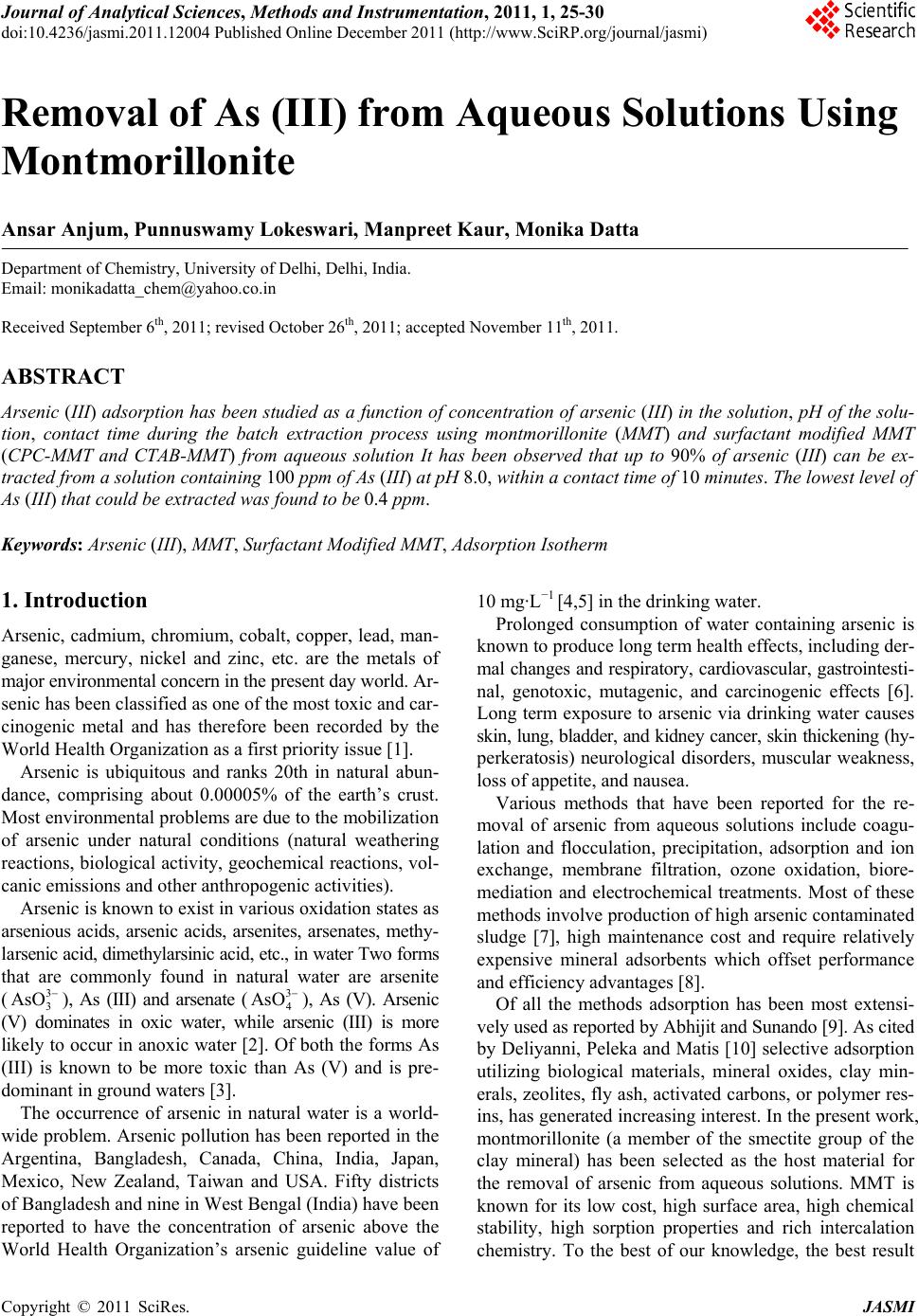
Journal of Analytical Sciences, Methods and Instrumentation, 2011, 1, 25-30
doi:10.4236/jasmi.2011.12004 Published Online December 2011 (http://www.SciRP.org/journal/jasmi)
Copyright © 2011 SciRes. JASMI
25
Removal of As (III) from Aqueous Solutions Using
Montmorillonite
Ansar Anjum, Punnuswamy Lokeswari, Manpreet Kaur, Monika Datta
Department of Chemistry, University of Delhi, Delhi, India.
Email: monikadatta_chem@yahoo.co.in
Received September 6th, 2011; revised October 26th, 2011; accepted November 11th, 2011.
ABSTRACT
Arsenic (III) adsorption has been studied as a function of concentration of arsenic (III) in the solution, pH of the solu-
tion, contact time during the batch extraction process using montmorillonite (MMT) and surfactant modified MMT
(CPC-MMT and CTAB-MMT) from aqueous solution It has been observed that up to 90% of arsenic (III) can be ex-
tracted from a solution containing 100 ppm of As (III) at pH 8.0, within a contact time of 10 minutes. The lowest level of
As (III) that could be extracted was found to be 0.4 ppm.
Keywords: Arsenic (III), MMT, Surfactant Modified MMT, Adsorption Isotherm
1. Introduction
Arsenic, cadmium, chromium, cobalt, copper, lead, man-
ganese, mercury, nickel and zinc, etc. are the metals of
major environmental concern in the present day world. Ar-
senic has been classified as one of the most toxic and car-
cinogenic metal and has therefore been recorded by the
World Health Organization as a first priority issue [1].
Arsenic is ubiquitous and ranks 20th in natural abun-
dance, comprising about 0.00005% of the earth’s crust.
Most environmental problems are due to the mobilization
of arsenic under natural conditions (natural weathering
reactions, biological activity, geochemical reactions, vol-
canic emissions and other anthropogenic activities).
Arsenic is known to exist in various oxidation states as
arsenious acids, arsenic acids, arsenites, arsenates, methy-
larsenic acid, dimethylarsinic acid, etc., in water Two forms
that are commonly found in natural water are arsenite
(3
As ), As (III) and arsenate (4), As (V). Arsenic
(V) dominates in oxic water, while arsenic (III) is more
likely to occur in anoxic water [2]. Of both the forms As
(III) is known to be more toxic than As (V) and is pre-
dominant in ground waters [3].
3
O3
AsO
The occurrence of arsenic in natural water is a world-
wide problem. Arsenic pollution has been reported in the
Argentina, Bangladesh, Canada, China, India, Japan,
Mexico, New Zealand, Taiwan and USA. Fifty districts
of Bangladesh and nine in West Bengal (India) have been
reported to have the concentration of arsenic above the
World Health Organization’s arsenic guideline value of
10 mg·L−1 [4,5] in the drinking water.
Prolonged consumption of water containing arsenic is
known to produce long term health effects, including der-
mal changes and respiratory, cardiovascular, gastrointesti-
nal, genotoxic, mutagenic, and carcinogenic effects [6].
Long term exposure to arsenic via drinking water causes
skin, lung, bladder, and kidney cancer, skin thickening (hy-
perkeratosis) neurological disorders, muscular weakness,
loss of appetite, and nausea.
Various methods that have been reported for the re-
moval of arsenic from aqueous solutions include coagu-
lation and flocculation, precipitation, adsorption and ion
exchange, membrane filtration, ozone oxidation, biore-
mediation and electrochemical treatments. Most of these
methods involve production of high arsenic contaminated
sludge [7], high maintenance cost and require relatively
expensive mineral adsorbents which offset performance
and efficiency advantages [8].
Of all the methods adsorption has been most extensi-
vely used as reported by Abhijit and Sunando [9]. As cited
by Deliyanni, Peleka and Matis [10] selective adsorption
utilizing biological materials, mineral oxides, clay min-
erals, zeolites, fly ash, activated carbons, or polymer res-
ins, has generated increasing interest. In the present work,
montmorillonite (a member of the smectite group of the
clay mineral) has been selected as the host material for
the removal of arsenic from aqueous solutions. MMT is
known for its low cost, high surface area, high chemical
stability, high sorption properties and rich intercalation
chemistry. To the best of our knowledge, the best result