 Vol.2, No.1, 18-25 (2010) Natural Science http://dx.doi.org/10.4236/ns.2010.21003 Copyright © 2010 SciRes. OPEN ACCESS Hydriding and dehydriding kinetics of melt spun nanocrystalline Mg20Ni10-xCux (x = 0-4) alloys Yang-Huan Zhang1,2,*, Dong-Liang Zhao1, Bao-Wei Li2, Hui-Ping Ren1, Shi-Hai Guo1, Xin-Lin Wang1 1Department of Functional Material Research, Central Iron and Steel Research Institute, Beijing, China 2School of Material, Inner Mongolia University of Science and Technology, Baotou, China; zyh59@yahoo.com.cn Received 22 October 2009; revised 13 November 2009; accepted 17 November 2009. ABSTRACT The nanocrystalline Mg2Ni-type electrode alloys with nominal compositions of Mg20Ni10-xCux (x = 0, 1, 2, 3, 4) were synthesized by melt-spinning technique. The microstructures of the alloys were characterized by XRD, SEM and HRTEM. The hydrogen absorption and desorption kinet- ics of the alloys were measured using an auto- matically controlled Sieverts apparatus. The re- sults show that all the as-spun alloys hold ty- pical nanocrystalline structure. The substitution of Cu for Ni does not change the major phase Mg2Ni but it leads to the formation of the sec- ondary phase Mg2Cu. The hydrogen absorption capacity of the alloys first increases and then decreases with rising Cu content, but the hy- drogen desorption capacity of the alloys mono- tonously grows with increasing Cu content. The melt spinning significantly improves the hydro- genation and dehydrogenation capacities and kinetics of the alloys. Keywords: Mg2Ni-Type Alloy; Substituting Ni with Cu; Melt Spinning; Hydriding and Dehydriding Kinetics 1. INTRODUCTION Mg and Mg-based alloys has been considered as poten- tial materials for solid state hydrogen storage in the form of metallic hydrides such as MgH2 and Mg2NiH4. The theoretical hydrogen storage capacities of MgH2 and Mg2NiH4 are 7.6 wt.% and 3.6 wt.% [1,2] respectively, which is quite adequate for commercial applications as a hydrogen fuel source [3]. Unfortunately, the slow sorp- tion/desorption kinetics and high dissociation tempera- ture of these kinds of metal hydrides limit their practical application. Therefore, finding ways of improving the hydration kinetics of Mg-based alloys has been one of the main challenges faced by researchers in this area. Various attempts, involving mechanical alloying (MA) [4], GPa hydrogen pressure method [5], melt spinning [6], gravity casting [7], polyol reduction [8], hydriding combustion synthesis [9], surface modification [10], al- loying with other elements [11,12], adding catalysts [13] etc, have been undertaken to improve the activation and hydriding property. Gennari et al. [14] reported that the nanocrystalline Mg2Ni synthesized by combined milling-annealing pro- cedure can readily hydrogen absorption during the first cycle and show excellent absorption kinetics at 200. ℃ Muthukumar et al. [15] confirmed that a maximum hy- drogen capacity of 3.67 wt.% for the Mg2Ni alloy pre- pared by mechanical alloying (MA) could be achieved for an initial absorption temperature of 300 ℃ and sup- ply pressure of 20 bar. Recham et al. [16] found that the hydrogen absorption property of ball-milled MgH2 can be enhanced by adding NbF5, and the milled MgH2+ NbF5 composite can desorbs 3 wt.% H2 at 150℃. Do- brovolsky et al. [17] synthesized a MgH2 (50 wt.%) + TiB2 (50 wt.%) composite by intensive mechanical mil- ling and found that TiB2 addition decreases the dissocia- tion temperature of the MgH2 hydride by about 50.℃ Indubitably, ball-milling is a very effective method for the preparation of nanocrystalline and amorphous Mg and Mg-based alloys. Particularly, it is suitable to solu- bilize particular elements into MgH2 or Mg2NiH4 above the thermodynamic equilibrium limit, which is helpful to destabilize MgH2 or Mg2NiH4 [18]. However, the milled Mg and Mg-based alloys show very poor hydrogen ab- sorbing and desorbing stability due to the fact that the metastable structures formed by ball milling tended to vanish during multiple hydrogen absorbing and desorb- ing cycles [19]. Alternatively, melt-spun technique can overcome the above mentioned shortcoming and effec- tive avoiding the significant degradation of hydrogen absorbing and desorbing cycle properties of Mg and Mg- based [20]. Additionally, the melt-spinning technique is a very effective method to obtain a nanocrystalline struc- ture and is very suitable for mass-production of nano- crystalline Mg-based alloys. It was also clarified that nanocrystalline alloys produced by melt-spinning could
 Y. H. Zhang et al. / Natural Science 2 (2010) 18-25 Copyright © 2010 SciRes. OPEN ACCESS 19 19 have excellent hydriding characteristics even at room temperature, similar to the alloys produced by the MA process. Spassov et al. [21] prepared Mg2(Ni, Y) hydro- gen storage alloy with exact composition Mg63Ni30Y7 by rapid solidification, and its maximum hydrogen absorp- tion capacity (about 3.0 wt.%) and hydrogenation kinet- ics of the as-spun Mg2(Ni, Y) alloy were found to ex- ceed those of the polycrystalline Mg2Ni alloys prepared by conventional technology and to be comparable to the hydrogen absorption characteristics of ball-milled na- nocrystalline Mg2Ni. It was determined that the addition of third element stabilizes the nanostructure of Mg-Ni based alloy, which could be very important for practical H-storage materials. Our previous work indicated that the substitution of Co for Ni significantly improved the hydriding and de- hydriding kinetics of the Mg2Ni-type alloys [22]. There- fore, it is very desirable to investigate the influence of substituting Ni with Cu on the hydriding and dehydrid- ing characteristics of Mg2Ni-type alloys prepared by melt-spinning. The objective of this work is to produce the Mg-Ni-based ternary nanocrystalline alloys by melt spinning and to examine their structures and hydriding and dehydriding kinetics. 2. EXPERIMENTAL METHODS The alloy ingots were prepared using a vacuum induc- tion furnace in a helium atmosphere at a pressure of 0.04 MPa. Part of the as-cast alloys was re-melted and spun by melt-spinning with a rotating copper roller. The spin- ning rate was approximately expressed by the linear ve- locity of the copper roller because it is too difficult to measure a real spinning rate i.e. cooling rate of the sam- ple during spinning. The spinning rates used in the ex- periment were 15, 20, 25 and 30 m/s, respectively. The nominal compositions of the experimental alloys were Mg20Ni10-xCux (x = 0, 1, 2, 3, 4). For convenience, the alloys were denoted with Cu content as Cu0, Cu1, Cu2, Cu3 and Cu4, respectively. The morphologies of the as-cast alloys were observed by scanning electron microscope (SEM) (Philips Q- UANTA 400). The phase structures of the as-cast and spun alloys were determined by XRD diffractometer (D/ max/2400). The diffraction, with the experimental pa- rameters of 160 mA, 40 kV and 10°/min respectively, was performed with CuKα1 radiation filtered by graphite. The thin film samples of the as-spun alloys were pre- pared by ion etching for observing the morphology with high resolution transmission electron microscope (H- RTEM) (JEM-2100F, operated at 200 kV), and for de- termining the crystalline state of the samples with elec- tron diffraction (ED). The hydrogen absorption and desorption kinetics of the alloys were measured by an automatically controlled Sieverts apparatus. The hydrogen absorption was con- ducted at 1.5 MPa at 200 and the hydrogen desor℃p- tion in a vacuum (1×10-4 MPa) at 200 too.℃ 3. RESULTS AND DISCUSSIONS 3.1. Microstructure Characteristics The SEM images of the as-cast alloy are illustrated in Figure 1, displaying a typical dendrite structure. The substitution of Cu for Ni does not change the morphol- ogy of the alloys but it causes a significant refinement of the grains. The result obtained by energy dispersive spectrometry (EDS) indicates that the major phase of the as-cast alloys is Mg2Ni phase (denoted as A). Some small massive matters in the alloys containing Cu can clearly be seen in Figure 1, which are determined by EDS to be Mg2Cu phase (denoted as B). Figure 2 presents the XRD profiles of the as-cast and spun Mg20Ni10-xCux (x = 0, 1, 2, 3, 4) alloys, showing that the substitution of Cu for Ni does not change the phase structure. All the as-cast and spun alloys display a single phase structure. This seems to be contrary with the result of SEM observation. It is most probably asso- ciated with the fact that Mg2Ni and Mg2Cu hold com- pletely identical structure and nearly same lattice con- stants. On the other hand, the amount of the Mg2Cu phase is very little so that the XRD observation can not detect its presence. Listed in Table 1 are the lattice pa- rameters, cell volume and full width at half maximum (FWHM) values of the main diffraction peaks of the as-cast and spun (20 m/s) alloys which were calculated by software of Jade 6.0. It can be derived from Table 1 that the substitution of Cu for Ni causes the FWHM values of the main diffraction peaks of the as-cast and spun alloys significantly increased, and it leads to the lattice parameters and cell volume of the alloys cleverly enlarged, which is attributed to the slightly larger atomic radius of Cu than Ni. It can be seen from Table 1 that melt spinning causes the FWHM values of the main dif- fraction peaks of the alloys significantly increased which is doubtless attributed to the refinement of the grains and the stored stress in the grains produced by melt spinning. Based on the FWHM values of the broad diffraction peak (203) in Figure 2b, the crystallite size <Dhkl > (Ǻ) of the as-spun alloy was calculated using Scherrer’s equation. The grain sizes of the as-spun alloys are in a range of 2-6 nm, consistent with results reported by Friedlmeier et al. [23]. It is very important to notice that <D> values were calculated on the same peak having Miller indices (203) due to better possibility of mutual comparison. Figure 3 shows HRTEM micrographs and electron diffraction patterns of the as-spun Cu1 and Cu3 alloys, which reveal a nanocrystalline microstructure, with an average crystal size of about 2-5 nm. This result agrees very well with the XRD observation shown in Figure 2.
 Y. H. Zhang et al. / Natural Science 2 (2010) 18-25 Copyright © 2010 SciRes. OPEN ACCESS 20 From HRTEM observations there is some evidence that the as-spun alloys are strongly disordered and nanos- tructured, but they are free of amorphous phase. Spassov et al. [21] reported that Mg-based alloys with nanocrys- talline microstructures can be obtained by controlling the cooling rates. The crystal defects in the as-spun alloy, stacking faults (denoted as A), twin-grain boundaries (denoted as B), dislocations (denoted as C) and sub- grain boundaries (denoted as D), can clearly be seen in Figure 4. Figure 1. SEM images of the as-cast alloys together with EDS spectra of sections A and B in Figure 1 (b): (a) Cu0 alloy, (b) Cu4 alloy. Figure 2. XRD profiles of the as-cast and spun alloys: (a) As-cast, (b) As-spun (20 m/s). a 20 μm A b 20 μm B A Section A 0 2 4 6 8 Mg i i Cu Cu keV Mg Cu i Cu Section B 02468 10 keV 20 30 40 50 60 70 80 90100 (403) (204) (220) (118) (215) (206) (203) (113) (105) (200) (112) △ (103) (102) (101) (003) (100) △ △ △ △ △ △ △ △ △ △ △ △ △ △ △ Cu0 2θ(de g re e ) Cu1 Cu2 Cu3 (b) Cu4 △ -Mg2Ni 20 30 40 50 60 70 80 90100 (403) (204) (220) (118) (215) (206) (212) (211) (106) (203) (113)(105) (200) (112) (103) (102) (101) (003) (100) △ △ △ △ △ △ △ △ △ △ △ △ △ △ △ △ △ △ △Cu0 2θ(deg r ee) Cu1 Cu2 Cu3 (a) △ -Mg2Ni Cu4
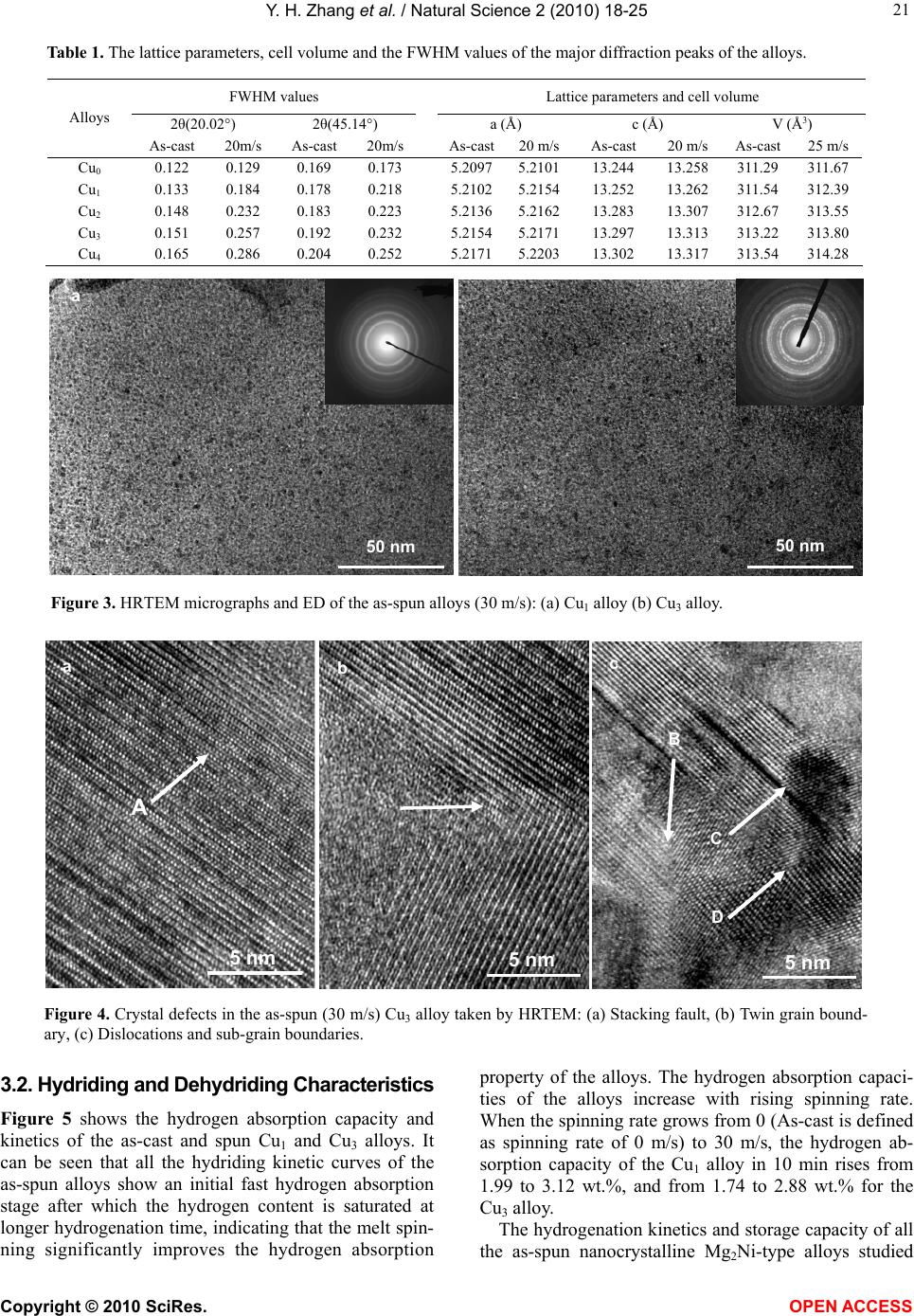 Y. H. Zhang et al. / Natural Science 2 (2010) 18-25 Copyright © 2010 SciRes. OPEN ACCESS 21 21 Table 1. The lattice parameters, cell volume and the FWHM values of the major diffraction peaks of the alloys. Figure 3. HRTEM micrographs and ED of the as-spun alloys (30 m/s): (a) Cu1 alloy (b) Cu3 alloy. Figure 4. Crystal defects in the as-spun (30 m/s) Cu3 alloy taken by HRTEM: (a) Stacking fault, (b) Twin grain bound- ary, (c) Dislocations and sub-grain boundaries. 3.2. Hydriding and Dehydriding Characteristics Figure 5 shows the hydrogen absorption capacity and kinetics of the as-cast and spun Cu1 and Cu3 alloys. It can be seen that all the hydriding kinetic curves of the as-spun alloys show an initial fast hydrogen absorption stage after which the hydrogen content is saturated at longer hydrogenation time, indicating that the melt spin- ning significantly improves the hydrogen absorption property of the alloys. The hydrogen absorption capaci- ties of the alloys increase with rising spinning rate. When the spinning rate grows from 0 (As-cast is defined as spinning rate of 0 m/s) to 30 m/s, the hydrogen ab- sorption capacity of the Cu1 alloy in 10 min rises from 1.99 to 3.12 wt.%, and from 1.74 to 2.88 wt.% for the Cu3 alloy. The hydrogenation kinetics and storage capacity of all the as-spun nanocrystalline Mg2Ni-type alloys studied FWHM values Lattice parameters and cell volume 2θ(20.02°) 2θ(45.14°) a (Å) c (Å) V (Å3) Alloys As-cast 20m/s As-cast 20m/sAs-cast20 m/sAs-cast 20 m/s As-cast 25 m/s Cu0 0.122 0.129 0.169 0.1735.20975.210113.244 13.258 311.29 311.67 Cu1 0.133 0.184 0.178 0.2185.21025.215413.252 13.262 311.54 312.39 Cu2 0.148 0.232 0.183 0.2235.21365.216213.283 13.307 312.67 313.55 Cu3 0.151 0.257 0.192 0.2325.21545.217113.297 13.313 313.22 313.80 Cu4 0.165 0.286 0.204 0.2525.21715.220313.302 13.317 313.54 314.28 50 nm a 50 nm 5 nm a A b 5 nm 5 nm c D C B
 Y. H. Zhang et al. / Natural Science 2 (2010) 18-25 Copyright © 2010 SciRes. OPEN ACCESS 22 Figure 5. Hydrogen absorption kinetic curves of the as-cast and spun alloys: (a) Cu1 alloy (b) Cu3 alloy. are superior to those of conventional polycrystalline ma- terials with similar composition. The enhanced hydro- genation property by melt spinning is undoubtedly asso- ciated with the refinement of the grains produced by melt spinning [24]. By refining the microstructure, a lot of new crystallites and grain boundaries are created which can act as fast diffusion paths for hydrogen ab- sorption. Based on the result reported by Orimo and Fu- jii [25], the distribution of the maximum hydrogen con- centrations in three nanometer-scale regions, i.e. grain region and grain boundary region as well as amorphous region, have been experimentally determined to be 0.3 wt.% H in the grain region of Mg2Ni, 4.0 wt.% H in the grain boundary and 2.2 wt.% H in the amorphous region. It revealed that the hydrides mainly exist in grain- boundary region and the amorphous phase region. The improved hydrogenation characteristics can be explained with the enhanced hydrogen diffusivity in the nanocrys- talline microstructure as the nanocrystalline leads to an easier access of hydrogen to the nanograins, avoiding the long-range diffusion of hydrogen through an already formed hydride, which is often the slowest stage of ab- sorption. It is known that the nanocrystalline micro- structures can accommodate higher amounts of hydrogen than polycrystalline ones. The crystalline material, when melt spun, becomes at least partially disordered and its structure changes to nanocrystalline. Consequently, high densities of crystal defects such as dislocations, stacking faults and grain boundaries are introduced. The large number of interfaces and grain boundaries available in the nanocrystalline materials provide easy pathways for hydrogen diffusion and accelerates the hydrogen ab- sorbing/desorbing process. The hydrogen absorption kinetic curves of the as-spun alloys are plotted in Figure 6. It can be seen that the hydrogen absorption capacity of as-spun alloys first in- creases and then decreases with the variation of Cu con- tent. The Cu2 alloy shows a maximum hydrogen absorp- tion capacity at 200℃. The kinetics of hydrogenation was extremely fast so that the alloys absorbed more than 95% of their hydrogen capacities within the first 5 min. The excellent hydriding kinetics is ascribed to the na- nocrystalline structure because the high surface to vol- ume ratios (high specific surface area) and the presence of large number of grain boundaries in nanocrystalline alloys enhance the kinetics of hydrogen absorption/ de- sorption. The positive function of Cu substitution on the hydrogen absorption capacity and kinetics of the alloy is attributed to the increased cell volume and the grain re- fined by Cu substitution. The increase of the cell volume is very helpful to hydrogen absorption capacity, and the grain boundary possesses the largest hydrogen absorp- tion capability [25]. It was well known that the catalytic action of Ni on hydriding is stronger than Cu. Therefore, it is understandable that a superfluous amount of Cu substitution (x>2) must lead to a decrease of the hydro- gen absorption capacity of the alloys. Figure 7 shows the hydrogen desorption capacity and kinetics of the as-cast and spun Cu1 and Cu3 alloys, in- dicating that the dehydriding capability of the alloys obviously meliorates with rising spinning rate. When the spinning rate grows from 0 to 30 m/s, the hydrogen de- sorption capacity of the Cu1 alloy in 20 min increases from 0.29 to 0.98 wt.%, and from 0.52 to 1.49 wt.% for Cu3 alloy, respectively. The nanocrystalline Mg2Ni- based alloys produced by melt spinning exhibit higher H-absorption capacity and faster kinetics of hydriding/ dehydriding than crystalline Mg2Ni. A similar result was reported by Spassov et al. [21]. The specific capacity and hydriding/dehydriding kinetics of hydride materials depend on their chemical composition and crystalline structure [26]. The observed essential differences in the hydriding/dehydriding kinetics of the melt-spun nano- crystalline Mg2Ni type alloys studied most probably have to be associated with the composition of the alloy as well as with the differences in their microstructure due to the different spinning rates. It was reported that 0 20406080100120 0.0 0.5 1.0 1.5 2.0 2.5 3.0 3.5 (a) Hydrogen absorptiom (wt.%) Time (min) As-cast 15 m/s 20 m/s 25 m/s 30 m/s 0 20406080100120 0.0 0.5 1.0 1.5 2.0 2.5 3.0 (b) Hydrogen absorption (wt.%) Time (min) As-cast 15 m/s 20 m/s 25 m/s 30 m/s
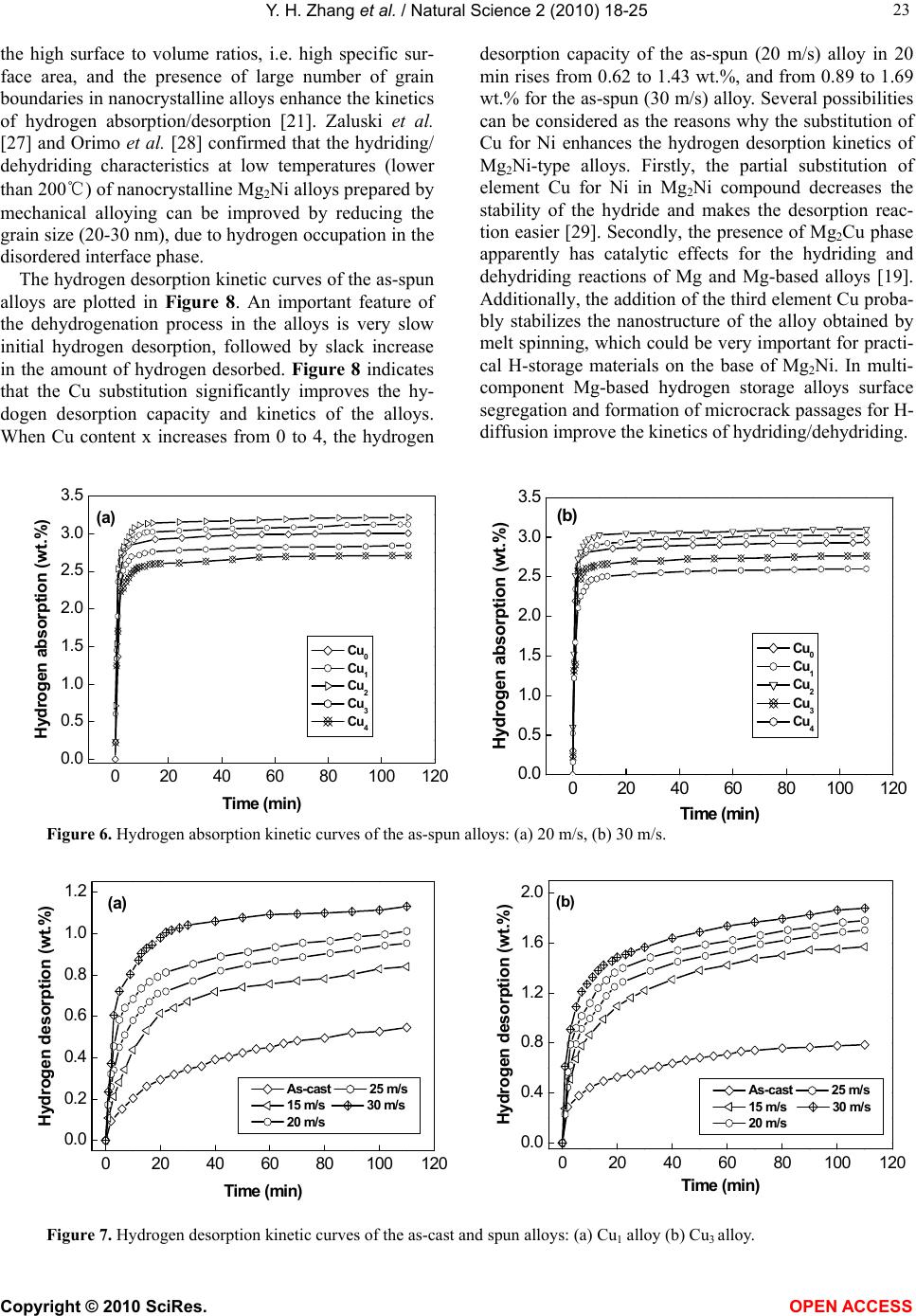 Y. H. Zhang et al. / Natural Science 2 (2010) 18-25 Copyright © 2010 SciRes. OPEN ACCESS 23 23 the high surface to volume ratios, i.e. high specific sur- face area, and the presence of large number of grain boundaries in nanocrystalline alloys enhance the kinetics of hydrogen absorption/desorption [21]. Zaluski et al. [27] and Orimo et al. [28] confirmed that the hydriding/ dehydriding characteristics at low temperatures (lower than 200℃) of nanocrystalline Mg2Ni alloys prepared by mechanical alloying can be improved by reducing the grain size (20-30 nm), due to hydrogen occupation in the disordered interface phase. The hydrogen desorption kinetic curves of the as-spun alloys are plotted in Figure 8. An important feature of the dehydrogenation process in the alloys is very slow initial hydrogen desorption, followed by slack increase in the amount of hydrogen desorbed. Figure 8 indicates that the Cu substitution significantly improves the hy- dogen desorption capacity and kinetics of the alloys. When Cu content x increases from 0 to 4, the hydrogen desorption capacity of the as-spun (20 m/s) alloy in 20 min rises from 0.62 to 1.43 wt.%, and from 0.89 to 1.69 wt.% for the as-spun (30 m/s) alloy. Several possibilities can be considered as the reasons why the substitution of Cu for Ni enhances the hydrogen desorption kinetics of Mg2Ni-type alloys. Firstly, the partial substitution of element Cu for Ni in Mg2Ni compound decreases the stability of the hydride and makes the desorption reac- tion easier [29]. Secondly, the presence of Mg2Cu phase apparently has catalytic effects for the hydriding and dehydriding reactions of Mg and Mg-based alloys [19]. Additionally, the addition of the third element Cu proba- bly stabilizes the nanostructure of the alloy obtained by melt spinning, which could be very important for practi- cal H-storage materials on the base of Mg2Ni. In multi- component Mg-based hydrogen storage alloys surface segregation and formation of microcrack passages for H- diffusion improve the kinetics of hydriding/dehydriding. Figure 6. Hydrogen absorption kinetic curves of the as-spun alloys: (a) 20 m/s, (b) 30 m/s. Figure 7. Hydrogen desorption kinetic curves of the as-cast and spun alloys: (a) Cu1 alloy (b) Cu3 alloy. 0 20406080100120 0.0 0.5 1.0 1.5 2.0 2.5 3.0 3.5 (b) Hydrogen absorption (wt.%) Time (min) Cu0 Cu1 Cu2 Cu3 Cu4 020406080100120 0.0 0.5 1.0 1.5 2.0 2.5 3.0 3.5 (a) Hydrogen absorption (wt.%) Time (min) Cu0 Cu1 Cu2 Cu3 Cu4 020 40 60 80100120 0.0 0.2 0.4 0.6 0.8 1.0 1.2 (a) Hydrogen desorption (wt.%) Time (min) As-cast 25 m/s 15 m/s 30 m/s 20 m/s 020 40 60 80100120 0.0 0.4 0.8 1.2 1.6 2.0 (b) Hydrogen desorption (wt.%) Time (min) As-cast 25 m/s 15 m/s 30 m/s 20 m/s
 Y. H. Zhang et al. / Natural Science 2 (2010) 18-25 Copyright © 2010 SciRes. OPEN ACCESS 24 Figure 8. Hydrogen desorption kinetic curves of the as-spun alloys: (a) 20 m/s, (b) 30 m/s. 4. CONCLUSIONS 1) All the as-spun Mg20Ni10-xCux (x = 0, 1, 2, 3, 4) alloys hold nanocrystalline structures and are free of amor- phous phase. The substitution of Cu for Ni does not change the major phase of the alloy, but it leads to a sig- nificant refinement of the grains and the formation of secondary phase Mg2Cu in the as-cast alloys. 2) Melt spinning significantly improves the hydriding and dehydriding properties of the alloys. Hydriding and dehydriding capacities and rates of the alloy markedly rise with increasing spinning rate, which is mainly at- tributed to the formation of the nanocrystalline structure caused by melt spinning. 3) With the substitution of Cu for Ni, the hydrogen absorption capacity of the alloys first increases and then decreases. But it clearly improves the hydrogen desorp- tion capacity and dehydriding rate of the alloys, for which the decreased stability of the hydride by Cu sub- stitution is mainly responsible. 5. ACKNOWLEDGEMENTS This work is supported by Hi-Tech Research and Development Pro- gram of China (2007AA03Z227), National Natural Science Founda- tions of China (50871050 and 50701011), Natural Science Foundation of Inner Mongolia, China (200711020703) and Higher Education Sci- ence Research Project of Inner Mongolia, China (NJzy08071). REFERENCES [1] Schlapbach, L. and Züttel, A. (2001) Hydrogen-storage materials for mobile applications [J]. Nature, 414, 353- 358. [2] Simičić, M.V., Zdujić, M., Dimitrijević, R., et al. (2006) Hydrogen absorption and electrochemical properties of Mg2Ni-type alloys synthesized by mechanical alloying [J]. Journal of Power Sources, 158, 730-734. [3] Schlapbach, L. (2002) Hydrogen as a fuel and its storage for mobility and transport [J]. MRS Bulletin, 27, 675-676. [4] Ebrahimi-Purkani, A. and Kashani-Bozorg, S.F. (2008) Nanocrystalline Mg2Ni-based powders produced by high-energy ball milling and subsequent annealing [J]. Journal of Alloys and Compounds, 456, 211-215. [5] Kyoi, D., Sakai, T., Kitamura, N., et al. (2008) Synthesis of FCC Mg-Ta hydrides using GPa hydrogen pressure method and their hydrogen-desorption properties [J]. Journal of Alloys and Compounds, 463, 306-310. [6] Palade, P., Sartori, S., Maddalena, A., et al. (2006) Hy- drogen storage in Mg-Ni-Fe compounds prepared by melt spinning and ball milling [J]. Journal of Alloys and Compounds, 415, 170-176. [7] Song, M.Y., Yim, C.D., Bae, J.S, et al. (2008) Prepara- tion by gravity casting and hydrogen-storage properties of Mg-23.5 wt.%Ni-(5, 10 and 15 wt.%)La [J]. Journal of Alloys and Compounds, 463, 143-147. [8] Hima Kumar, L., Viswanathan, B. and Srinivasa Murthy S. (2008) Hydrogen absorption by Mg2Ni prepared by polyol reduction [J]. Journal of Alloys and Compounds, 461, 72-76. [9] Liu, X.F., Zhu, Y.F. and Li, L.Q. (2008) Structure and hydrogenation properties of nanocrystalline Mg2Ni pre- pared by hydriding combustion synthesis and mechanical milling [J]. Journal of Alloys Compounds, 455, 197-202. [10] Liu, F.J. and Suda, S. (1995) A method for improving the long-term storability of hydriding alloys by air water ex- posure [J]. Journal of Alloys Compounds, 231, 742-750. [11] Czujko, T., Varin, R.A., Chiu, C., et al. (2006) Investiga- tion of the hydrogen desorption properties of Mg+10 wt.% X (X = V, Y, Zr) submicrocrystalline composites [J]. Journal of Alloys Compounds, 414, 240-247. [12] Gasiorowski, A., Iwasieczko, W., Skoryna., et al. (2004) Hydriding properties of nanocrystalline Mg2−xMxNi al- loys synthesized by mechanical alloying (M = Mn, Al) [J]. Journal of Alloys Compounds, 364, 283-288. [13] Sakintuna, B., Lamari-Darkrim, F. and Hirscher, M. (2007) Metal hydride materials for solid hydrogen stor- age: A review [J]. International Journal of Hydrogen Energy, 32, 1121-1140. [14] Gennari, F.C. and Esquivel, M.R. (2008) Structural char- acterization and hydrogen sorption properties of nanocrystalline Mg2Ni [J]. Journal of Alloys Compounds, 459, 425-432. [15] Muthukumar, P., Prakash Maiya, M., Srinivasa Murthy, 020 40 60 80100120 0.0 0.4 0.8 1.2 1.6 2.0 (a) Hydrogen desorption (wt.%) Time (min) Cu0 Cu3 Cu1 Cu4 Cu2 0 20406080100120 0.0 0.4 0.8 1.2 1.6 2.0 (b) Hydrogen desorption (wt.%) Time (min) Cu0 Cu3 Cu1 Cu4 Cu2
 Y. H. Zhang et al. / Natural Science 2 (2010) 18-25 Copyright © 2010 SciRes. OPEN ACCESS 25 25 S., et al. (2008) Tests on mechanically alloyed Mg2Ni for hydrogen storage [J]. Journal of Alloys Compounds, 452, 456-461. [16] Recham, N., Bhat, V.V., Kandavel, M., et al. (2008) Reduction of hydrogen desorption temperature of ball- illed MgH2 by NbF5 addition [J]. Journal of Alloys Compounds, 464, 377-382. [17] Dobrovolsky, V.D., Ershova, O.G., Solonin, Yu. M., et al. (2008) Influence of TiB2 addition upon thermal sta- bility and decomposition temperature of the MgH2 hy- dride of a Mg-based mechanical alloy [J]. Journal of Al- loys Compounds, 465, 177-182. [18] Liang, G. (2004) Synthesis and hydrogen storage proper- ties of Mg-based alloys [J]. Journal of Alloys Compounds, 370, 123-128. [19] Song, M.Y., Kwon, S.N., Bae, J.S., et al. (2008) Hydro- gen-storage properties of Mg-23.5Ni-(0 and 5)Cu pre- pared by melt spinning and crystallization heat treatment [J]. International Journal of Hydrogen Energy, 33, 1711-1718. [20] Savyak, M., Hirnyj, S., Bauer, H.D., et al. (2004) Elec- trochemical hydrogenation of Mg65Cu25Y10 metallic glass [J]. Journal of Alloys Compounds, 364, 229-237. [21] Spassov, T. and Köster, U. (1998) Thermal stability and hydriding properties of nanocrystalline melt-spun Mg63Ni30Y7 alloy [J]. Journal of Alloys Compounds, 279, 279-286. [22] Zhang,Y.H., Li, B.W., Ren, H.P., et al. (2009) Hydriding and dehydriding characteristics of nanocrystalline and amorphous Mg20Ni10-xCox (x=0–4) alloys prepared by melt-spinning [J]. International Journal of Hydrogen Energy, 34, 2684-2691. [23] Friedlmeier, G., Arakawa, M., Hiraia, T., et al. (1999) Preparation and structural, thermal and hydriding char- acteristics of melt-spun Mg-Ni alloys [J]. Journal of Al- loys Compounds, 292, 107-117. [24] Tanaka, K., Kanda, Y., Furuhashi, M., et al. (1999) Im- provement of hydrogen storage properties of melt-spun Mg-Ni-RE alloys by nanocrystallization [J]. Journal of Alloys Compounds, 293-295, 521-525. [25] Orimo, S. and Fujii, H. (2001) Materials science of Mg-Ni-based new hydrides [J]. Applied Physics A, 72, 167-186. [26] Mulas, G., Schiffini, L. and Cocco, G. (2004) Mechano- chemical study of the hydriding properties of nanostruc- tured Mg2Ni–Ni composites [J]. Journal of Materials Research, 19, 3279-3289. [27] Zaluski, L., Zaluska, A.J. and Ström-Olsen, O. (1997) Nanocrystalline metal hydrides [J]. Journal of Alloys Compounds, 253-254, 70-79. [28] Orimo, S., Fujii, H. and Ikeda, K. (1997) Notable hy- driding properties of a nanostructured composite material of the Mg2Ni-H system synthesized by reactive me- chanical grinding [J]. Acta Materialia, 45, 331-341. [29] Woo, J.H. and Lee, K.S. (1999) Electrode characteristics of nanostructured MgNi-type alloys prepared by me- chanical alloying [J]. Journal of The Electrochemical So- ciety, 146, 819-823.
|