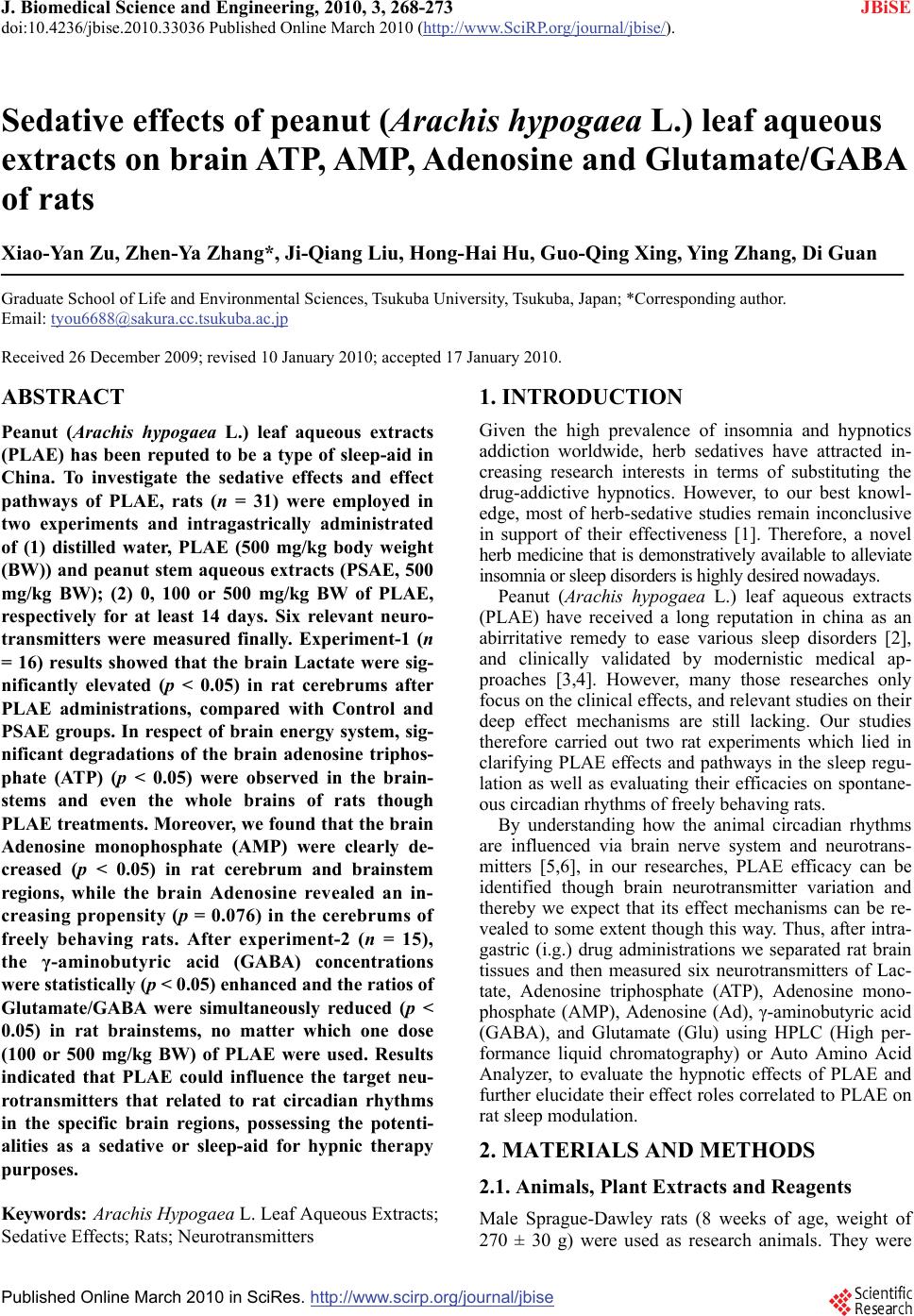 J. Biomedical Science and Engineering, 2010, 3, 268-273 doi:10.4236/jbise.2010.33036 Published Online March 2010 (http://www.SciRP.org/journal/jbise/ JBiSE ). Published Online March 2010 in SciRes. http://www.scirp.org/journal/jbise Sedative effects of peanut (Arachis hypogaea L.) leaf aqueous extracts on brain ATP, AMP, Adenosine and Glutamate/GABA of rats Xiao-Yan Zu, Zhen-Ya Zhang*, Ji-Qiang Liu, Hong-Hai Hu, Guo-Qing Xing, Ying Zhang, Di Guan Graduate School of Life and Environmental Sciences, Tsukuba University, Tsukuba, Japan; *Corresponding author. Email: tyou6688@sakura.cc.tsukuba.ac.jp Received 26 December 2009; revised 10 January 2010; accepted 17 January 2010. ABSTRACT Peanut (Arachis hypogaea L.) leaf aqueous extracts (PLAE) has been reputed to be a type of sleep-aid in China. To investigate the sedative effects and effect pathways of PLAE, rats (n = 31) were employed in two experiments and intragastrically administrated of (1) distilled water, PLAE (500 mg/kg body weight (BW)) and peanut stem aqueous extracts (PSAE, 500 mg/kg BW); (2) 0, 100 or 500 mg/kg BW of PLAE, respectively for at least 14 days. Six relevant neuro- transmitters were measured finally. Experiment-1 (n = 16) results showed that the brain Lactate were sig- nificantly elevated (p < 0.05) in rat cerebrums after PLAE administrations, compared with Control and PSAE groups. In respect of brain energy system, sig- nificant degradations of the brain adenosine triphos- phate (ATP) (p < 0.05) were observed in the brain- stems and even the whole brains of rats though PLAE treatments. Moreover, we found that the brain Adenosine monophosphate (AMP) were clearly de- creased (p < 0.05) in rat cerebrum and brainstem regions, while the brain Adenosine revealed an in- creasing propensity (p = 0.076) in the cerebrums of freely behaving rats. After experiment-2 (n = 15), the γ-aminobutyric acid (GABA) concentrations were statistically (p < 0.05) enhanced and the ratios of Glutamate/GABA were simultaneously reduced (p < 0.05) in rat brainstems, no matter which one dose (100 or 500 mg/kg BW) of PLAE were used. Results indicated that PLAE could influence the target neu- rotransmitters that related to rat circadian rhythms in the specific brain regions, possessing the potenti- alities as a sedative or sleep-aid for hypnic therapy purposes. Keywords: Arachis Hypogaea L. Leaf Aqueous Extracts; Sedative Effects; Rats; Neurotransmitters 1. INTRODUCTION Given the high prevalence of insomnia and hypnotics addiction worldwide, herb sedatives have attracted in- creasing research interests in terms of substituting the drug-addictive hypnotics. However, to our best knowl- edge, most of herb-sedative studies remain inconclusive in support of their effectiveness [1]. Therefore, a novel herb medicine that is demonstratively available to alleviate insomnia or sleep disorders is highly desired nowadays. Peanut (Arachis hypogaea L.) leaf aqueous extracts (PLAE) have received a long reputation in china as an abirritative remedy to ease various sleep disorders [2], and clinically validated by modernistic medical ap- proaches [3,4]. However, many those researches only focus on the clinical effects, and relevant studies on their deep effect mechanisms are still lacking. Our studies therefore carried out two rat experiments which lied in clarifying PLAE effects and pathways in the sleep regu- lation as well as evaluating their efficacies on spontane- ous circadian rhythms of freely behaving rats. By understanding how the animal circadian rhythms are influenced via brain nerve system and neurotrans- mitters [5,6], in our researches, PLAE efficacy can be identified though brain neurotransmitter variation and thereby we expect that its effect mechanisms can be re- vealed to some extent though this way. Thus, after intr a- gastric (i.g.) drug administrations we separated rat brain tissues and then measured six neurotransmitters of Lac- tate, Adenosine triphosphate (ATP), Adenosine mono- phosphate (AMP), Adenosine (Ad), γ-aminobutyric acid (GABA), and Glutamate (Glu) using HPLC (High per- formance liquid chromatography) or Auto Amino Acid Analyzer, to evaluate the hypnotic effects of PLAE and further elucidate their effect roles correlated to PLAE on rat sleep modulation. 2. MATERIALS AND METHODS 2.1. Animals, Plant Extracts and Reagents Male Sprague-Dawley rats (8 weeks of age, weight of 270 ± 30 g) were used as research animals. They were
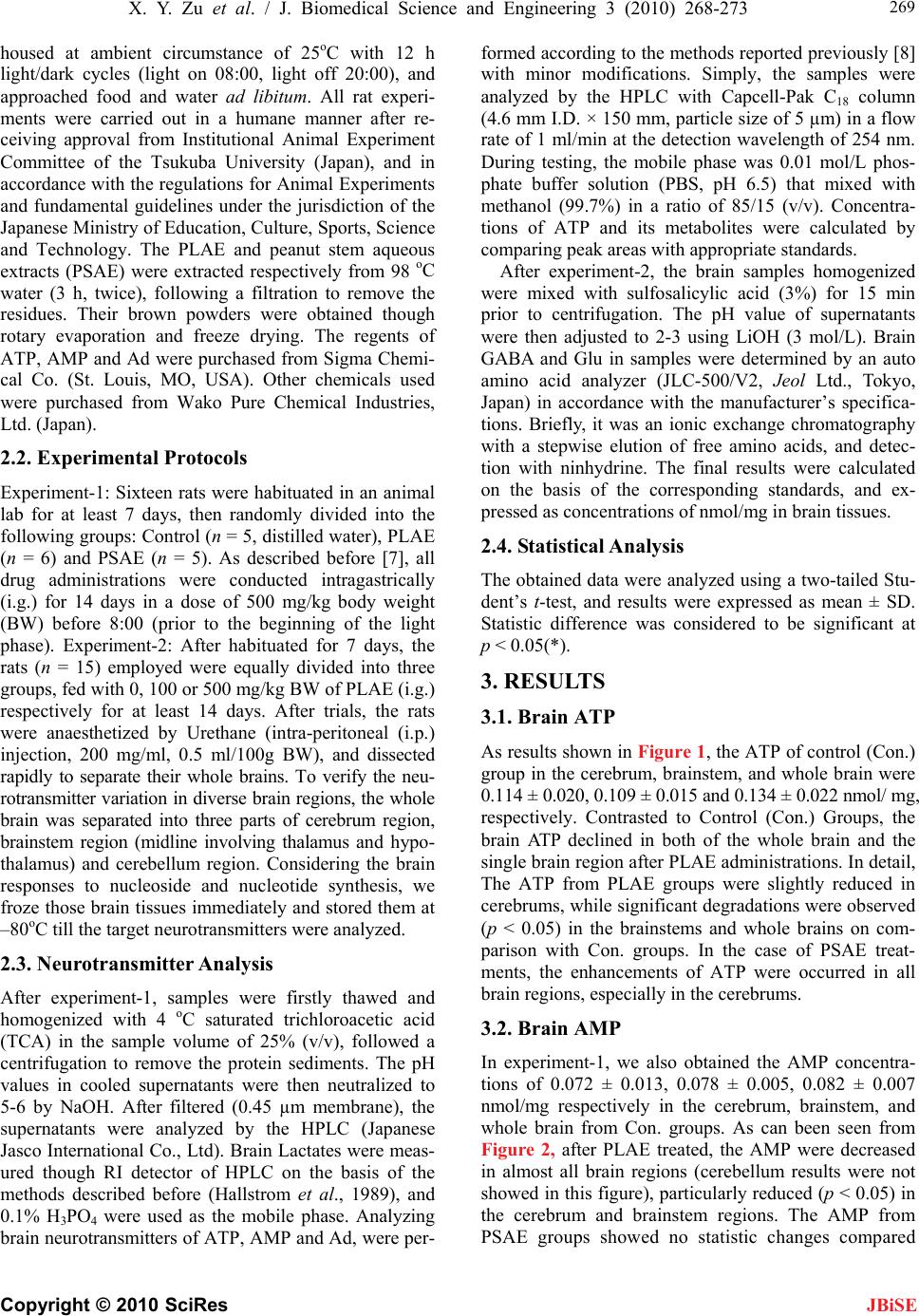 X. Y. Zu et al. / J. Biomedical Science and Engineering 3 (2010) 268-273 Copyright © 2010 SciRes 269 JBiSE housed at ambient circumstance of 25oC with 12 h light/dark cycles (light on 08:00, light off 20:00), and approached food and water ad libitum. All rat experi- ments were carried out in a humane manner after re- ceiving approval from Institutional Animal Experiment Committee of the Tsukuba University (Japan), and in accordance with the regulations for Animal Experiments and fundamental guidelines under the jurisdiction of the Japanese Ministry of Education, Culture, Sports, Science and Technology. The PLAE and peanut stem aqueous extracts (PSAE) were extracted respectively from 98 oC water (3 h, twice), following a filtration to remove the residues. Their brown powders were obtained though rotary evaporation and freeze drying. The regents of ATP, AMP and Ad were purchased from Sigma Chemi- cal Co. (St. Louis, MO, USA). Other chemicals used were purchased from Wako Pure Chemical Industries, Ltd. (Japan). 2.2. Experimental Protocols Experiment-1: Sixteen rats were habituated in an animal lab for at least 7 days, then randomly divided into the following groups: Control (n = 5, distilled water), PLAE (n = 6) and PSAE (n = 5). As described before [7], all drug administrations were conducted intragastrically (i.g.) for 14 days in a dose of 500 mg/kg body weight (BW) before 8:00 (prior to the beginning of the light phase). Experiment-2: After habituated for 7 days, the rats (n = 15) employed were equally divided into three groups, fed with 0, 100 or 500 mg/kg BW of PLAE (i.g.) respectively for at least 14 days. After trials, the rats were anaesthetized by Urethane (intra-peritoneal (i.p.) injection, 200 mg/ml, 0.5 ml/100g BW), and dissected rapidly to separate their whole brains. To verify the neu- rotransmitter variation in diverse brain regions, the whole brain was separated into three parts of cerebrum region, brainstem region (midline involving thalamus and hypo- thalamus) and cerebellum region. Considering the brain responses to nucleoside and nucleotide synthesis, we froze those brain tissues immediately and stored them at –80oC till the target neurotransmitters were analyzed. 2.3. Neurotransmitter Analysis After experiment-1, samples were firstly thawed and homogenized with 4 oC saturated trichloroacetic acid (TCA) in the sample volume of 25% (v/v), followed a centrifugation to remove the protein sediments. The pH values in cooled supernatants were then neutralized to 5-6 by NaOH. After filtered (0.45 µm membrane), the supernatants were analyzed by the HPLC (Japanese Jasco International Co., Ltd). Brain Lactates were meas- ured though RI detector of HPLC on the basis of the methods described before (Hallstrom et al., 1989), and 0.1% H3PO4 were used as the mobile phase. Analyzing brain neurotransmitters of ATP, AMP and Ad, were per- formed according to the methods reported previously [8] with minor modifications. Simply, the samples were analyzed by the HPLC with Capcell-Pak C18 column (4.6 mm I.D. × 150 mm, particle size of 5 µm) in a flow rate of 1 ml/min at the detection wavelength of 254 nm. During testing, the mobile phase was 0.01 mol/L phos- phate buffer solution (PBS, pH 6.5) that mixed with methanol (99.7%) in a ratio of 85/15 (v/v). Concentra- tions of ATP and its metabolites were calculated by comparing peak areas with appropriate standards. After experiment-2, the brain samples homogenized were mixed with sulfosalicylic acid (3%) for 15 min prior to centrifugation. The pH value of supernatants were then adjusted to 2-3 using LiOH (3 mol/L). Brain GABA and Glu in samples were determined by an auto amino acid analyzer (JLC-500/V2, Jeol Ltd., Tokyo, Japan) in accordance with the manufacturer’s specifica- tions. Briefly, it was an ionic exchange chromatography with a stepwise elution of free amino acids, and detec- tion with ninhydrine. The final results were calculated on the basis of the corresponding standards, and ex- pressed as concentrations of nmol/mg in brain tissues. 2.4. Statistical Analysis The obtained data were analyzed using a two-tailed Stu- dent’s t-test, and results were expressed as mean ± SD. Statistic difference was considered to be significant at p < 0.05(*). 3. RESULTS 3.1. Brain ATP As results shown in Figure 1, the ATP of control (Con.) group in the cerebrum, brainstem, and whole brain were 0.114 ± 0.020, 0.109 ± 0.015 and 0.134 ± 0.022 nmol/ mg, respectively. Contrasted to Control (Con.) Groups, the brain ATP declined in both of the whole brain and the single brain region after PLAE administrations. In detail, The ATP from PLAE groups were slightly reduced in cerebrums, while significant degradations were observed (p < 0.05) in the brainstems and whole brains on com- parison with Con. groups. In the case of PSAE treat- ments, the enhancements of ATP were occurred in all brain regions, especially in the cerebrums. 3.2. Brain AMP In experiment-1, we also obtained the AMP concentra- tions of 0.072 ± 0.013, 0.078 ± 0.005, 0.082 ± 0.007 nmol/mg respectively in the cerebrum, brainstem, and whole brain from Con. groups. As can been seen from Figure 2, after PLAE treated, the AMP were decreased in almost all brain regions (cerebellum results were not showed in this figure), particularly reduced (p < 0.05) in the cerebrum and brainstem regions. The AMP from PSAE groups showed no statistic changes compared
 270 X. Y. Zu et al. / J. Biomedical Science and Engineering 3 (2010) 268-273 Copyright © 2010 SciRes JBiSE Figure 1. ATP concentrations in the cerebrum, brainstem and whole brain of freely behaving rats at experiment-1. Open bars, Con. (Control, distilled water); closed bars, PLAE (500 mg/kg BW); di- agonal bars, PSAE (500 mg/kg BW). Data were expressed as mean ± SD of 5-6 independent sam- ples (*p < 0.05, vs. Con.). Figure 2. AMP concentrations in the cerebrum, brainstem and whole brain of freely behaving rats at experiment-1. Open bars, Con. (Control, distilled water); closed bars, PLAE (500 mg/kg BW); di- agonal bars, PSAE (500 mg/kg BW). Data were expressed as mean ± SD of 5-6 independent sam- ples (*p < 0.05, vs. Con.). with group Con. though the trails, although the declines were observed as well. 3.3. Brain Ad and Lactate Figure 3 showed the results of Ad and lactate in Cere- brum, and revealed that the Lactate changes were con- sistent with Ad in cerebrum after PLAE administrations in Experiment-1. As can be seen, compared with group Con. and PSAE, the Ad amelioration (p = 0.076) in PLAE group was occurred in cerebrum where played the main roles on modulating animal sleepiness. And the Lactate was elevated (p < 0.05) in cerebrum simultane- ously after PLAE treated. On the other hand, PSAE re- sults presented only slight differences in contrast to the Con. groups. 3.4. Brain GABA Brain GABA of PLAE-0 group were 0.767±0.180 and 0.767 ± 0.079 nmol/mg respectively in the brainstem and Whole brain. And the GABA results of cerebrums and cerebellums were monotonous and eliminated from Figure 4. In the results, we found that the GABA con- centrations exceeded statistically (p < 0.05) than PLAE-0 group in brainstems at both PLAE-100 and PLAE-500 groups. The GABA from PLAE-500 group showed sig- nificant improvement (p < 0.05) even in the whole brain of the experimental rats. 3.5. Brain Glu After experiment-2, we investigated the brain Glu contents in different brain regions simultaneously. As Figure 5 Figure 3. Concentrations of Ad and Lactate in the cerebrum of freely behaving rats at experiment-1. Open bars, Con. (Control, distilled water); closed bars, PLAE (500 mg/kg BW); diago- nal bars, PSAE (500 mg/kg BW). Data were expressed as mean ± SD of 5-6 independent samples (*p < 0.05, vs. Con.).
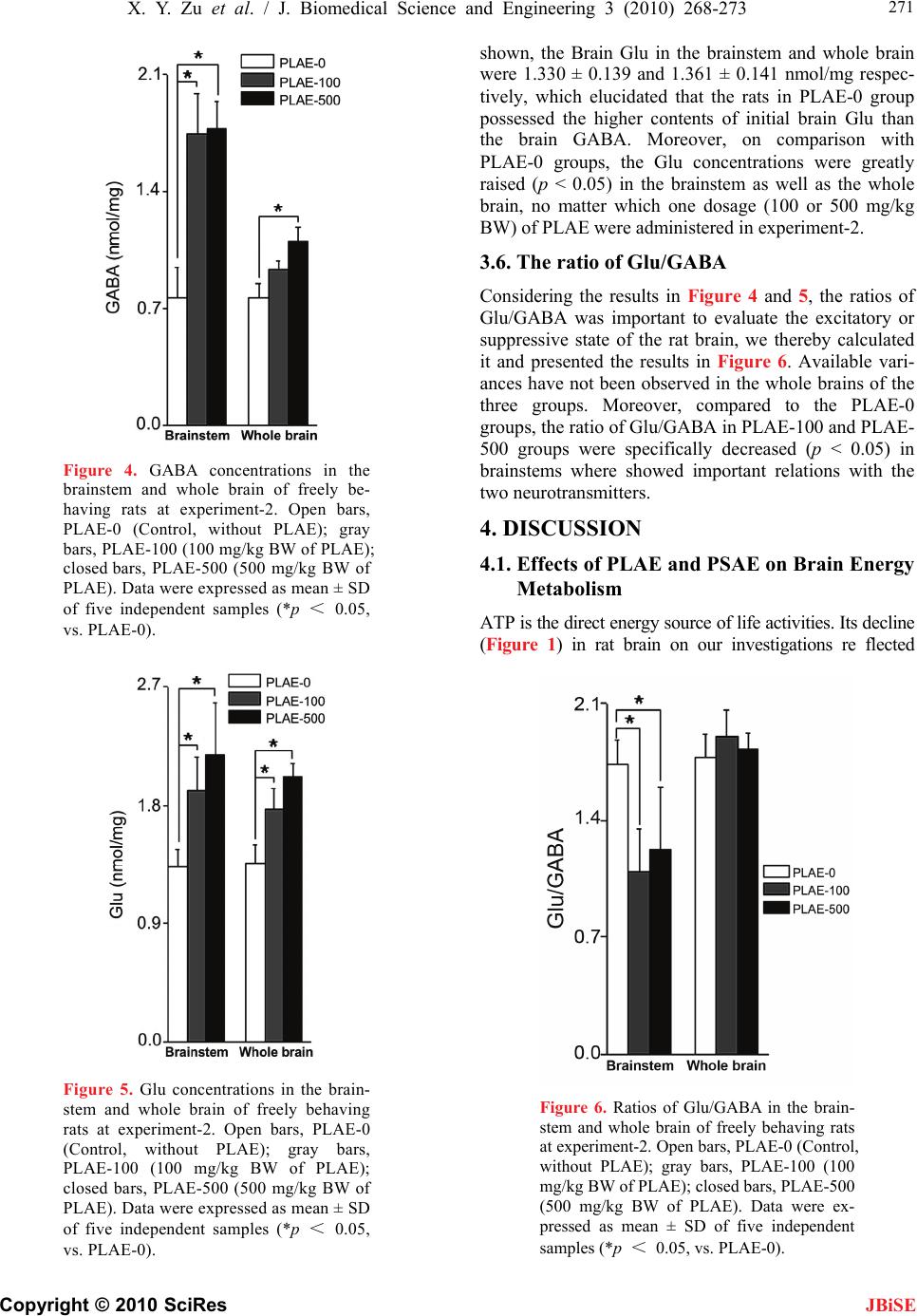 X. Y. Zu et al. / J. Biomedical Science and Engineering 3 (2010) 268-273 Copyright © 2010 SciRes 271 JBiSE Figure 4. GABA concentrations in the brainstem and whole brain of freely be- having rats at experiment-2. Open bars, PLAE-0 (Control, without PLAE); gray bars, PLAE-100 (100 mg/kg BW of PLAE); closed bars, PLAE-500 (500 mg/kg BW of PLAE). Data were expressed as mean ± SD of five independent samples (*p < 0.05, vs. PLAE-0). Figure 5. Glu concentrations in the brain- stem and whole brain of freely behaving rats at experiment-2. Open bars, PLAE-0 (Control, without PLAE); gray bars, PLAE-100 (100 mg/kg BW of PLAE); closed bars, PLAE-500 (500 mg/kg BW of PLAE). Data were expressed as mean ± SD of five independent samples (*p < 0.05, vs. PLAE-0). shown, the Brain Glu in the brainstem and whole brain were 1.330 ± 0.139 and 1.361 ± 0.141 nmol/mg respec- tively, which elucidated that the rats in PLAE-0 group possessed the higher contents of initial brain Glu than the brain GABA. Moreover, on comparison with PLAE-0 groups, the Glu concentrations were greatly raised (p < 0.05) in the brainstem as well as the whole brain, no matter which one dosage (100 or 500 mg/kg BW) of PLAE were administered in experiment-2. 3.6. The ratio of Glu/GABA Considering the results in Figure 4 and 5, the ratios of Glu/GABA was important to evaluate the excitatory or suppressive state of the rat brain, we thereby calculated it and presented the results in Figure 6. Available vari- ances have not been observed in the whole brains of the three groups. Moreover, compared to the PLAE-0 groups, the ratio of Glu/GABA in PLAE-100 and PLAE- 500 groups were specifically decreased (p < 0.05) in brainstems where showed important relations with the two neurotransmitters. 4. DISCUSSION 4.1. Effects of PLAE and PSAE on Brain Energy Metabolism ATP is the direct energy source of life activities. Its decline (Figure 1) in rat brain on our investigations re flected Figure 6. Ratios of Glu/GABA in the brain- stem and whole brain of freely behaving rats at experiment-2. Open bars, PLAE-0 (Control, without PLAE); gray bars, PLAE-100 (100 mg/kg BW of PLAE); closed bars, PLAE-500 (500 mg/kg BW of PLAE). Data were ex- pressed as mean ± SD of five independent samples (*p < 0.05, vs. PLAE-0).
 272 X. Y. Zu et al. / J. Biomedical Science and Engineering 3 (2010) 268-273 Copyright © 2010 SciRes JBiSE the requirement of energy reserves, and indicated the potential of rat sleepiness. AMP can transfer into ATP or degraded into Ad [9]. According to the deficiency of ATP in the results, the reduction of AMP (Figure 2) in group PLAE elucidated the tendency of its breaking down to Ad. As reported previously, Ad is a critical neurotransmitter in brain sleep system [10], which ac- cumulates in the cholinergic basal forebrain of cerebrum, has been proposed as one of the important homeostatic sleep factors [11]. In our researches, as a result of the deficiency of high-energy phosphates, the observed in- creases of Ad concentrations in cerebrums reflected a bio-energetic stress of animals. Energy depletion can build up the sleep pressure [7] and it is an available pathway and measure to explain the PLAE induced sleep propensity. From experiment-1, the great decrease of the ATP and AMP strongly indicated that the PLAE was promising in driving homeostatic sleeps of freely be- having rats. Besides, the insignificant accumulation of Ad in the PLAE group suggested a mild effect of PLAE on sleep ameliorating. Lactate, an end product of metabolic system, can be regarded as an additional metabolic marker of the sleep pressure. Our results (Figure 3) illuminated that Lactate changed together with Ad, in agreement with those re- ported previously [12] and indicative of the PLAE con- tributions in sedative or hypnic propensities. In all PSAE investigations, the rat brain energy sys- tem presented the great enhancement of ATP in cere- brum compared with Control and PLAE groups. How- ever, such variations were disadvantageous for estab- lishing the sleep pressure and meliorating the sleep of our experimental rats. 4.2. Effects of PLAE on Brain GABA, Glu and the Ratio of Glu/GABA GABA is a type of inhibitory neurotransmitter amino acid in brain. To clarify the PLAE effects on brain GABA, fifteen rats were employed in experiment-2 and randomly divided into three groups, and treated with PLAE in diverse dosages for 14 days. The GABA- contained neurons in brainstem and thalamic nucleus released GABA neurotransmitters [13,14], which can response for inhibiting target neurons of the arousal sys- tems in brainstem [15] and play an important role in meliorating rats’ sleep [6]. Thus, the GABA increase in our studies (Figure 4) not only suggested the specific effect region (brainstem) of GABA-neurotransmitter, and also testified the sedative efficacy of PLAE. It is well demonstrated that Glu play an excitatory role in animal brain, and then drives the animals to arousal. Glu receptors, expressed in the basal forebrain [16,17], stimulate gamma and theta electroencephalographic (EEG) activities of arousal and then suppress rat sleepi- ness [18,19]. In these researches, we found that PLAE also aroused the neurons to release Glu neurotransmit- ters (Figure 5) for exciting the rat brains. In deed, GABA can be formed from Glu primarily via the action of Glu decarboxylase [20]. Glu/GABA in brainstem is a type of available parameters that can characterize the state of sleep-wake system [21]. Since GABA is inhibitory and Glu is excitatory, both of them control many tranquilizing or exciting processes in rat brain. Apparently, PLAE we used stimulated both the brain Glu and the brain GABA. However, the sleep-modulation effects of Glu sited specifically in perifornical-lateral hypothalamic area [22] of the brain- stem which is the main region that releases GABA to inhibit the arousal of animal. Hence, the Glu/GABA decrease in this area (Figure 6) indicated superior GABA efficacy than Glu, and preferred to drive rats into drowsiness. On the other hand, as a mild tranquilizer, it was not surprising that PLAE significantly elevated GABA-mediated neurotransmission, and reduced Glu/ GABA in target brain region. Thus, in profile, it was still suggested the PLAE efficacy on spontaneous sleep im- provement. In summary, PLAE resulted in the great ATP con- sumption, slight Ad accumulation, as well as the signifi- cant decrease of Glu/GABA in corresponding brain areas. It revealed a mild hypnotic effect of PLAE on sleep ameliorations. In the case of sedatives, the PSAE was considered to be ineffective on meliorating rats’ sleeps at least view the matter from our investigations. On the other hand, in these researches, more detailed works on the effect components of such PLAE are desired to be determined in the future. Moreover, apart from the aqueous extracts of peanut leaves and stems, alcohol extracts of them are needed as well to as the contrasts in further investigations. REFERENCES [1] Diana, M.T., Carol, A.L., Heidi, P. and Michael, V.V. (2007) A systematic review of valerian as a sleep aid: Safe but not effective. Sleep Medicine Reviews, 11, 209-230. [2] Wang, Q.C., Xu, J. and Shi, M. (2001) Clinical efficacy of Groundnut leaves on insomnia treatments. Shanghai Journal of Traditional Chinese Medicine, 5, 8-10. [3] Hu, P.F., Fan, R.P., Li, Y.P. and Pang, C.Y. (2001) Studies on pharmacological action of Luohuashengzhiye extracts. Chinese Traditional Patent Medicine, 23, 919- 920. [4] Wang, Y.F., Li, H.F., Xu, Y.F., Zhang, Y.L., Xu, D.S., Xiao, L.M. and Li, X.M. (2001). Clinical confirmation of preparation from the branch and leaf of peanut in treating insomnia. Shanghai Journal of Traditional Chinese Medicine, 35, 8-10. [5] Saper, C.B., Scammell, T.E. and Lu, J. (2005) Hypotha- lamic regulation of sleep and circadian rhythms. Nature, 437, 1257-1263.
 X. Y. Zu et al. / J. Biomedical Science and Engineering 3 (2010) 268-273 Copyright © 2010 SciRes 273 JBiSE [6] Jones, B.E. (2005) From waking to sleeping: Neuronal and chemical substrates. Trends in Pharmacological Sci- ences, 26, 578-586. [7] Thakkar, M.M., Engemann, S.C., Walsh, K.M. and Sa- hota, P.K. (2008) Adenosine and the homeostatic control of sleep: Effects of A1 receptor blockade in the perifor- nical lateral hypothalamus on sleep-wakefulness. Neuro- science, 153, 875-880. [8] Schweinsberg, P.D. and Loo, T.L. (1980) Simultaneous analysis of ATP, ADP, AMP, and other purines in human erythrocytes by high-performance liquid chromatography. Journal of Chromatography, 181, 103-107. [9] Dworak, M., Diel, P., Voss, S., Hollmann, W.K. and Strüder, H. (2007) Intense exercise increases adenosine concentrations in rat brain: Implications for a homeo- static sleep drive. Neuroscience, 150, 789-795. [10] Huang, Z.L., Urade, Y. and Hayaishi, O. (2007) Pros- taglandins and adenosine in the regulation of sleep and wakefulness. Current Opinion in Pharmacology, 7, 33-38. [11] Kalinchuk, A.V., Urrila, A.S., Alanko, L., Heiskanen, S., Wigren, H.K., Suomela, M., Stenberg, D. and Porkka- Heiskanen, T. (2003) Local energy depletion in the basal forebrain increases sleep. European Journal of Neuro- science, 17, 863-869. [12] Wigren, H.K., Schepens, M., Matto, V., Stenberg, D. and Porkka-Heiskanen, T. (2007) Glutamatergic stimulation of the basal forebrain elevates extracellular adenosine and increases the subsequent sleep. Neuroscience, 147, 811-823. [13] Maloney, K.J., Mainville, L. and Jones, B.E. (1999) Dif- ferential c-Fos expression in cholinergic, mono-aminergic and GABAergic cell groups of the pontomesencephalic tegmentum after paradoxical sleep deprivation and re- covery. Neuroscience, 19, 3057-3072. [14] Maloney, K.J., Mainville, L. and Jones, B.E. (2000) c-Fos expression in GABAergic, serotonergic and other neurons of the pontomedullary reticular formation and raphe after paradoxical sleep deprivation and recovery. Neuroscience, 20, 4669-4679. [15] Sherin, J.E., Shiromani, P.J., McCarley, R.W. and Saper, C. B. (1996) Activation of ventrolateral preoptic neurons during sleep. Science, 271, 216-219. [16] Martin, L.J., Blackstone, C.D., Levey, A.I., Huganir, R. L. and Price, D.L. (1993) Cellular localizations of AMPA glutamate receptors within the basal forebrain magno- cellular complex of rat and monkey. Neuroscience, 13, 2249-2263. [17] Page, K.J. and Everitt, B.J. (1995) The distribution of neurons coexpressing immunoreactivity to AMPA- sensitive glutamate receptor subtypes (GluR1-4) and nerve growth factor receptor in the rat basal forebrain. European Journal of Neuroscience, 7, 1022-1033. [18] Cape, E.G. and Jones, B.E. (2000) Effects of glutamate agonist versus procaine microinjections into the basal forebrain cholinergic cell area upon gamma and theta EEG activity and sleep-wake state. European Journal of Neuroscience, 12, 2166-2184. [19] Fournier, G.N., Materi, L.M., Semba, K. and Rasmusson, D.D. (2004) Cortical acetylcholine release and electro- encephalogram activation evoked by ionotropic gluta- mate receptor agonists in the rat basal forebrain. Neuro- science, 123, 785-792. [20] Bown, A.W. and Shelp, B.J. (1997) The metabolism and functions of γ-aminobutyric acid. Plant Physiology, 115, 1-5. [21] Schousboe, A., Westergaard, N., Sonnewald, U., Petersen, S.B., Yu, A.C. and Hertz, L. (1992) Regulatory role of astrocytes for neuronal biosynthesis and homeostasis of glutamate and GABA. Progress in Brain Research, 94, 199-211. [22] Alam, M.A. and Mallick, B.N. (2008) Glutamic acid stimulation of the perifornical-lateral hypothalamic area promotes arousal and inhibits non-REM/REM sleep. Neuroscience Letters, 439, 281-286.
|