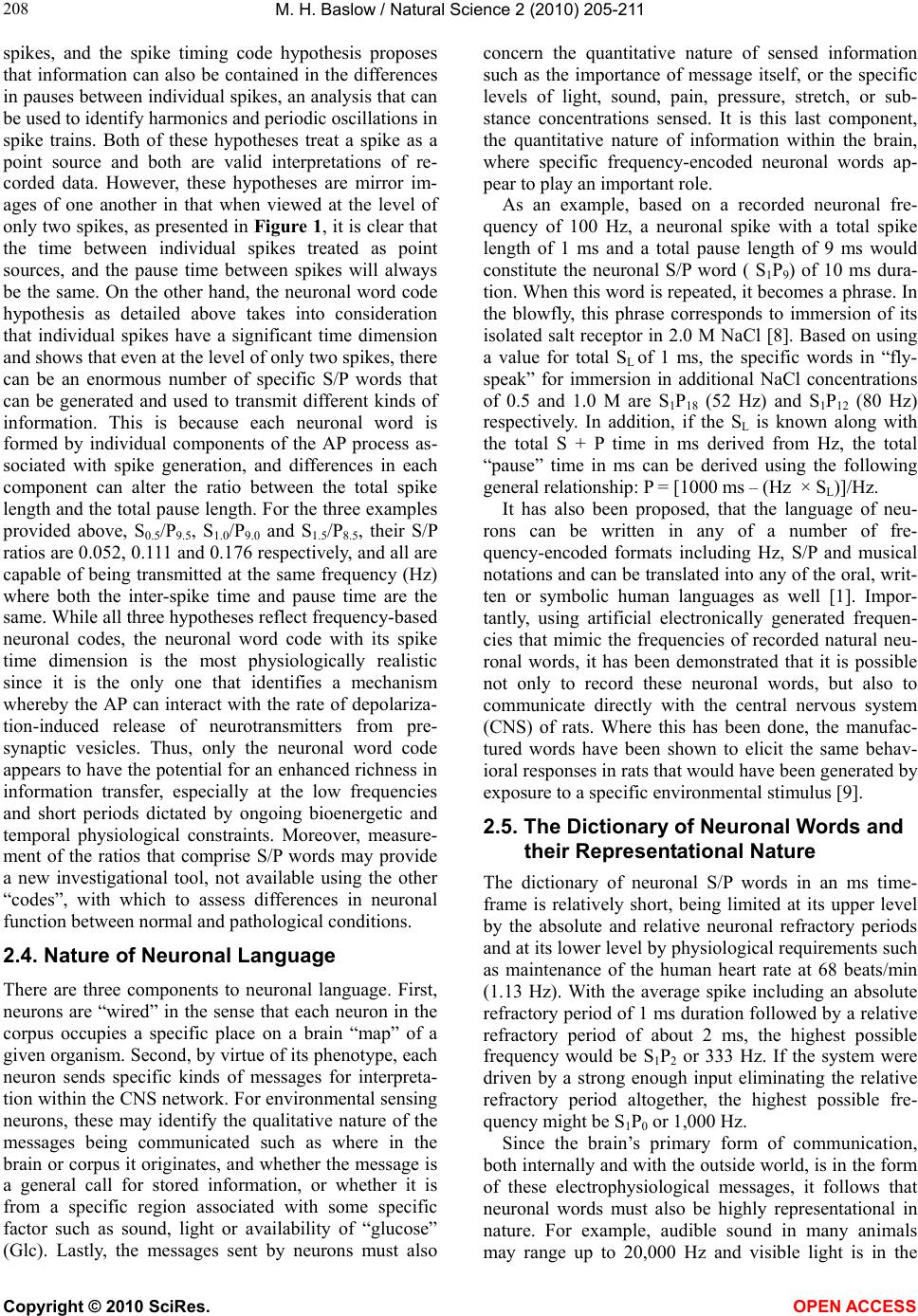
M. H. Baslow / Natural Science 2 (2010) 205-211
Copyright © 2010 SciRes. OPEN ACCESS
208
spikes, and the spike timing code hypothesis proposes
that information can also be contained in the differences
in pauses between individual spikes, an analysis that can
be used to identify harmonics and periodic oscillations in
spike trains. Both of these hypotheses treat a spike as a
point source and both are valid interpretations of re-
corded data. However, these hypotheses are mirror im-
ages of one another in that when viewed at the level of
only two spikes, as presented in Figure 1, it is clear that
the time between individual spikes treated as point
sources, and the pause time between spikes will always
be the same. On the other hand, the neuronal word code
hypothesis as detailed above takes into consideration
that individual spikes have a significant time dimension
and shows that even at the level of only two spikes, there
can be an enormous number of specific S/P words that
can be generated and used to transmit different kinds of
information. This is because each neuronal word is
formed by individual components of the AP process as-
sociated with spike generation, and differences in each
component can alter the ratio between the total spike
length and the total pause length. For the three examples
provided above, S0.5/P 9.5, S1.0/P9.0 and S1.5/P8.5, their S/P
ratios are 0.052, 0.111 and 0.176 respectively, and all are
capable of being transmitted at the same frequency (Hz)
where both the inter-spike time and pause time are the
same. While all three hypotheses reflect frequency-based
neuronal codes, the neuronal word code with its spike
time dimension is the most physiologically realistic
since it is the only one that identifies a mechanism
whereby the AP can interact with the rate of depolariza-
tion-induced release of neurotransmitters from pre-
synaptic vesicles. Thus, only the neuronal word code
appears to have the potential for an enhanced richness in
information transfer, especially at the low frequencies
and short periods dictated by ongoing bioenergetic and
temporal physiological constraints. Moreover, measure-
ment of the ratios that comprise S/P words may provide
a new investigational tool, not available using the other
“codes”, with which to assess differences in neuronal
function between normal and pathological conditions.
2.4. Nature of Neuronal Language
There are three components to neuronal language. First,
neurons are “wired” in the sense that each neuron in the
corpus occupies a specific place on a brain “map” of a
given organism. Second, by virtue of its phenotype, each
neuron sends specific kinds of messages for interpreta-
tion within the CNS network. For environmental sensing
neurons, these may identify the qualitative nature of the
messages being communicated such as where in the
brain or corpus it originates, and whether the message is
a general call for stored information, or whether it is
from a specific region associated with some specific
factor such as sound, light or availability of “glucose”
(Glc). Lastly, the messages sent by neurons must also
concern the quantitative nature of sensed information
such as the importance of message itself, or the specific
levels of light, sound, pain, pressure, stretch, or sub-
stance concentrations sensed. It is this last component,
the quantitative nature of information within the brain,
where specific frequency-encoded neuronal words ap-
pear to play an important role.
As an example, based on a recorded neuronal fre-
quency of 100 Hz, a neuronal spike with a total spike
length of 1 ms and a total pause length of 9 ms would
constitute the neuronal S/P word ( S1P9) of 10 ms dura-
tion. When this word is repeated, it becomes a phrase. In
the blowfly, this phrase corresponds to immersion of its
isolated salt receptor in 2.0 M NaCl [8]. Based on using
a value for total SL of 1 ms, the specific words in “fly-
speak” for immersion in additional NaCl concentrations
of 0.5 and 1.0 M are S1P18 (52 Hz) and S1P12 (80 Hz)
respectively. In addition, if the SL is known along with
the total S + P time in ms derived from Hz, the total
“pause” time in ms can be derived using the following
general relationship: P = [1000 ms – (Hz × SL)]/Hz.
It has also been proposed, that the language of neu-
rons can be written in any of a number of fre-
quency-encoded formats including Hz, S/P and musical
notations and can be translated into any of the oral, writ-
ten or symbolic human languages as well [1]. Impor-
tantly, using artificial electronically generated frequen-
cies that mimic the frequencies of recorded natural neu-
ronal words, it has been demonstrated that it is possible
not only to record these neuronal words, but also to
communicate directly with the central nervous system
(CNS) of rats. Where this has been done, the manufac-
tured words have been shown to elicit the same behav-
ioral responses in rats that would have been generated by
exposure to a specific environmental stimulus [9].
2.5. The Dictionary of Neuronal Words and
their Representational Nature
The dictionary of neuronal S/P words in an ms time-
frame is relatively short, being limited at its upper level
by the absolute and relative neuronal refractory periods
and at its lower level by physiological requirements such
as maintenance of the human heart rate at 68 beats/min
(1.13 Hz). With the average spike including an absolute
refractory period of 1 ms duration followed by a relative
refractory period of about 2 ms, the highest possible
frequency would be S1P2 or 333 Hz. If the system were
driven by a strong enough input eliminating the relative
refractory period altogether, the highest possible fre-
quency might be S1P0 or 1,000 Hz.
Since the brain’s primary form of communication,
both internally and with the outside world, is in the form
of these electrophysiological messages, it follows that
neuronal words must also be highly representational in
nature. For example, audible sound in many animals
may range up to 20,000 Hz and visible light is in the