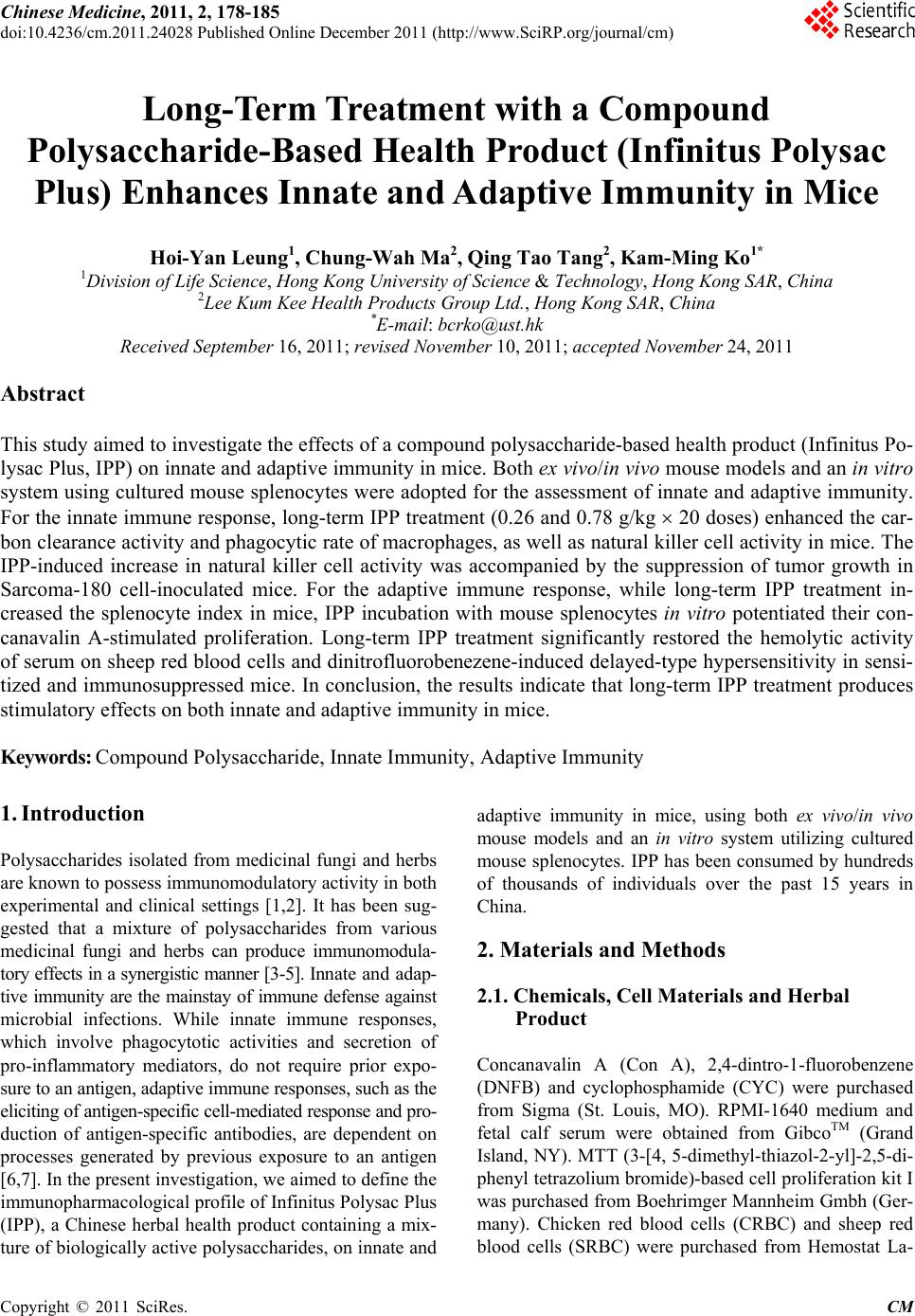 Chinese Medicine, 2011, 2, 178-185 doi:10.4236/cm.2011.24028 Published Online December 2011 (http://www.SciRP.org/journal/cm) Copyright © 2011 SciRes. CM Long-Term Treatment with a Compound Polysaccharide-Based Health Product (Infinitus Polysac Plus) Enhances Innate and Adaptive Immunity in Mice Hoi-Yan Leung1, Chung-Wah Ma2, Qing Tao Tang2, Kam-Ming Ko1* 1Division of Life Science, Hong Kong University of Science & Technology, Hong Kong SAR, China 2Lee Kum Kee Health Products Group Ltd., Hong Kong SAR, China *E-mail: bcrko@ust.hk Received September 16, 2011; revised November 10, 2011; accepted November 24, 2011 Abstract This study aimed to investigate the effects of a compound polysaccharide-based health product (Infinitus Po- lysac Plus, IPP) on innate and adaptive immunity in mice. Both ex vivo/in vivo mouse models and an in vitro system using cultured mouse splenocytes were adopted for the assessment of innate and adaptive immunity. For the innate immune response, long-term IPP treatment (0.26 and 0.78 g/kg 20 doses) enhanced the car- bon clearance activity and phagocytic rate of macrophages, as well as natural killer cell activity in mice. The IPP-induced increase in natural killer cell activity was accompanied by the suppression of tumor growth in Sarcoma-180 cell-inoculated mice. For the adaptive immune response, while long-term IPP treatment in- creased the splenocyte index in mice, IPP incubation with mouse splenocytes in vitro potentiated their con- canavalin A-stimulated proliferation. Long-term IPP treatment significantly restored the hemolytic activity of serum on sheep red blood cells and dinitrofluorobenezene-induced delayed-type hypersensitivity in sensi- tized and immunosuppressed mice. In conclusion, the results indicate that long-term IPP treatment produces stimulatory effects on both innate and adaptive immunity in mice. Keywords: Compound Polysaccharide, Innate Immunity, Adaptive Immunity 1. Introduction Polysaccharides isolated from medicinal fungi and herbs are known to possess immunomodulatory activity in both experimental and clinical settings [1,2]. It has been sug- gested that a mixture of polysaccharides from various medicinal fungi and herbs can produce immunomodula- tory effects in a synergistic manner [3-5]. Innate and adap- tive immunity are the mainstay of immune defense against microbial infections. While innate immune responses, which involve phagocytotic activities and secretion of pro-inflammatory mediators, do not require prior expo- sure to an antigen, adaptive immune responses, such as the eliciting of antigen-specific cell-mediated response and pro- duction of antigen-specific antibodies, are dependent on processes generated by previous exposure to an antigen [6,7]. In the present investigation, we aimed to define the immunopharmacological profile of Infinitus Polysac Plus (IPP), a Chinese herbal health product containing a mix- ture of biologically active polysaccharides, on innate and adaptive immunity in mice, using both ex vivo/in vivo mouse models and an in vitro system utilizing cultured mouse splenocytes. IPP has been consumed by hundreds of thousands of individuals over the past 15 years in China. 2. Materials and Methods 2.1. Chemicals, Cell Materials and Herbal Product Concanavalin A (Con A), 2,4-dintro-1-fluorobenzene (DNFB) and cyclophosphamide (CYC) were purchased from Sigma (St. Louis, MO). RPMI-1640 medium and fetal calf serum were obtained from GibcoTM (Grand Island, NY). MTT (3-[4, 5-dimethyl-thiazol-2-yl]-2,5-di- phenyl tetrazolium bromide)-based cell proliferation kit I was purchased from Boehrimger Mannheim Gmbh (Ger- many). Chicken red blood cells (CRBC) and sheep red blood cells (SRBC) were purchased from Hemostat La-
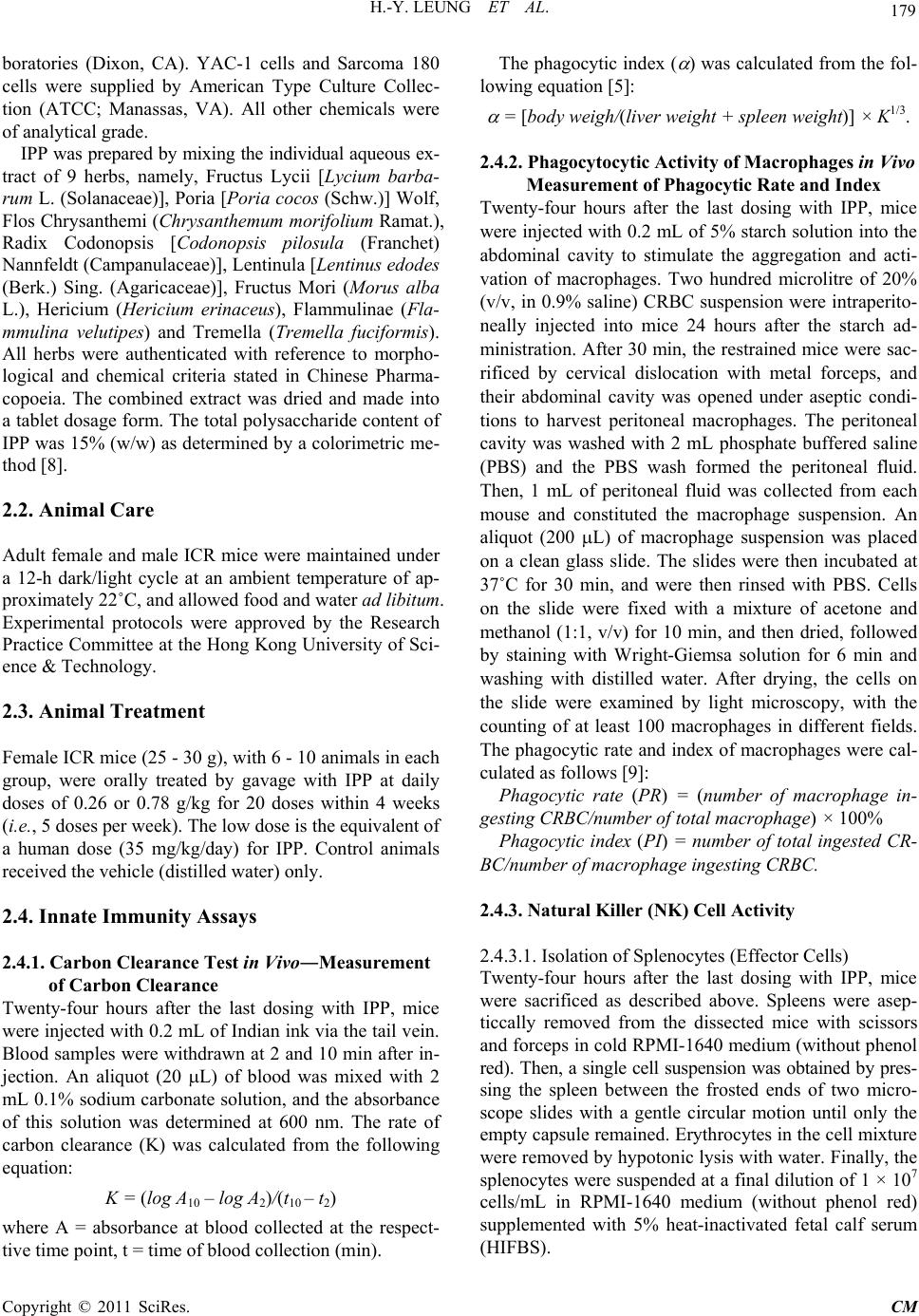 179 H.-Y. LEUNG ET AL. boratories (Dixon, CA). YAC-1 cells and Sarcoma 180 cells were supplied by American Type Culture Collec- tion (ATCC; Manassas, VA). All other chemicals were of analytical grade. IPP was prepared by mixing the individual aqueous ex- tract of 9 herbs, namely, Fructus Lycii [Lycium barba- rum L. (Solanaceae)], Poria [Poria cocos (Schw.)] Wolf, Flos Chrysanthemi (Chrysanthemum morifolium Ramat.), Radix Codonopsis [Codonopsis pilosula (Franchet) Nannfeldt (Campanulaceae)], Lentinula [Lentinus edodes (Berk.) Sing. (Agaricaceae)], Fructus Mori (Morus alba L.), Hericium (Hericium erinaceus), Flammulinae (Fla- mmulina velutipes) and Tremella (Tremella fuciformis). All herbs were authenticated with reference to morpho- logical and chemical criteria stated in Chinese Pharma- copoeia. The combined extract was dried and made into a tablet dosage form. The total polysaccharide content of IPP was 15% (w/w) as determined by a colorimetric me- thod [8]. 2.2. Animal Care Adult female and male ICR mice were maintained under a 12-h dark/light cycle at an ambient temperature of ap- proximately 22˚C, and allowed food and water ad libitum. Experimental protocols were approved by the Research Practice Committee at the Hong Kong University of Sci- ence & Technology. 2.3. Animal Treatment Female ICR mice (25 - 30 g), with 6 - 10 animals in each group, were orally treated by gavage with IPP at daily doses of 0.26 or 0.78 g/kg for 20 doses within 4 weeks (i.e., 5 doses per week). The low dose is the equivalent of a human dose (35 mg/kg/day) for IPP. Control animals received the vehicle (distilled water) only. 2.4. Innate Immunity Assays 2.4.1. Carbon Clearance Test in Vivo―Measurement of Carbon Clearance Twenty-four hours after the last dosing with IPP, mice were injected with 0.2 mL of Indian ink via the tail vein. Blood samples were withdrawn at 2 and 10 min after in- jection. An aliquot (20 L) of blood was mixed with 2 mL 0.1% sodium carbonate solution, and the absorbance of this solution was determined at 600 nm. The rate of carbon clearance (K) was calculated from the following equation: K = (log A10 – log A2)/(t10 – t2) where A = absorbance at blood collected at the respect- tive time point, t = time of blood collection (min). The phagocytic index ( ) was calculated from the fol- lowing equation [5]: = [body weigh/(liver weight + spleen weight)] × K1/3. 2.4.2. Phagocytocytic Activity of Macrophages in Vivo Measurement of Phagocytic Rate and Index Twenty-four hours after the last dosing with IPP, mice were injected with 0.2 mL of 5% starch solution into the abdominal cavity to stimulate the aggregation and acti- vation of macrophages. Two hundred microlitre of 20% (v/v, in 0.9% saline) CRBC suspension were intraperito- neally injected into mice 24 hours after the starch ad- ministration. After 30 min, the restrained mice were sac- rificed by cervical dislocation with metal forceps, and their abdominal cavity was opened under aseptic condi- tions to harvest peritoneal macrophages. The peritoneal cavity was washed with 2 mL phosphate buffered saline (PBS) and the PBS wash formed the peritoneal fluid. Then, 1 mL of peritoneal fluid was collected from each mouse and constituted the macrophage suspension. An aliquot (200 L) of macrophage suspension was placed on a clean glass slide. The slides were then incubated at 37˚C for 30 min, and were then rinsed with PBS. Cells on the slide were fixed with a mixture of acetone and methanol (1:1, v/v) for 10 min, and then dried, followed by staining with Wright-Giemsa solution for 6 min and washing with distilled water. After drying, the cells on the slide were examined by light microscopy, with the counting of at least 100 macrophages in different fields. The phagocytic rate and index of macrophages were cal- culated as follows [9]: Phagocytic rate (PR) = (number of macrophage in- gesting CRBC/number of total macrophage) × 100% Phagocytic index (PI) = number of total ingested CR- BC/number of macr oph a ge in gest in g CRBC. 2.4.3. Natural Killer (NK) Cell Activity 2.4.3.1. Isolation of Splenocytes (Effector Cells) Twenty-four hours after the last dosing with IPP, mice were sacrificed as described above. Spleens were asep- ticcally removed from the dissected mice with scissors and forceps in cold RPMI-1640 medium (without phenol red). Then, a single cell suspension was obtained by pres- sing the spleen between the frosted ends of two micro- scope slides with a gentle circular motion until only the empty capsule remained. Erythrocytes in the cell mixture were removed by hypotonic lysis with water. Finally, the splenocytes were suspended at a final dilution of 1 × 107 cells/mL in RPMI-1640 medium (without phenol red) supplemented with 5% heat-inactivated fetal calf serum (HIFBS). Copyright © 2011 SciRes. CM
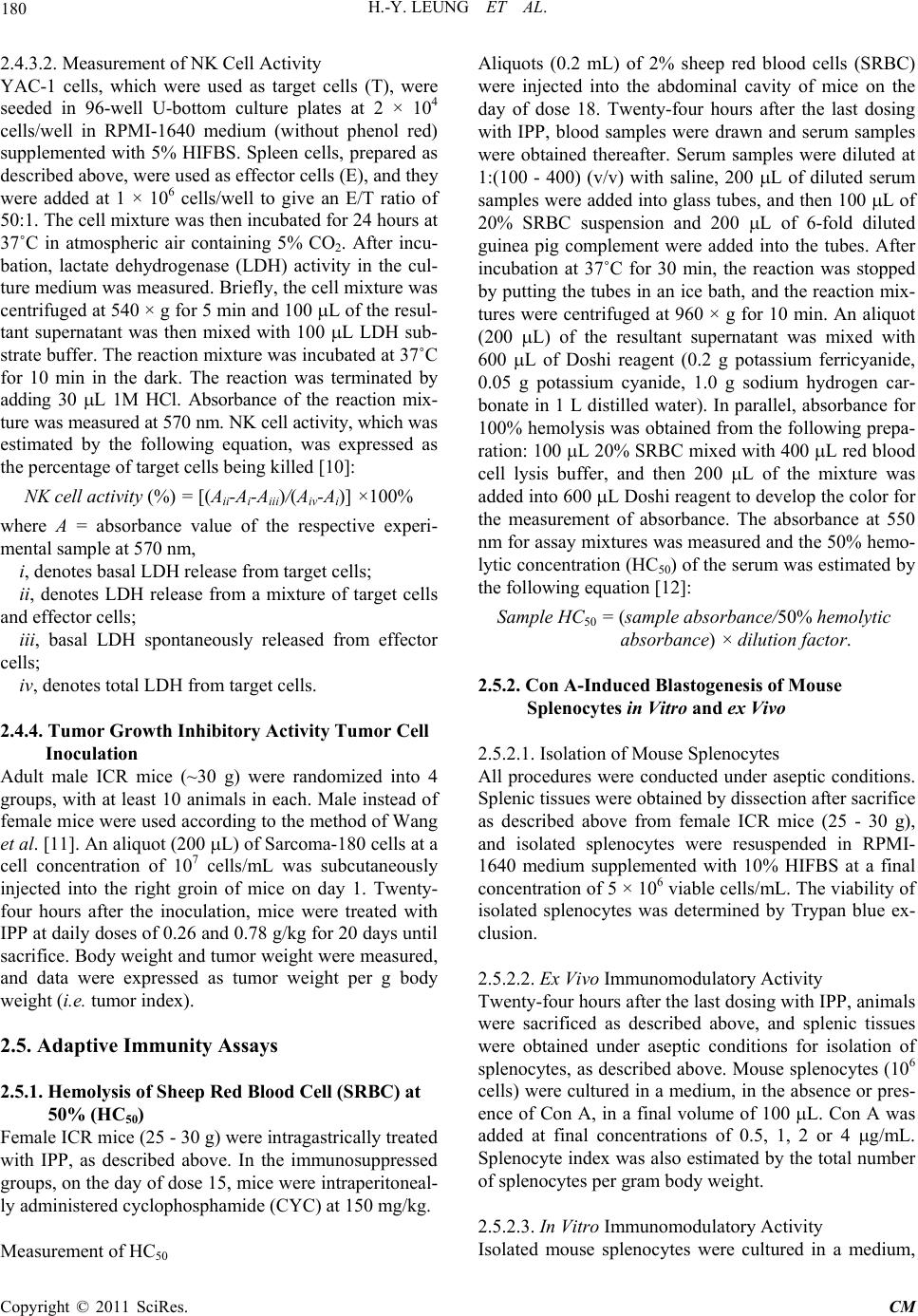 H.-Y. LEUNG ET AL. 180 2.4.3.2. Measurement of NK Cell Activity YAC-1 cells, which were used as target cells (T), were seeded in 96-well U-bottom culture plates at 2 × 104 cells/well in RPMI-1640 medium (without phenol red) supplemented with 5% HIFBS. Spleen cells, prepared as described above, were used as effector cells (E), and they were added at 1 × 106 cells/well to give an E/T ratio of 50:1. The cell mixture was then incubated for 24 hours at 37˚C in atmospheric air containing 5% CO2. After incu- bation, lactate dehydrogenase (LDH) activity in the cul- ture medium was measured. Briefly, the cell mixture was centrifuged at 540 × g for 5 min and 100 L of the resul- tant supernatant was then mixed with 100 L LDH sub- strate buffer. The reaction mixture was incubated at 37˚C for 10 min in the dark. The reaction was terminated by adding 30 L 1M HCl. Absorbance of the reaction mix- ture was measured at 570 nm. NK cell activity, which was estimated by the following equation, was expressed as the percentage of target cells being killed [10]: NK cell activity (%) = [(Aii-Ai-Aiii)/(Aiv-Ai)] ×100% where A = absorbance value of the respective experi- mental sample at 570 nm, i, denotes basal LDH release from target cells; ii, denotes LDH release from a mixture of target cells and effector cells; iii, basal LDH spontaneously released from effector cells; iv, denotes total LDH from target cells. 2.4.4. Tumor Growth Inhibitory Activity Tumor Cell Inoculation Adult male ICR mice (~30 g) were randomized into 4 groups, with at least 10 animals in each. Male instead of female mice were used according to the method of Wang et al. [11]. An aliquot (200 L) of Sarcoma-180 cells at a cell concentration of 107 cells/mL was subcutaneously injected into the right groin of mice on day 1. Twenty- four hours after the inoculation, mice were treated with IPP at daily doses of 0.26 and 0.78 g/kg for 20 days until sacrifice. Body weight and tumor weight were measured, and data were expressed as tumor weight per g body weight (i.e. tumor index). 2.5. Adaptive Immunity Assays 2.5.1. Hemolysis of Sheep Red Blood Cell (SRBC) at 50% (HC50) Female ICR mice (25 - 30 g) were intragastrically treated with IPP, as described above. In the immunosuppressed groups, on the day of dose 15, mice were intraperitoneal- ly administered cyclophosphamide (CYC) at 150 mg/kg. Measurement of HC50 Aliquots (0.2 mL) of 2% sheep red blood cells (SRBC) were injected into the abdominal cavity of mice on the day of dose 18. Twenty-four hours after the last dosing with IPP, blood samples were drawn and serum samples were obtained thereafter. Serum samples were diluted at 1:(100 - 400) (v/v) with saline, 200 L of diluted serum samples were added into glass tubes, and then 100 L of 20% SRBC suspension and 200 L of 6-fold diluted guinea pig complement were added into the tubes. After incubation at 37˚C for 30 min, the reaction was stopped by putting the tubes in an ice bath, and the reaction mix- tures were centrifuged at 960 × g for 10 min. An aliquot (200 L) of the resultant supernatant was mixed with 600 L of Doshi reagent (0.2 g potassium ferricyanide, 0.05 g potassium cyanide, 1.0 g sodium hydrogen car- bonate in 1 L distilled water). In parallel, absorbance for 100% hemolysis was obtained from the following prepa- ration: 100 L 20% SRBC mixed with 400 L red blood cell lysis buffer, and then 200 L of the mixture was added into 600 L Doshi reagent to develop the color for the measurement of absorbance. The absorbance at 550 nm for assay mixtures was measured and the 50% hemo- lytic concentration (HC50) of the serum was estimated by the following equation [12]: Sample HC50 = (sample absorbance/50% hemolytic absorbance) × dilution factor. 2.5.2. Con A-Induced Blastogenesis of Mouse Splenocytes in Vitro and ex Vivo 2.5.2.1. Isolation of Mouse Splenocytes All procedures were conducted under aseptic conditions. Splenic tissues were obtained by dissection after sacrifice as described above from female ICR mice (25 - 30 g), and isolated splenocytes were resuspended in RPMI- 1640 medium supplemented with 10% HIFBS at a final concentration of 5 × 106 viable cells/mL. The viability of isolated splenocytes was determined by Trypan blue ex- clusion. 2.5.2.2. Ex Vivo Immunomodulatory Activity Twenty-four hours after the last dosing with IPP, animals were sacrificed as described above, and splenic tissues were obtained under aseptic conditions for isolation of splenocytes, as described above. Mouse splenocytes (106 cells) were cultured in a medium, in the absence or pres- ence of Con A, in a final volume of 100 L. Con A was added at final concentrations of 0.5, 1, 2 or 4 g/mL. Splenocyte index was also estimated by the total number of splenocytes per gram body weight. 2.5.2.3. In Vitro Immunomodulatory Activity Isolated mouse splenocytes were cultured in a medium, Copyright © 2011 SciRes. CM
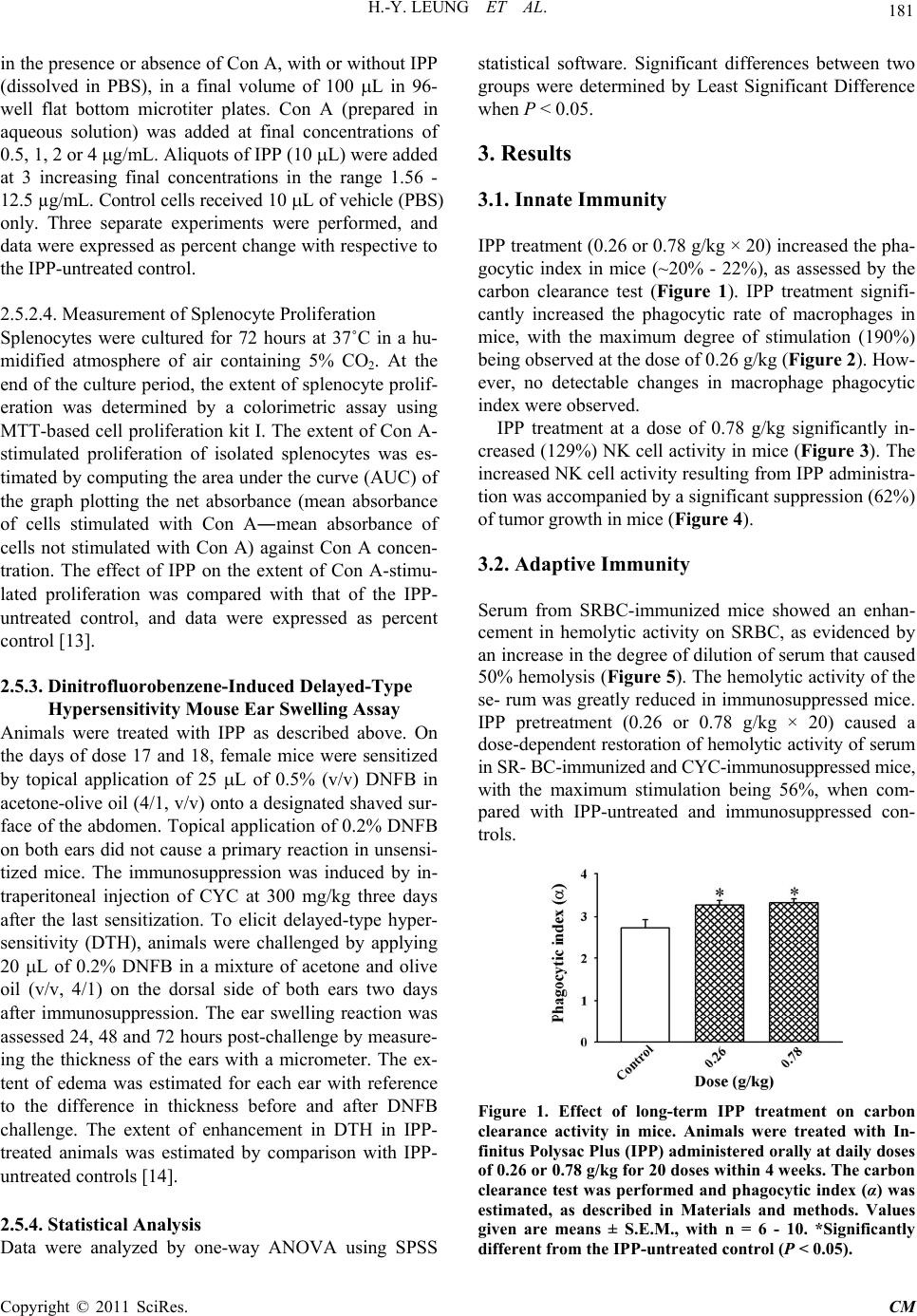 181 H.-Y. LEUNG ET AL. in the presence or absence of Con A, with or without IPP (dissolved in PBS), in a final volume of 100 L in 96- well flat bottom microtiter plates. Con A (prepared in aqueous solution) was added at final concentrations of 0.5, 1, 2 or 4 g/mL. Aliquots of IPP (10 L) were added at 3 increasing final concentrations in the range 1.56 - 12.5 µg/mL. Control cells received 10 L of vehicle (PBS) only. Three separate experiments were performed, and data were expressed as percent change with respective to the IPP-untreated control. 2.5.2.4. Measurement of Splenocyte Proliferation Splenocytes were cultured for 72 hours at 37˚C in a hu- midified atmosphere of air containing 5% CO2. At the end of the culture period, the extent of splenocyte prolif- eration was determined by a colorimetric assay using MTT-based cell proliferation kit I. The extent of Con A- stimulated proliferation of isolated splenocytes was es- timated by computing the area under the curve (AUC) of the graph plotting the net absorbance (mean absorbance of cells stimulated with Con A―mean absorbance of cells not stimulated with Con A) against Con A concen- tration. The effect of IPP on the extent of Con A-stimu- lated proliferation was compared with that of the IPP- untreated control, and data were expressed as percent control [13]. 2.5.3. Dinitrofluorobenzene-Induced Delayed-Type Hypersensitivity Mouse Ear Swelling Assay Animals were treated with IPP as described above. On the days of dose 17 and 18, female mice were sensitized by topical application of 25 L of 0.5% (v/v) DNFB in acetone-olive oil (4/1, v/v) onto a designated shaved sur- face of the abdomen. Topical application of 0.2% DNFB on both ears did not cause a primary reaction in unsensi- tized mice. The immunosuppression was induced by in- traperitoneal injection of CYC at 300 mg/kg three days after the last sensitization. To elicit delayed-type hyper- sensitivity (DTH), animals were challenged by applying 20 L of 0.2% DNFB in a mixture of acetone and olive oil (v/v, 4/1) on the dorsal side of both ears two days after immunosuppression. The ear swelling reaction was assessed 24, 48 and 72 hours post-challenge by measure- ing the thickness of the ears with a micrometer. The ex- tent of edema was estimated for each ear with reference to the difference in thickness before and after DNFB challenge. The extent of enhancement in DTH in IPP- treated animals was estimated by comparison with IPP- untreated controls [14]. 2.5.4. Statistical Analysis Data were analyzed by one-way ANOVA using SPSS statistical software. Significant differences between two groups were determined by Least Significant Difference when P < 0.05. 3. Results 3.1. Innate Immunity IPP treatment (0.26 or 0.78 g/kg × 20) increased the pha- gocytic index in mice (~20% - 22%), as assessed by the carbon clearance test (Figure 1). IPP treatment signifi- cantly increased the phagocytic rate of macrophages in mice, with the maximum degree of stimulation (190%) being observed at the dose of 0.26 g/kg (Figure 2). How- ever, no detectable changes in macrophage phagocytic index were observed. IPP treatment at a dose of 0.78 g/kg significantly in- creased (129%) NK cell activity in mice (Figure 3). The increased NK cell activity resulting from IPP administra- tion was accompanied by a significant suppression (62%) of tumor growth in mice (Figure 4). 3.2. Adaptive Immunity Serum from SRBC-immunized mice showed an enhan- cement in hemolytic activity on SRBC, as evidenced by an increase in the degree of dilution of serum that caused 50% hemolysis (Figure 5). The hemolytic activity of the se- rum was greatly reduced in immunosuppressed mice. IPP pretreatment (0.26 or 0.78 g/kg × 20) caused a dose-dependent restoration of hemolytic activity of serum in SR- BC-immunized and CYC-immunosuppressed mice, with the maximum stimulation being 56%, when com- pared with IPP-untreated and immunosuppressed con- trols. Figure 1. Effect of long-term IPP treatment on carbon clearance activity in mice. Animals were treated with In- finitus Polysac Plus (IPP) administered orally at daily doses of 0.26 or 0.78 g/kg for 20 doses within 4 weeks. The carbon clearance test was performed and phagocytic index (α) was estimated, as described in Materials and methods. Values given are means ± S.E.M., with n = 6 - 10. *Significantly different from the IPP-untreated control (P < 0.05). Copyright © 2011 SciRes. CM
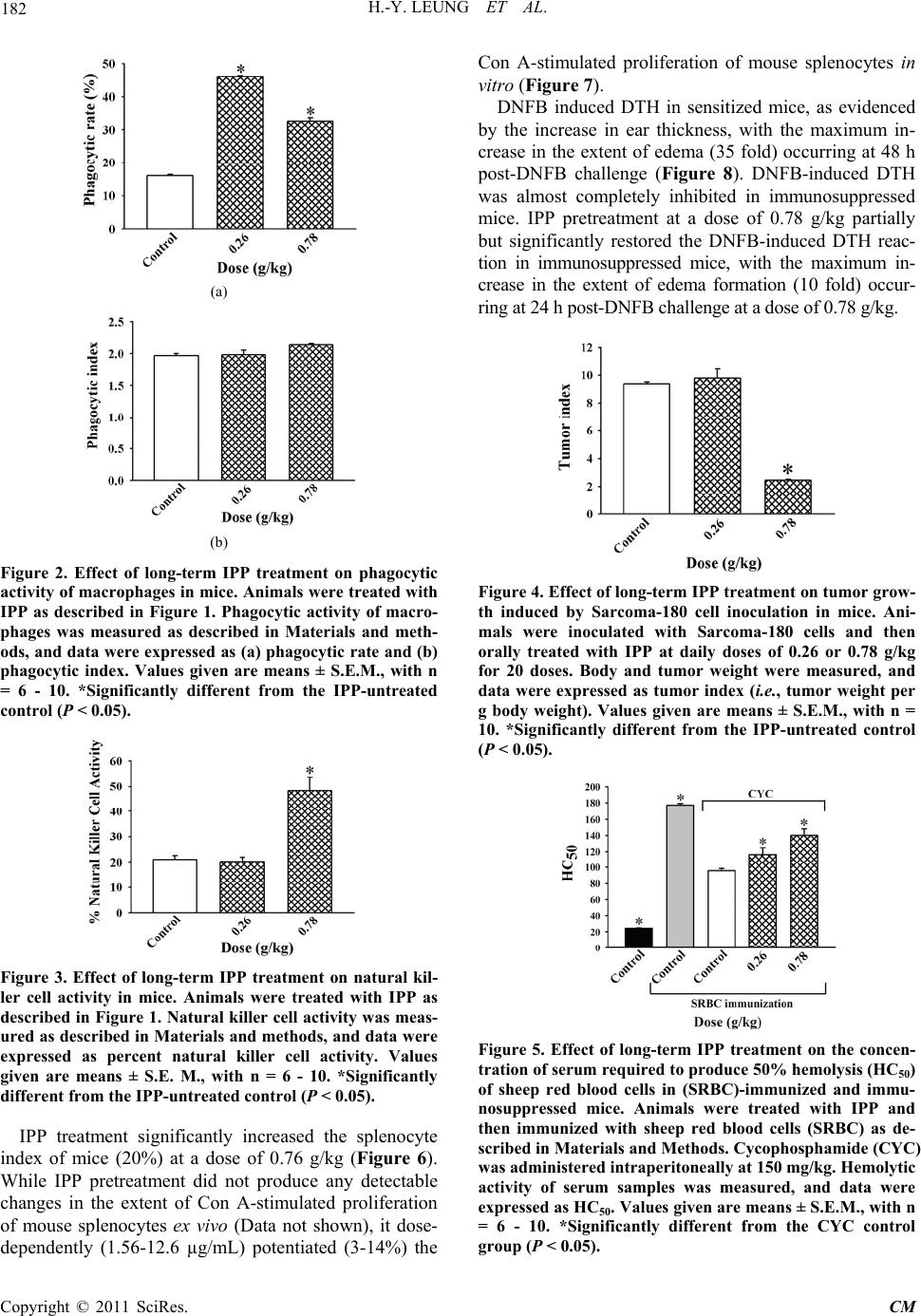 H.-Y. LEUNG ET AL. 182 (a) (b) Figure 2. Effect of long-term IPP treatment on phagocytic activity of macrophages in mice. Animals were treated with IPP as described in Figure 1. Phagocytic activity of macro- phages was measured as described in Materials and meth- ods, and data were expressed as (a) phagocytic rate and (b) phagocytic index. Values given are means ± S.E.M., with n = 6 - 10. *Significantly different from the IPP-untreated control (P < 0.05). Figure 3. Effect of long-term IPP treatment on natural kil- ler cell activity in mice. Animals were treated with IPP as described in Figure 1. Natural killer cell activity was meas- ured as described in Materials and methods, and data were expressed as percent natural killer cell activity. Values given are means ± S.E. M., with n = 6 - 10. *Significantly different from the IPP-untreated control (P < 0.05). IPP treatment significantly increased the splenocyte index of mice (20%) at a dose of 0.76 g/kg (Figure 6). While IPP pretreatment did not produce any detectable changes in the extent of Con A-stimulated proliferation of mouse splenocytes ex vivo (Data not shown), it dose- dependently (1.56-12.6 µg/mL) potentiated (3-14%) the Con A-stimulated proliferation of mouse splenocytes in vitro (Figure 7). DNFB induced DTH in sensitized mice, as evidenced by the increase in ear thickness, with the maximum in- crease in the extent of edema (35 fold) occurring at 48 h post-DNFB challenge (Figure 8). DNFB-induced DTH was almost completely inhibited in immunosuppressed mice. IPP pretreatment at a dose of 0.78 g/kg partially but significantly restored the DNFB-induced DTH reac- tion in immunosuppressed mice, with the maximum in- crease in the extent of edema formation (10 fold) occur- ring at 24 h post-DNFB challenge at a dose of 0.78 g/kg. Figure 4. Effect of long-term IPP treatment on tumor grow- th induced by Sarcoma-180 cell inoculation in mice. Ani- mals were inoculated with Sarcoma-180 cells and then orally treated with IPP at daily doses of 0.26 or 0.78 g/kg for 20 doses. Body and tumor weight were measured, and data were expressed as tumor index (i.e., tumor weight per g body weight). Values given are means ± S.E.M., with n = 10. *Significantly different from the IPP-untreated control (P < 0.05). Figure 5. Effect of long-term IPP treatment on the concen- tration of serum required to produce 50% hemolysis (HC50) of sheep red blood cells in (SRBC)-immunized and immu- nosuppressed mice. Animals were treated with IPP and then immunized with sheep red blood cells (SRBC) as de- scribed in Materials and Methods. Cycophosphamide (CYC) was administered intraperitoneally at 150 mg/kg. Hemolytic activity of serum samples was measured, and data were expressed as HC50. Values given are means ± S.E.M., with n = 6 - 10. *Significantly different from the CYC control group (P < 0.05). Copyright © 2011 SciRes. CM
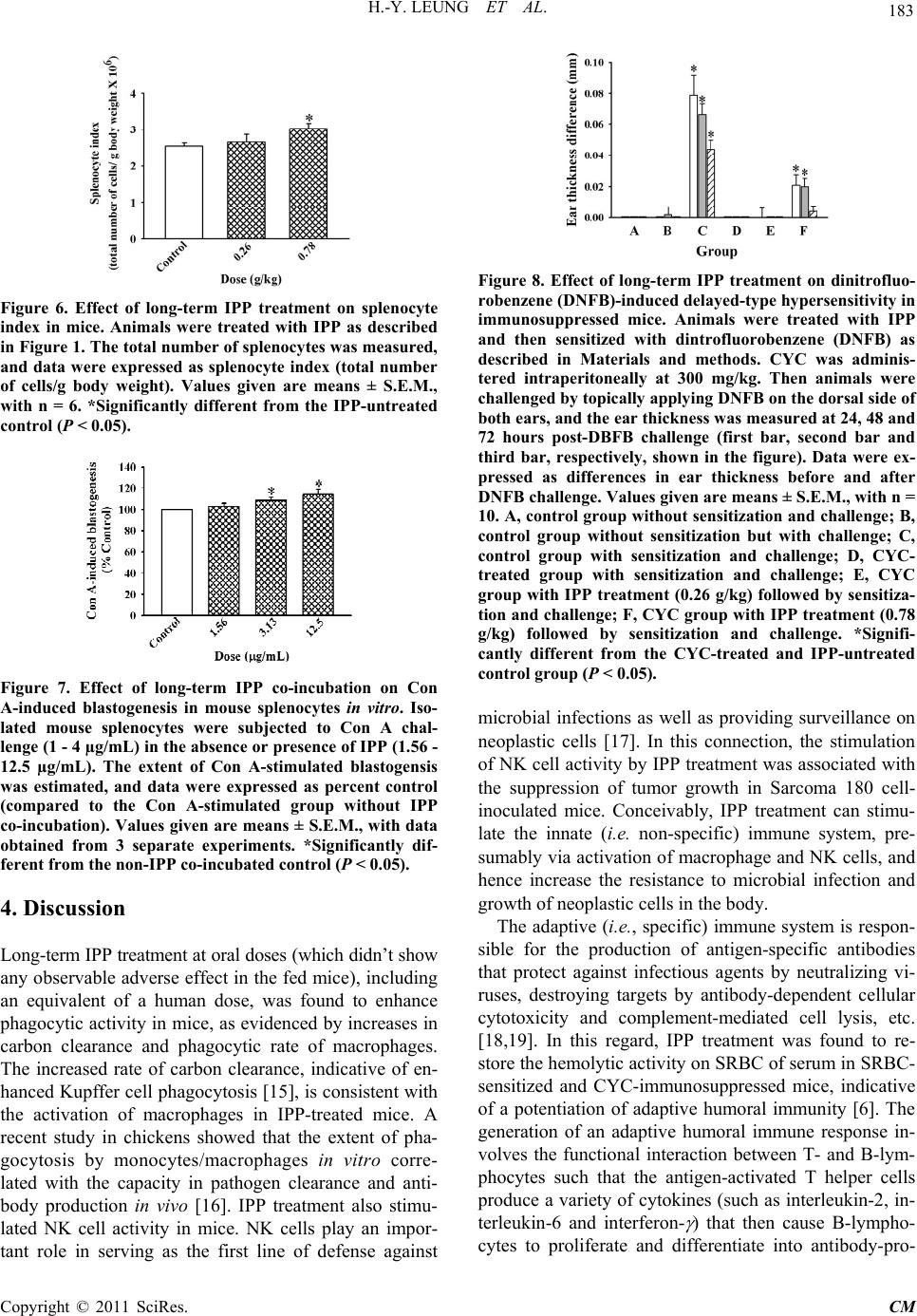 183 H.-Y. LEUNG ET AL. Figure 6. Effect of long-term IPP treatment on splenocyte index in mice. Animals were treated with IPP as described in Figure 1. The total number of splenocytes was measured, and data were expressed as splenocyte index (total number of cells/g body weight). Values given are means ± S.E.M., with n = 6. *Significantly different from the IPP-untreated control (P < 0.05). Figure 7. Effect of long-term IPP co-incubation on Con A-induced blastogenesis in mouse splenocytes in vitro. Iso- lated mouse splenocytes were subjected to Con A chal- lenge (1 - 4 µg/mL) in the absence or presence of IPP (1.56 - 12.5 µg/mL). The extent of Con A-stimulated blastogensis was estimated, and data were expressed as percent control (compared to the Con A-stimulated group without IPP co-incubation). Values given are means ± S.E.M., with data obtained from 3 separate experiments. *Significantly dif- ferent from the non-IPP co-incubated control (P < 0.05). 4. Discussion Long-term IPP treatment at oral doses (which didn’t show any observable adverse effect in the fed mice), including an equivalent of a human dose, was found to enhance phagocytic activity in mice, as evidenced by increases in carbon clearance and phagocytic rate of macrophages. The increased rate of carbon clearance, indicative of en- hanced Kupffer cell phagocytosis [15], is consistent with the activation of macrophages in IPP-treated mice. A recent study in chickens showed that the extent of pha- gocytosis by monocytes/macrophages in vitro corre- lated with the capacity in pathogen clearance and anti- body production in vivo [16]. IPP treatment also stimu- lated NK cell activity in mice. NK cells play an impor- tant role in serving as the first line of defense against Figure 8. Effect of long-term IPP treatment on dinitrofluo- robenzene (DNFB)-induced delayed-type hypersensitivity in immunosuppressed mice. Animals were treated with IPP and then sensitized with dintrofluorobenzene (DNFB) as described in Materials and methods. CYC was adminis- tered intraperitoneally at 300 mg/kg. Then animals were challenged by topically applying DNFB on the dorsal side of both ears, and the ear thickness was measured at 24, 48 and 72 hours post-DBFB challenge (first bar, second bar and third bar, respectively, shown in the figure). Data were ex- pressed as differences in ear thickness before and after DNFB challenge. Values given are means ± S.E.M., with n = 10. A, control group without sensitization and challenge; B, control group without sensitization but with challenge; C, control group with sensitization and challenge; D, CYC- treated group with sensitization and challenge; E, CYC group with IPP treatment (0.26 g/kg) followed by sensitiza- tion and challenge; F, CYC group with IPP treatment (0.78 g/kg) followed by sensitization and challenge. *Signifi- cantly different from the CYC-treated and IPP-untreated control group (P < 0.05). microbial infections as well as providing surveillance on neoplastic cells [17]. In this connection, the stimulation of NK cell activity by IPP treatment was associated with the suppression of tumor growth in Sarcoma 180 cell- inoculated mice. Conceivably, IPP treatment can stimu- late the innate (i.e. non-specific) immune system, pre- sumably via activation of macrophage and NK cells, and hence increase the resistance to microbial infection and growth of neoplastic cells in the body. The adaptive (i.e., specific) immune system is respon- sible for the production of antigen-specific antibodies that protect against infectious agents by neutralizing vi- ruses, destroying targets by antibody-dependent cellular cytotoxicity and complement-mediated cell lysis, etc. [18,19]. In this regard, IPP treatment was found to re- store the hemolytic activity on SRBC of serum in SRBC- sensitized and CYC-immunosuppressed mice, indicative of a potentiation of adaptive humoral immunity [6]. The generation of an adaptive humoral immune response in- volves the functional interaction between T- and B-lym- phocytes such that the antigen-activated T helper cells produce a variety of cytokines (such as interleukin-2, in- terleukin-6 and interferon- ) that then cause B-lympho- cytes to proliferate and differentiate into antibody-pro- Copyright © 2011 SciRes. CM
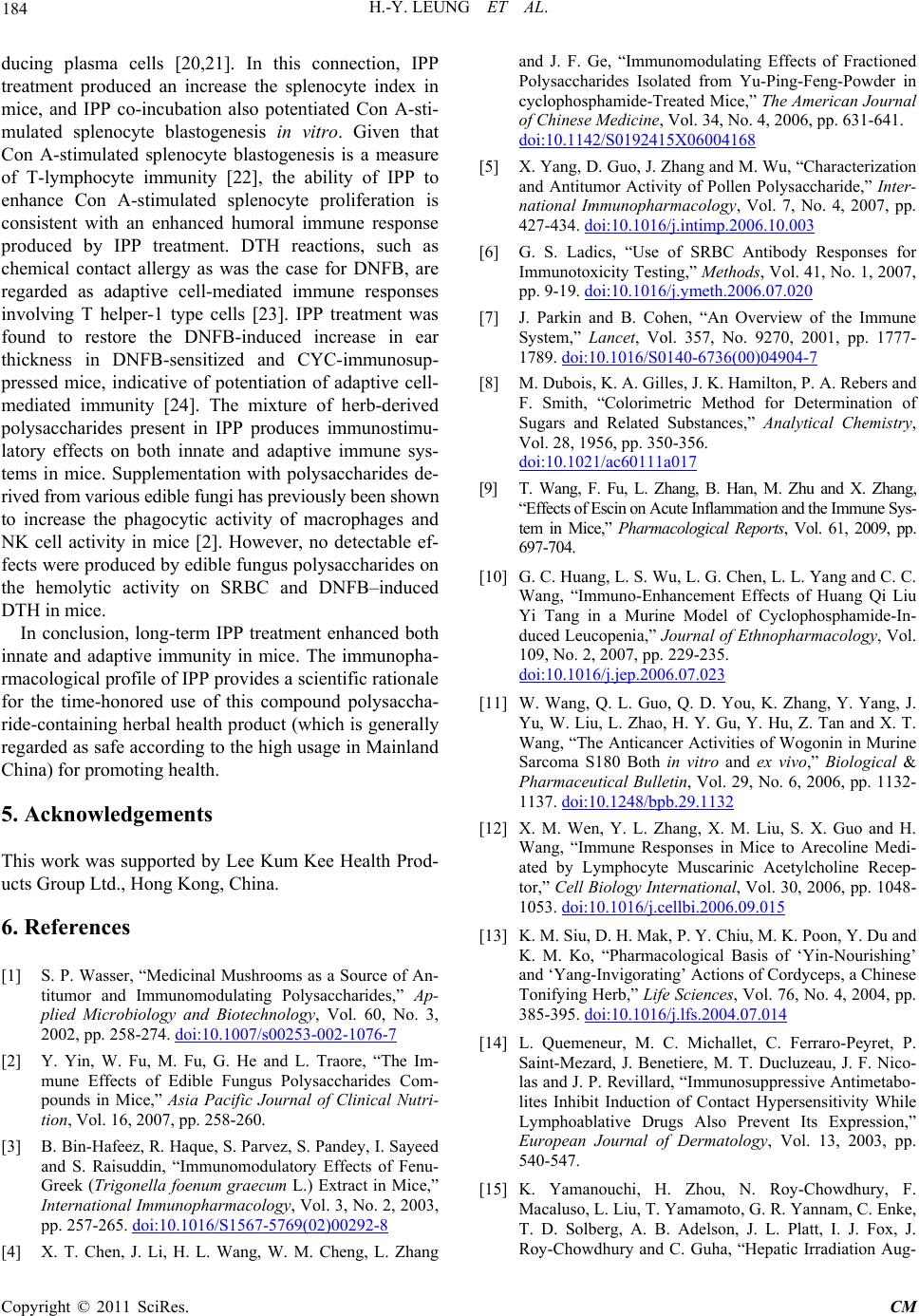 H.-Y. LEUNG ET AL. 184 ducing plasma cells [20,21]. In this connection, IPP treatment produced an increase the splenocyte index in mice, and IPP co-incubation also potentiated Con A-sti- mulated splenocyte blastogenesis in vitro. Given that Con A-stimulated splenocyte blastogenesis is a measure of T-lymphocyte immunity [22], the ability of IPP to enhance Con A-stimulated splenocyte proliferation is consistent with an enhanced humoral immune response produced by IPP treatment. DTH reactions, such as chemical contact allergy as was the case for DNFB, are regarded as adaptive cell-mediated immune responses involving T helper-1 type cells [23]. IPP treatment was found to restore the DNFB-induced increase in ear thickness in DNFB-sensitized and CYC-immunosup- pressed mice, indicative of potentiation of adaptive cell- mediated immunity [24]. The mixture of herb-derived polysaccharides present in IPP produces immunostimu- latory effects on both innate and adaptive immune sys- tems in mice. Supplementation with polysaccharides de- rived from various edible fungi has previously been shown to increase the phagocytic activity of macrophages and NK cell activity in mice [2]. However, no detectable ef- fects were produced by edible fungus polysaccharides on the hemolytic activity on SRBC and DNFB–induced DTH in mice. In conclusion, long-term IPP treatment enhanced both innate and adaptive immunity in mice. The immunopha- rmacological profile of IPP provides a scientific rationale for the time-honored use of this compound polysaccha- ride-containing herbal health product (which is generally regarded as safe according to the high usage in Mainland China) for promoting health. 5. Acknowledgements This work was supported by Lee Kum Kee Health Prod- ucts Group Ltd., Hong Kong, China. 6. References [1] S. P. Wasser, “Medicinal Mushrooms as a Source of An- titumor and Immunomodulating Polysaccharides,” Ap- plied Microbiology and Biotechnology, Vol. 60, No. 3, 2002, pp. 258-274. doi:10.1007/s00253-002-1076-7 [2] Y. Yin, W. Fu, M. Fu, G. He and L. Traore, “The Im- mune Effects of Edible Fungus Polysaccharides Com- pounds in Mice,” Asia Pacific Journal of Clinical Nutri- tion, Vol. 16, 2007, pp. 258-260. [3] B. Bin-Hafeez, R. Haque, S. Parvez, S. Pandey, I. Sayeed and S. Raisuddin, “Immunomodulatory Effects of Fenu- Greek (Trigonella foenum graecum L.) Extract in Mice,” International Immunopharmacology, Vol. 3, No. 2, 2003, pp. 257-265. doi:10.1016/S1567-5769(02)00292-8 [4] X. T. Chen, J. Li, H. L. Wang, W. M. Cheng, L. Zhang and J. F. Ge, “Immunomodulating Effects of Fractioned Polysaccharides Isolated from Yu-Ping-Feng-Powder in cyclophosphamide-Treated Mice,” The American Journal of Chinese Medicine, Vol. 34, No. 4, 2006, pp. 631-641. doi:10.1142/S0192415X06004168 [5] X. Yang, D. Guo, J. Zhang and M. Wu, “Characterization and Antitumor Activity of Pollen Polysaccharide,” Inter- national Immunopharmacology, Vol. 7, No. 4, 2007, pp. 427-434. doi:10.1016/j.intimp.2006.10.003 [6] G. S. Ladics, “Use of SRBC Antibody Responses for Immunotoxicity Testing,” Meth ods, Vol. 41, No. 1, 2007, pp. 9-19. doi:10.1016/j.ymeth.2006.07.020 [7] J. Parkin and B. Cohen, “An Overview of the Immune System,” Lancet, Vol. 357, No. 9270, 2001, pp. 1777- 1789. doi:10.1016/S0140-6736(00)04904-7 [8] M. Dubois, K. A. Gilles, J. K. Hamilton, P. A. Rebers and F. Smith, “Colorimetric Method for Determination of Sugars and Related Substances,” Analytical Chemistry, Vol. 28, 1956, pp. 350-356. doi:10.1021/ac60111a017 [9] T. Wang, F. Fu, L. Zhang, B. Han, M. Zhu and X. Zhang, “Effects of Escin on Acute Inflammation and the Immune Sys- tem in Mice,” Pharmacological Reports, Vol. 61, 2009, pp. 697-704. [10] G. C. Huang, L. S. Wu, L. G. Chen, L. L. Yang and C. C. Wang, “Immuno-Enhancement Effects of Huang Qi Liu Yi Tang in a Murine Model of Cyclophosphamide-In- duced Leucopenia,” Journal of Ethnopharmacology, Vol. 109, No. 2, 2007, pp. 229-235. doi:10.1016/j.jep.2006.07.023 [11] W. Wang, Q. L. Guo, Q. D. You, K. Zhang, Y. Yang, J. Yu, W. Liu, L. Zhao, H. Y. Gu, Y. Hu, Z. Tan and X. T. Wang, “The Anticancer Activities of Wogonin in Murine Sarcoma S180 Both in vitro and ex vivo,” Biological & Pharmaceutical Bulletin, Vol. 29, No. 6, 2006, pp. 1132- 1137. doi:10.1248/bpb.29.1132 [12] X. M. Wen, Y. L. Zhang, X. M. Liu, S. X. Guo and H. Wang, “Immune Responses in Mice to Arecoline Medi- ated by Lymphocyte Muscarinic Acetylcholine Recep- tor,” Cell Biology International, Vol. 30, 2006, pp. 1048- 1053. doi:10.1016/j.cellbi.2006.09.015 [13] K. M. Siu, D. H. Mak, P. Y. Chiu, M. K. Poon, Y. Du and K. M. Ko, “Pharmacological Basis of ‘Yin-Nourishing’ and ‘Yang-Invigorating’ Actions of Cordyceps, a Chinese Tonifying Herb,” Life Sciences, Vol. 76, No. 4, 2004, pp. 385-395. doi:10.1016/j.lfs.2004.07.014 [14] L. Quemeneur, M. C. Michallet, C. Ferraro-Peyret, P. Saint-Mezard, J. Benetiere, M. T. Ducluzeau, J. F. Nico- las and J. P. Revillard, “Immunosuppressive Antimetabo- lites Inhibit Induction of Contact Hypersensitivity While Lymphoablative Drugs Also Prevent Its Expression,” European Journal of Dermatology, Vol. 13, 2003, pp. 540-547. [15] K. Yamanouchi, H. Zhou, N. Roy-Chowdhury, F. Macaluso, L. Liu, T. Yamamoto, G. R. Yannam, C. Enke, T. D. Solberg, A. B. Adelson, J. L. Platt, I. J. Fox, J. Roy-Chowdhury and C. Guha, “Hepatic Irradiation Aug- Copyright © 2011 SciRes. CM
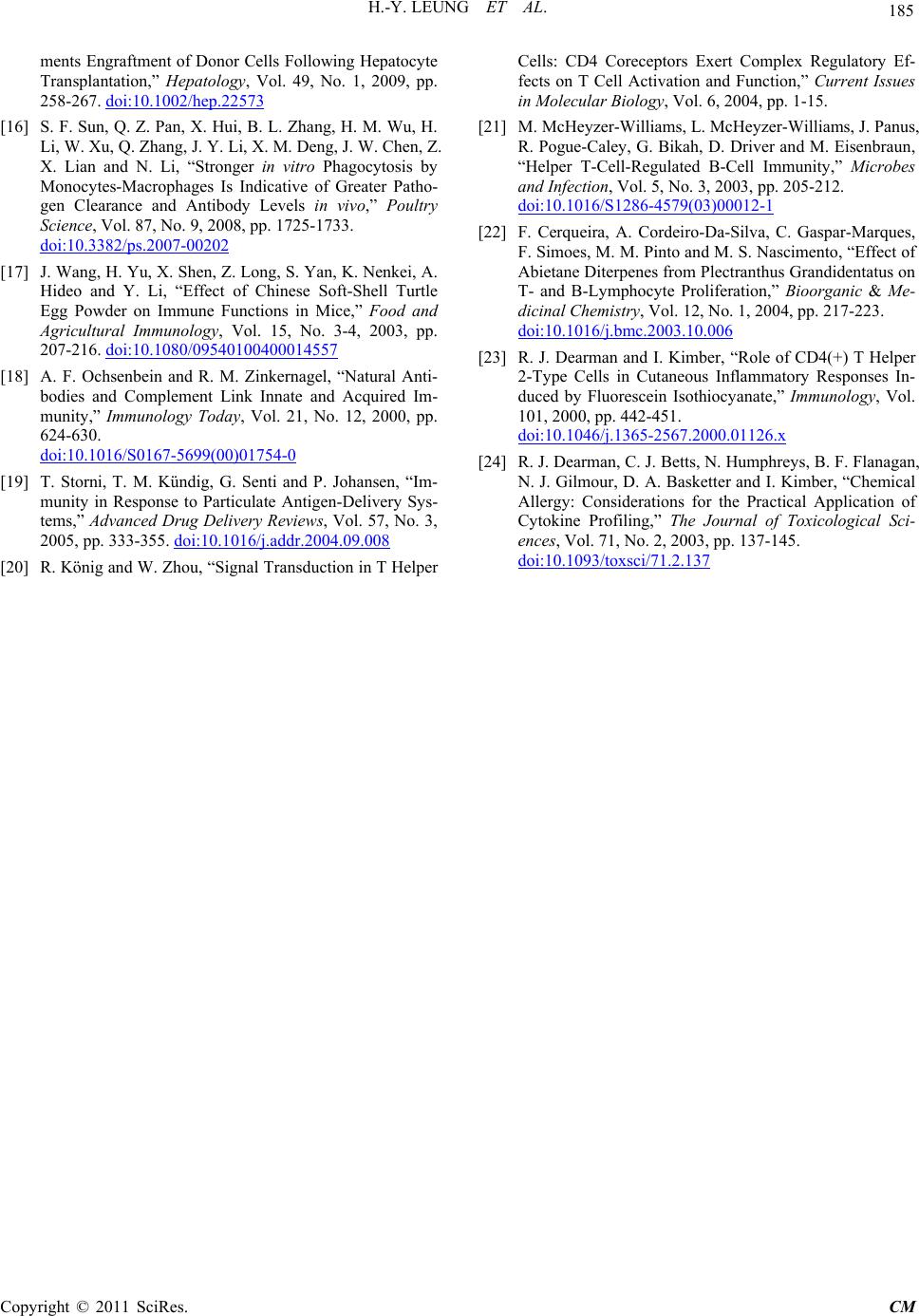 H.-Y. LEUNG ET AL. Copyright © 2011 SciRes. CM 185 ments Engraftment of Donor Cells Following Hepatocyte Transplantation,” Hepatology, Vol. 49, No. 1, 2009, pp. 258-267. doi:10.1002/hep.22573 [16] S. F. Sun, Q. Z. Pan, X. Hui, B. L. Zhang, H. M. Wu, H. Li, W. Xu, Q. Zhang, J. Y. Li, X. M. Deng, J. W. Chen, Z. X. Lian and N. Li, “Stronger in vitro Phagocytosis by Monocytes-Macrophages Is Indicative of Greater Patho- gen Clearance and Antibody Levels in vivo,” Poultry Science, Vol. 87, No. 9, 2008, pp. 1725-1733. doi:10.3382/ps.2007-00202 [17] J. Wang, H. Yu, X. Shen, Z. Long, S. Yan, K. Nenkei, A. Hideo and Y. Li, “Effect of Chinese Soft-Shell Turtle Egg Powder on Immune Functions in Mice,” Food and Agricultural Immunology, Vol. 15, No. 3-4, 2003, pp. 207-216. doi:10.1080/09540100400014557 [18] A. F. Ochsenbein and R. M. Zinkernagel, “Natural Anti- bodies and Complement Link Innate and Acquired Im- munity,” Immunology Today, Vol. 21, No. 12, 2000, pp. 624-630. doi:10.1016/S0167-5699(00)01754-0 [19] T. Storni, T. M. Kündig, G. Senti and P. Johansen, “Im- munity in Response to Particulate Antigen-Delivery Sys- tems,” Advanced Drug Delivery Reviews, Vol. 57, No. 3, 2005, pp. 333-355. doi:10.1016/j.addr.2004.09.008 [20] R. König and W. Zhou, “Signal Transduction in T Helper Cells: CD4 Coreceptors Exert Complex Regulatory Ef- fects on T Cell Activation and Function,” Current Issues in Molecular Biology, Vol. 6, 2004, pp. 1-15. [21] M. McHeyzer-Williams, L. McHeyzer-Williams, J. Panus, R. Pogue-Caley, G. Bikah, D. Driver and M. Eisenbraun, “Helper T-Cell-Regulated B-Cell Immunity,” Microbes and Infection, Vol. 5, No. 3, 2003, pp. 205-212. doi:10.1016/S1286-4579(03)00012-1 [22] F. Cerqueira, A. Cordeiro-Da-Silva, C. Gaspar-Marques, F. Simoes, M. M. Pinto and M. S. Nascimento, “Effect of Abietane Diterpenes from Plectranthus Grandidentatus on T- and B-Lymphocyte Proliferation,” Bioorganic & Me- dicinal Chemistry, Vol. 12, No. 1, 2004, pp. 217-223. doi:10.1016/j.bmc.2003.10.006 [23] R. J. Dearman and I. Kimber, “Role of CD4(+) T Helper 2-Type Cells in Cutaneous Inflammatory Responses In- duced by Fluorescein Isothiocyanate,” Immunology, Vol. 101, 2000, pp. 442-451. doi:10.1046/j.1365-2567.2000.01126.x [24] R. J. Dearman, C. J. Betts, N. Humphreys, B. F. Flanagan, N. J. Gilmour, D. A. Basketter and I. Kimber, “Chemical Allergy: Considerations for the Practical Application of Cytokine Profiling,” The Journal of Toxicological Sci- ences, Vol. 71, No. 2, 2003, pp. 137-145. doi:10.1093/toxsci/71.2.137
|