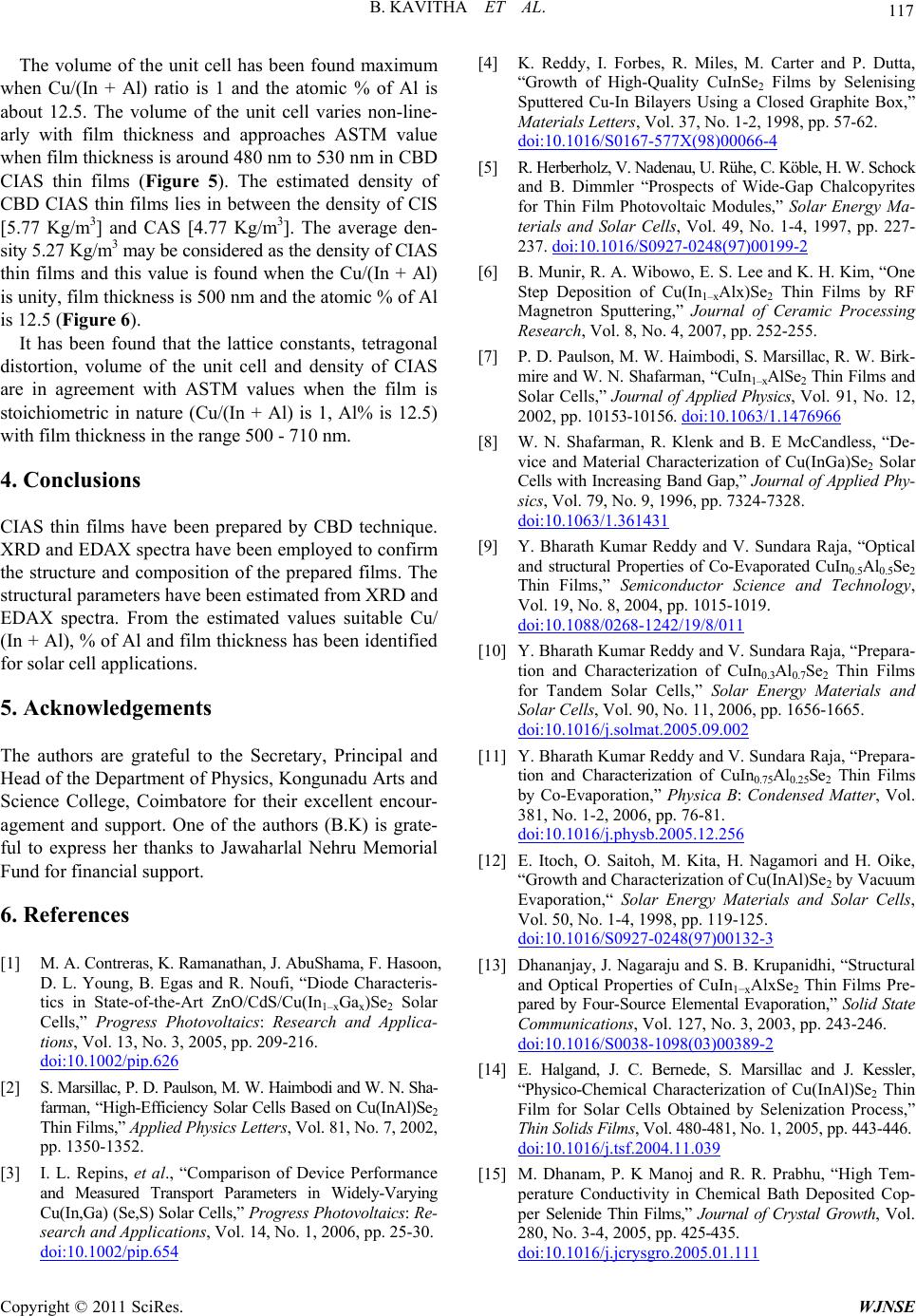
B. KAVITHA ET AL.
Copyright © 2011 SciRes. WJNSE
117
The volume of the unit cell has been found maximum
when Cu/(In + Al) ratio is 1 and the atomic % of Al is
about 12.5. The volume of the unit cell varies non-line-
arly with film thickness and approaches ASTM value
when film thickness is around 480 nm to 530 nm in CBD
CIAS thin films (Figure 5). The estimated density of
CBD CIAS thin films lies in between the density of CIS
[5.77 Kg/m3] and CAS [4.77 Kg/m3]. The average den-
sity 5.27 Kg/m3 may be considered as the density of CIAS
thin films and this value is found when the Cu/(In + Al)
is unity, film thickness is 500 nm and the atomic % of Al
is 12.5 (Figure 6).
It has been found that the lattice constants, tetragonal
distortion, volume of the unit cell and density of CIAS
are in agreement with ASTM values when the film is
stoichiometric in nature (Cu/(In + Al) is 1, Al% is 12.5)
with film thickness in the range 500 - 710 nm.
4. Conclusions
CIAS thin films have been prepared by CBD technique.
XRD and EDAX spectra have been employed to confirm
the structure and composition of the prepared films. The
structural parameters have been estimated from XRD and
EDAX spectra. From the estimated values suitable Cu/
(In + Al), % of Al and film thickness has been identified
for solar cell applications.
5. Acknowledgements
The authors are grateful to the Secretary, Principal and
Head of the Department of Physics, Kongunadu Arts and
Science College, Coimbatore for their excellent encour-
agement and support. One of the authors (B.K) is grate-
ful to express her thanks to Jawaharlal Nehru Memorial
Fund for financial support.
6. References
[1] M. A. Contreras, K. Ramanathan, J. AbuShama, F. Hasoon,
D. L. Young, B. Egas and R. Noufi, “Diode Characteris-
tics in State-of-the-Art ZnO/CdS/Cu(In1–xGax)Se2 Solar
Cells,” Progress Photovoltaics: Research and Applica-
tions, Vol. 13, No. 3, 2005, pp. 209-216.
doi:10.1002/pip.626
[2] S. Marsillac, P. D. Paulson, M. W. Haimbodi and W. N. Sha-
farman, “High-Efficiency Solar Cells Based on Cu(InAl)Se2
Thin Films,” Applied Physics Letters, Vol. 81, No. 7, 2002,
pp. 1350-1352.
[3] I. L. Repins, et al., “Comparison of Device Performance
and Measured Transport Parameters in Widely-Varying
Cu(In,Ga) (Se,S) Solar Cells,” Progress Photovoltaics: Re-
search and Applications, Vol. 14, No. 1, 2006, pp. 25-30.
doi:10.1002/pip.654
[4] K. Reddy, I. Forbes, R. Miles, M. Carter and P. Dutta,
“Growth of High-Quality CuInSe2 Films by Selenising
Sputtered Cu-In Bilayers Using a Closed Graphite Box,”
Materials Letters, Vol. 37, No. 1-2, 1998, pp. 57-62.
doi:10.1016/S0167-577X(98)00066-4
[5] R. Herberholz, V. Nadenau, U. Rühe, C. Köble, H. W. Schock
and B. Dimmler “Prospects of Wide-Gap Chalcopyrites
for Thin Film Photovoltaic Modules,” Solar Energy Ma-
terials and Solar Cells, Vol. 49, No. 1-4, 1997, pp. 227-
237. doi:10.1016/S0927-0248(97)00199-2
[6] B. Munir, R. A. Wibowo, E. S. Lee and K. H. Kim, “One
Step Deposition of Cu(In1–xAlx)Se2 Thin Films by RF
Magnetron Sputtering,” Journal of Ceramic Processing
Research, Vol. 8, No. 4, 2007, pp. 252-255.
[7] P. D. Paulson, M. W. Haimbodi, S. Marsillac, R. W. Birk-
mire and W. N. Shafarman, “CuIn1–xAlSe2 Thin Films and
Solar Cells,” Journal of Applied Physics, Vol. 91, No. 12,
2002, pp. 10153-10156. doi:10.1063/1.1476966
[8] W. N. Shafarman, R. Klenk and B. E McCandless, “De-
vice and Material Characterization of Cu(InGa)Se2 Solar
Cells with Increasing Band Gap,” Journal of Applied Phy-
sics, Vol. 79, No. 9, 1996, pp. 7324-7328.
doi:10.1063/1.361431
[9] Y. Bharath Kumar Reddy and V. Sundara Raja, “Optical
and structural Properties of Co-Evaporated CuIn0.5Al0.5Se2
Thin Films,” Semiconductor Science and Technology,
Vol. 19, No. 8, 2004, pp. 1015-1019.
doi:10.1088/0268-1242/19/8/011
[10] Y. Bharath Kumar Reddy and V. Sundara Raja, “Prepara-
tion and Characterization of CuIn0.3Al0.7Se2 Thin Films
for Tandem Solar Cells,” Solar Energy Materials and
Solar Cells, Vol. 90, No. 11, 2006, pp. 1656-1665.
doi:10.1016/j.solmat.2005.09.002
[11] Y. Bharath Kumar Reddy and V. Sundara Raja, “Prepara-
tion and Characterization of CuIn0.75Al 0.2 5Se 2 Thin Films
by Co-Evaporation,” Physica B: Condensed Matter, Vol.
381, No. 1-2, 2006, pp. 76-81.
doi:10.1016/j.physb.2005.12.256
[12] E. Itoch, O. Saitoh, M. Kita, H. Nagamori and H. Oike,
“Growth and Characterization of Cu(InAl)Se2 by Vacuum
Evaporation,“ Solar Energy Materials and Solar Cells,
Vol. 50, No. 1-4, 1998, pp. 119-125.
doi:10.1016/S0927-0248(97)00132-3
[13] Dhananjay, J. Nagaraju and S. B. Krupanidhi, “Structural
and Optical Properties of CuIn1−xAlxSe2 Thin Films Pre-
pared by Four-Source Elemental Evaporation,” Solid State
Communications, Vol. 127, No. 3, 2003, pp. 243-246.
doi:10.1016/S0038-1098(03)00389-2
[14] E. Halgand, J. C. Bernede, S. Marsillac and J. Kessler,
“Physico-Chemical Characterization of Cu(InAl)Se2 Thin
Film for Solar Cells Obtained by Selenization Process,”
Thin Solids Films, Vol. 480-481, No. 1, 2005, pp. 443-446.
doi:10.1016/j.tsf.2004.11.039
[15] M. Dhanam, P. K Manoj and R. R. Prabhu, “High Tem-
perature Conductivity in Chemical Bath Deposited Cop-
per Selenide Thin Films,” Journal of Crystal Growth, Vol.
280, No. 3-4, 2005, pp. 425-435.
doi:10.1016/j.jcrysgro.2005.01.111