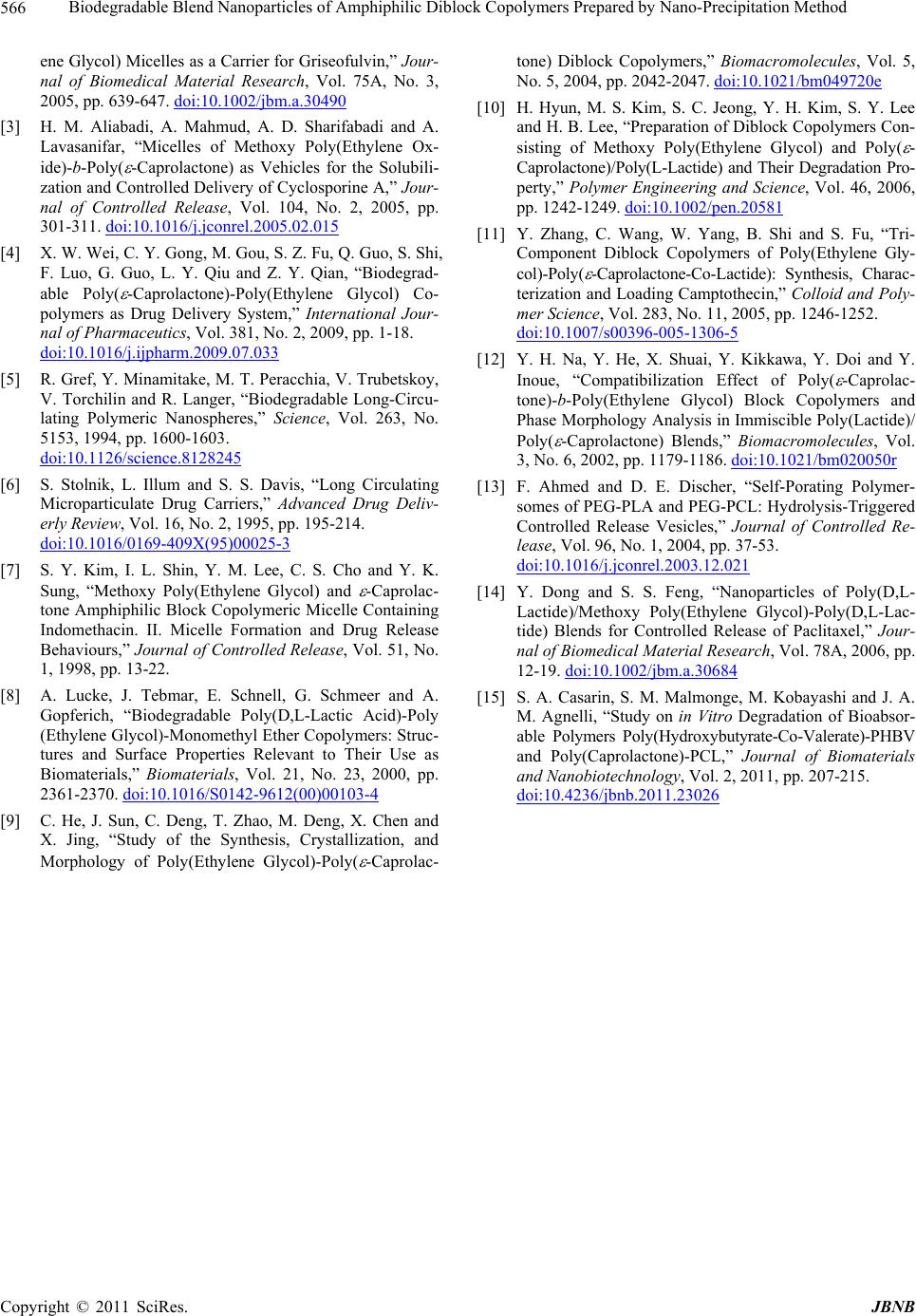
Biodegradable Blend Nanoparticles of Amphiphilic Diblock Copolymers Prepared by Nano-Precipitation Method
Copyright © 2011 SciRes. JBNB
566
ene Glycol) Micelles as a Carrier for Griseofulvin,” Jour-
nal of Biomedical Material Research, Vol. 75A, No. 3,
2005, pp. 639-647. doi:10.1002/jbm.a.30490
[3] H. M. Aliabadi, A. Mahmud, A. D. Sharifabadi and A.
Lavasanifar, “Micelles of Methoxy Poly(Ethylene Ox-
ide)-b-Poly(
-Caprolactone) as Vehicles for the Solubili-
zation and Controlled Delivery of Cy closporine A, ” Jour-
nal of Controlled Release, Vol. 104, No. 2, 2005, pp.
301-311. doi:10.1016/j.jconrel.2005.02.015
[4] X. W. Wei, C. Y. Gong, M. Gou, S. Z. Fu, Q. Guo, S. Shi,
F. Luo, G. Guo, L. Y. Qiu and Z. Y. Qian, “Biodegrad-
able Poly(
-Caprolactone)-Poly(Ethylene Glycol) Co-
polymers as Drug Delivery System,” International Jour-
nal of Pharmaceutics, Vol. 381, No. 2, 2009, pp. 1-18.
doi:10.1016/j.ijpharm.2009.07.033
[5] R. Gref, Y. Minamitake, M. T. Peracchia, V. Trubetskoy,
V. Torchilin and R. Langer, “Biodegradable Long-Circu-
lating Polymeric Nanospheres,” Science, Vol. 263, No.
5153, 1994, pp. 1600-1603.
doi:10.1126/science.8128245
[6] S. Stolnik, L. Illum and S. S. Davis, “Long Circulating
Microparticulate Drug Carriers,” Advanced Drug Deliv-
erly Review, Vol. 16, No. 2, 1995, pp. 195-214.
doi:10.1016/0169-409X(95)00025-3
[7] S. Y. Kim, I. L. Shin, Y. M. Lee, C. S. Cho and Y. K.
Sung, “Methoxy Poly(Ethylene Glycol) and
-Caprolac-
tone Amphiphilic Block Copolymeric Micelle Containing
Indomethacin. II. Micelle Formation and Drug Release
Behaviours,” Journal of Controlled Release, Vol. 51, No.
1, 1998, pp. 13-22.
[8] A. Lucke, J. Tebmar, E. Schnell, G. Schmeer and A.
Gopferich, “Biodegradable Poly(D,L-Lactic Acid)-Poly
(Ethylene Glycol)-Monomethyl Ether Copolymers: Struc-
tures and Surface Properties Relevant to Their Use as
Biomaterials,” Biomaterials, Vol. 21, No. 23, 2000, pp.
2361-2370. doi:10.1016/S0142-9612(00)00103-4
[9] C. He, J. Sun, C. Deng, T. Zhao, M. Deng, X. Chen and
X. Jing, “Study of the Synthesis, Crystallization, and
Morphology of Poly(Ethylene Glycol)-Poly(
-Caprolac-
tone) Diblock Copolymers,” Biomacromolecules, Vol. 5,
No. 5, 2004, pp. 2042-2047. doi:10.1021/bm049720e
[10] H. Hyun, M. S. Kim, S. C. Jeong, Y. H. Kim, S. Y. Lee
and H. B. Lee, “Preparation of Diblock Copolymers Con-
sisting of Methoxy Poly(Ethylene Glycol) and Poly(
-
Caprolactone)/Poly(L-Lactide) and Their Degradation Pro-
perty,” Polymer Engineering and Science, Vol. 46, 2006,
pp. 1242-1249. doi:10.1002/pen.20581
[11] Y. Zhang, C. Wang, W. Yang, B. Shi and S. Fu, “Tri-
Component Diblock Copolymers of Poly(Ethylene Gly-
col)-Poly(
-Caprolactone-Co-Lactide): Synthesis, Charac-
terization and Loading Camptothecin,” Colloid and Poly-
mer Science, Vol. 283, No. 11, 2005, pp. 1246-1252.
doi:10.1007/s00396-005-1306-5
[12] Y. H. Na, Y. He, X. Shuai, Y. Kikkawa, Y. Doi and Y.
Inoue, “Compatibilization Effect of Poly(
-Caprolac-
tone)-b-Poly(Ethylene Glycol) Block Copolymers and
Phase Morphology Analysis in Immiscible Poly(Lactide)/
Poly(
-Caprolactone) Blends,” Biomacromolecules, Vol.
3, No. 6, 2002, pp. 1179-1186. doi:10.1021/bm020050r
[13] F. Ahmed and D. E. Discher, “Self-Porating Polymer-
somes of PEG-PLA and PEG-PCL : Hydrolysis-Triggered
Controlled Release Vesicles,” Journal of Controlled Re-
lease, Vol. 96, No. 1, 2004, pp. 37-53.
doi:10.1016/j.jconrel.2003.12.021
[14] Y. Dong and S. S. Feng, “Nanoparticles of Poly(D,L-
Lactide)/Methoxy Poly(Ethylene Glycol)-Poly(D,L-Lac-
tide) Blends for Controlled Release of Paclitaxel,” Jour-
nal of Biomedical Material Research, Vol. 78A, 2006, pp.
12-19. doi:10.1002/jbm.a.30684
[15] S. A. Casarin, S. M. Malmonge, M. Kobayashi and J. A.
M. Agnelli, “Study on in Vitro Degradation of Bioabsor-
able Polymers Poly(Hydroxybutyrate-Co-Valerate)-PHBV
and Poly(Caprolactone)-PCL,” Journal of Biomaterials
and Nanobiotechnology, Vol. 2, 2011, pp. 207-215.
doi:10.4236/jbnb.2011.23026