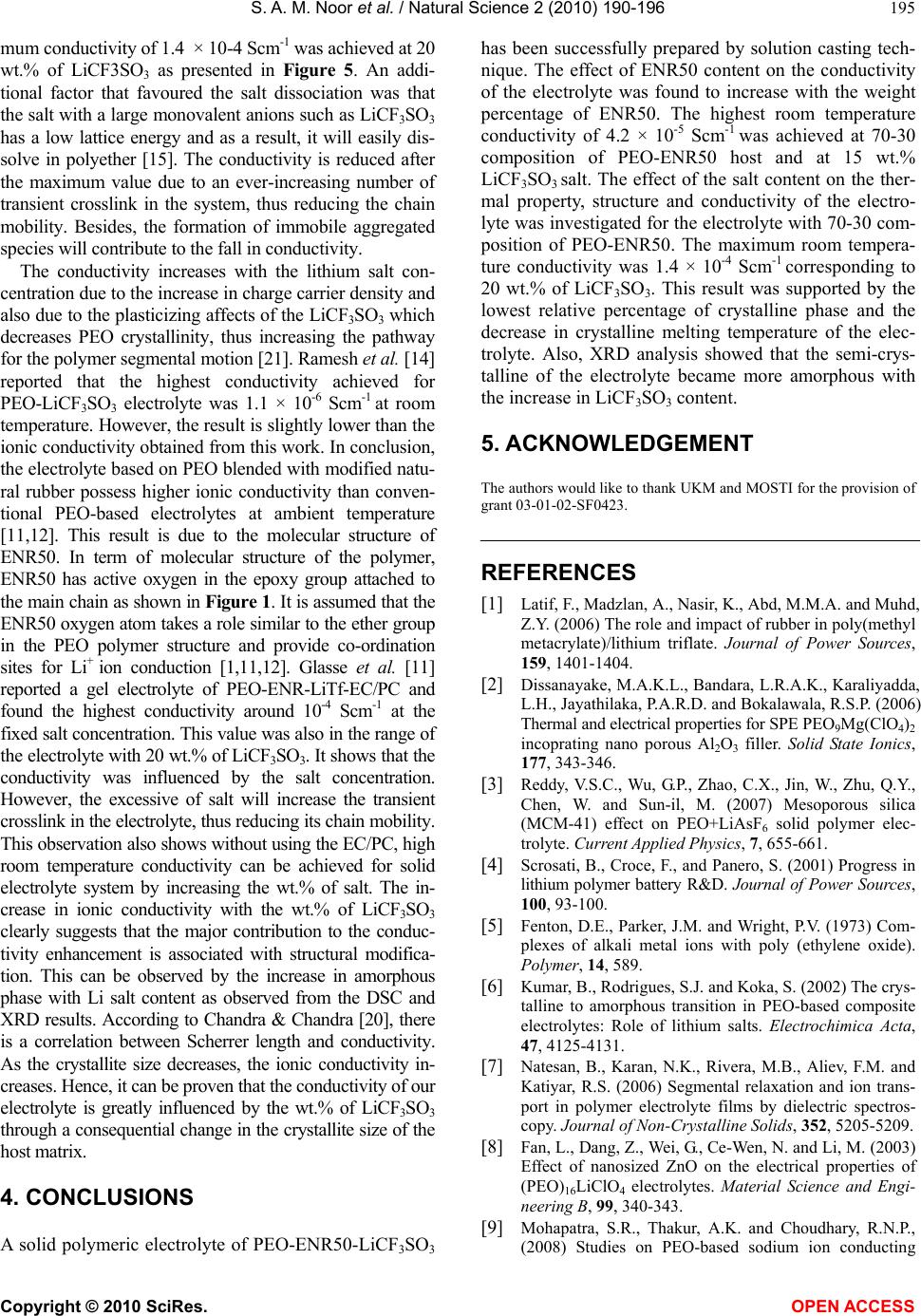
S. A. M. Noor et al. / Natural Science 2 (2010) 190-196
Copyright © 2010 SciRes. OPEN ACCESS
195
mum conductivity of 1.4 × 10-4 Scm-1 was achieved at 20
wt.% of LiCF3SO3 as presented in Figure 5. An addi-
tional factor that favoured the salt dissociation was that
the salt with a large monovalent anions such as LiCF3SO3
has a low lattice energy and as a result, it will easily dis-
solve in polyether [15]. The conductivity is reduced after
the maximum value due to an ever-increasing number of
transient crosslink in the system, thus reducing the chain
mobility. Besides, the formation of immobile aggregated
species will contribute to the fall in conductivity.
The conductivity increases with the lithium salt con-
centration due to the increase in charge carrier density and
also due to the plasticizing affects of the LiCF3SO3 which
decreases PEO crystallinity, thus increasing the pathway
for the polymer segmental motion [21]. Ramesh et al. [14]
reported that the highest conductivity achieved for
PEO-LiCF3SO3 electrolyte was 1.1 × 10-6 Scm-1 at room
temperature. However, the result is slightly lower than the
ionic conductivity obtained from this work. In conclusion,
the electrolyte based on PEO blended with modified natu-
ral rubber possess higher ionic conductivity than conven-
tional PEO-based electrolytes at ambient temperature
[11,12]. This result is due to the molecular structure of
ENR50. In term of molecular structure of the polymer,
ENR50 has active oxygen in the epoxy group attached to
the main chain as shown in Figure 1. It is assumed that the
ENR50 oxygen atom takes a role similar to the ether group
in the PEO polymer structure and provide co-ordination
sites for Li+ ion conduction [1,11,12]. Glasse et al. [11]
reported a gel electrolyte of PEO-ENR-LiTf-EC/PC and
found the highest conductivity around 10-4 Scm-1 at the
fixed salt concentration. This value was also in the range of
the electrolyte with 20 wt.% of LiCF3SO3. It shows that the
conductivity was influenced by the salt concentration.
However, the excessive of salt will increase the transient
crosslink in the electrolyte, thus reducing its chain mobility.
This observation also shows without using the EC/PC, high
room temperature conductivity can be achieved for solid
electrolyte system by increasing the wt.% of salt. The in-
crease in ionic conductivity with the wt.% of LiCF3SO3
clearly suggests that the major contribution to the conduc-
tivity enhancement is associated with structural modifica-
tion. This can be observed by the increase in amorphous
phase with Li salt content as observed from the DSC and
XRD results. According to Chandra & Chandra [20], there
is a correlation between Scherrer length and conductivity.
As the crystallite size decreases, the ionic conductivity in-
creases. Hence, it can be proven that the conductivity of our
electrolyte is greatly influenced by the wt.% of LiCF3SO3
through a consequential change in the crystallite size of the
host matrix.
4. CONCLUSIONS
A solid polymeric electrolyte of PEO-ENR50-LiCF3SO 3
has been successfully prepared by solution casting tech-
nique. The effect of ENR50 content on the conductivity
of the electrolyte was found to increase with the weight
percentage of ENR50. The highest room temperature
conductivity of 4.2 × 10-5 Scm-1 was achieved at 70-30
composition of PEO-ENR50 host and at 15 wt.%
LiCF3SO3 salt. The effect of the salt content on the ther-
mal property, structure and conductivity of the electro-
lyte was investigated for the electrolyte with 70-30 com-
position of PEO-ENR50. The maximum room tempera-
ture conductivity was 1.4 × 10-4 Scm-1 corresponding to
20 wt.% of LiCF3SO3. This result was supported by the
lowest relative percentage of crystalline phase and the
decrease in crystalline melting temperature of the elec-
trolyte. Also, XRD analysis showed that the semi-crys-
talline of the electrolyte became more amorphous with
the increase in LiCF3SO3 content.
5. ACKNOWLEDGEMENT
The authors would like to thank UKM and MOSTI for the provision of
grant 03-01-02-SF0423.
REFERENCES
[1] Latif, F., Madzlan, A., Nasir, K., Abd, M.M.A. and Muhd,
Z.Y. (2006) The role and impact of rubber in poly(methyl
metacrylate)/lithium triflate. Journal of Power Sources,
159, 1401-1404.
[2] Dissanayake, M.A.K.L., Bandara, L.R.A.K., Karaliyadda,
L.H., Jayathilaka, P.A.R.D. and Bokalawala, R.S.P. (2006)
Thermal and electrical properties for SPE PEO9Mg(ClO4)2
incoprating nano porous Al2O3 filler. Solid State Ionics,
177, 343-346.
[3] Reddy, V.S.C., Wu, G.P., Zhao, C.X., Jin, W., Zhu, Q.Y.,
Chen, W. and Sun-il, M. (2007) Mesoporous silica
(MCM-41) effect on PEO+LiAsF6 solid polymer elec-
trolyte. Current Applied Physics, 7, 655-661.
[4] Scrosati, B., Croce, F., and Panero, S. (2001) Progress in
lithium polymer battery R&D. Journal of Power Sources,
100, 93-100.
[5] Fenton, D.E., Parker, J.M. and Wright, P.V. (1973) Com-
plexes of alkali metal ions with poly (ethylene oxide).
Polymer, 14, 589.
[6] Kumar, B., Rodrigues, S.J. and Koka, S. (2002) The crys-
talline to amorphous transition in PEO-based composite
electrolytes: Role of lithium salts. Electrochimica Acta,
47, 4125-4131.
[7] Natesan, B., Karan, N.K., Rivera, M.B., Aliev, F.M. and
Katiyar, R.S. (2006) Segmental relaxation and ion trans-
port in polymer electrolyte films by dielectric spectros-
copy. Journal of Non-Crystalline Solids, 352, 5205-5209.
[8] Fan, L., Dang, Z., Wei, G., Ce-Wen, N. and Li, M. (2003)
Effect of nanosized ZnO on the electrical properties of
(PEO)16LiClO4 electrolytes. Material Science and Engi-
neering B, 99, 340-343.
[9] Mohapatra, S.R., Thakur, A.K. and Choudhary, R.N.P.,
(2008) Studies on PEO-based sodium ion conducting