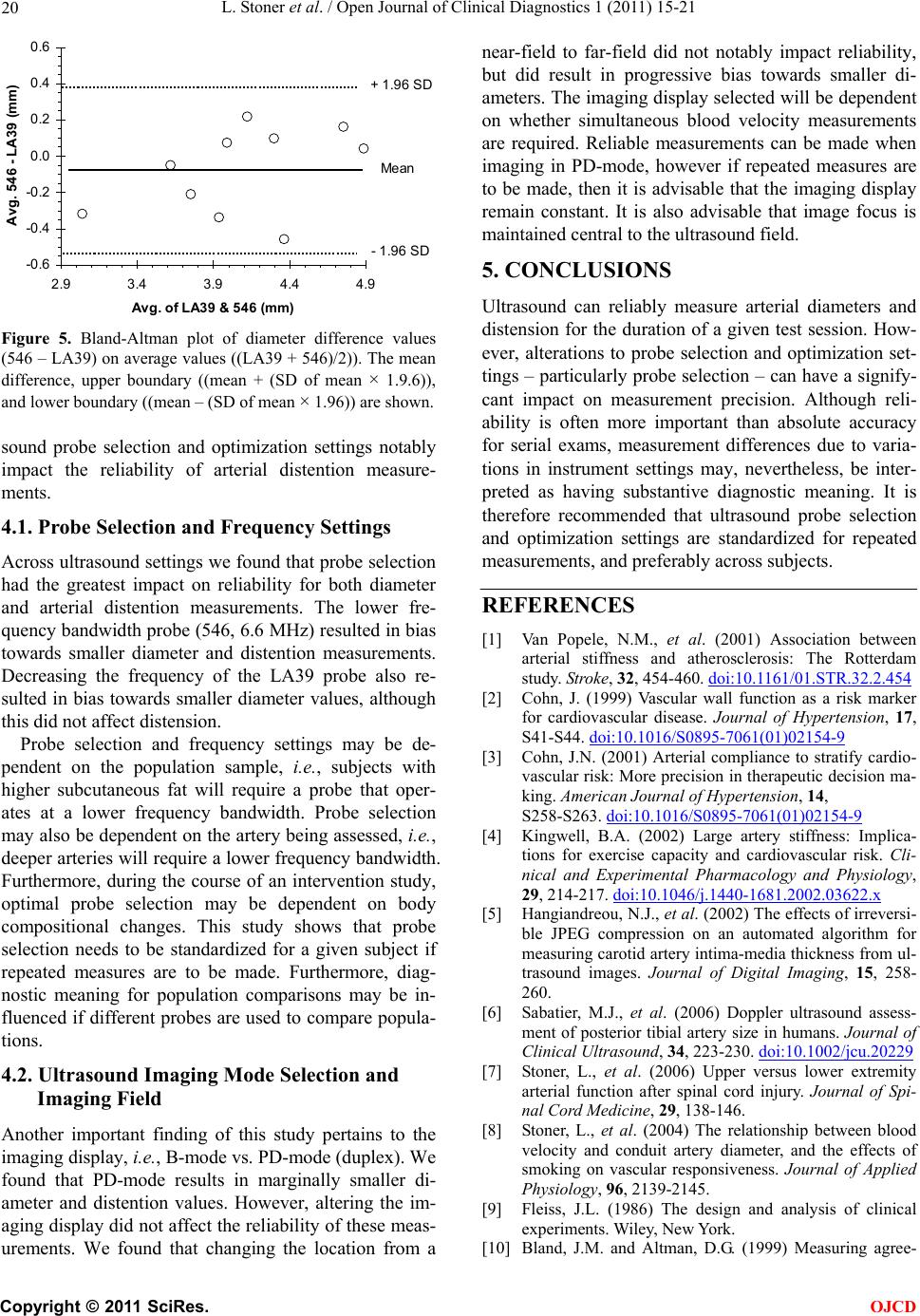
L. Stoner et al. / Open Journal of Clinical Diagnostics 1 (2011) 15-21
20
-0 .6
-0 .4
-0 .2
0.0
0.2
0.4
0.6
2.9 3.4 3.94.4 4.9
Avg. of LA39 & 546 ( m m )
Avg. 54 6 - LA39 (mm)
Mean
- 1.96 SD
+ 1.96 SD
Figure 5. Bland-Altman plot of diameter difference values
(546 – LA39) on average values ((LA39 + 546)/2)). The mean
difference, upper boundary ((mean + (SD of mean × 1.9.6)),
and lower boundary ((mean – (SD of mean × 1.96)) are shown.
sound probe selection and optimization settings notably
impact the reliability of arterial distention measure-
ments.
4.1. Probe Selection and Frequency Settings
Across ultrasound settings we found that probe selection
had the greatest impact on reliability for both diameter
and arterial distention measurements. The lower fre-
quency bandwidth probe (546, 6.6 MHz) resulted in bias
towards smaller diameter and distention measurements.
Decreasing the frequency of the LA39 probe also re-
sulted in bias towards smaller diameter values, although
this did not affect distension.
Probe selection and frequency settings may be de-
pendent on the population sample, i.e., subjects with
higher subcutaneous fat will require a probe that oper-
ates at a lower frequency bandwidth. Probe selection
may also be dependent on the artery being assessed, i.e.,
deeper arteries will require a lower frequency bandwidth.
Furthermore, during the course of an intervention study,
optimal probe selection may be dependent on body
compositional changes. This study shows that probe
selection needs to be standardized for a given subject if
repeated measures are to be made. Furthermore, diag-
nostic meaning for population comparisons may be in-
fluenced if different probes are used to compare popula-
tions.
4.2. Ultrasound Imaging Mode Selection and
Imaging Field
Another important finding of this study pertains to the
imaging display, i.e., B-mode vs. PD-mode (duplex). We
found that PD-mode results in marginally smaller di-
ameter and distention values. However, altering the im-
aging display did not affect the reliability of these meas-
urements. We found that changing the location from a
near-field to far-field did not notably impact reliability,
but did result in progressive bias towards smaller di-
ameters. The imaging display selected will be dependent
on whether simultaneous blood velocity measurements
are required. Reliable measurements can be made when
imaging in PD-mode, however if repeated measures are
to be made, then it is advisable that the imaging display
remain constant. It is also advisable that image focus is
maintained central to the ultrasound field.
5. CONCLUSIONS
Ultrasound can reliably measure arterial diameters and
distension for the duration of a given test session. How-
ever, alterations to probe selection and optimization set-
tings – particularly probe selection – can have a signify-
cant impact on measurement precision. Although reli-
ability is often more important than absolute accuracy
for serial exams, measurement differences due to varia-
tions in instrument settings may, nevertheless, be inter-
preted as having substantive diagnostic meaning. It is
therefore recommended that ultrasound probe selection
and optimization settings are standardized for repeated
measurements, and preferably across subjects.
REFERENCES
[1] Van Popele, N.M., et al. (2001) Association between
arterial stiffness and atherosclerosis: The Rotterdam
study. St roke, 32, 454-460. doi:10.1161/01.STR.32.2.454
[2] Cohn, J. (1999) Vascular wall function as a risk marker
for cardiovascular disease. Journal of Hypertension, 17,
S41-S44. doi:10.1016/S0895-7061(01)02154-9
[3] Cohn, J.N. (2001) Arterial compliance to stratify cardio-
vascular risk: More precision in therapeutic decision ma-
king. American Journal of Hypertension, 14,
S258-S263. doi:10.1016/S0895-7061(01)02154-9
[4] Kingwell, B.A. (2002) Large artery stiffness: Implica-
tions for exercise capacity and cardiovascular risk. Cli-
nical and Experimental Pharmacology and Physiology,
29, 214-217. doi:10.1046/j.1440-1681.2002.03622.x
[5] Hangiandreou, N.J., et al. (2002) The effects of irreversi-
ble JPEG compression on an automated algorithm for
measuring carotid artery intima-media thickness from ul-
trasound images. Journal of Digital Imaging, 15, 258-
260.
[6] Sabatier, M.J., et al. (2006) Doppler ultrasound assess-
ment of posterior tibial artery size in humans. Journal of
Clinical Ultrasound, 34, 223-230. doi:10.1002/jcu.20229
[7] Stoner, L., et al. (2006) Upper versus lower extremity
arterial function after spinal cord injury. Journal of Spi-
nal Cord Medicine, 29, 138-146.
[8] Stoner, L., et al. (2004) The relationship between blood
velocity and conduit artery diameter, and the effects of
smoking on vascular responsiveness. Journal of Applied
Physiology, 96, 2139-2145.
[9] Fleiss, J.L. (1986) The design and analysis of clinical
experiments. Wiley, New York.
[10] Bland, J.M. and Altman, D.G. (1999) Measuring agree-
C
opyright © 2011 SciRes. OJCD