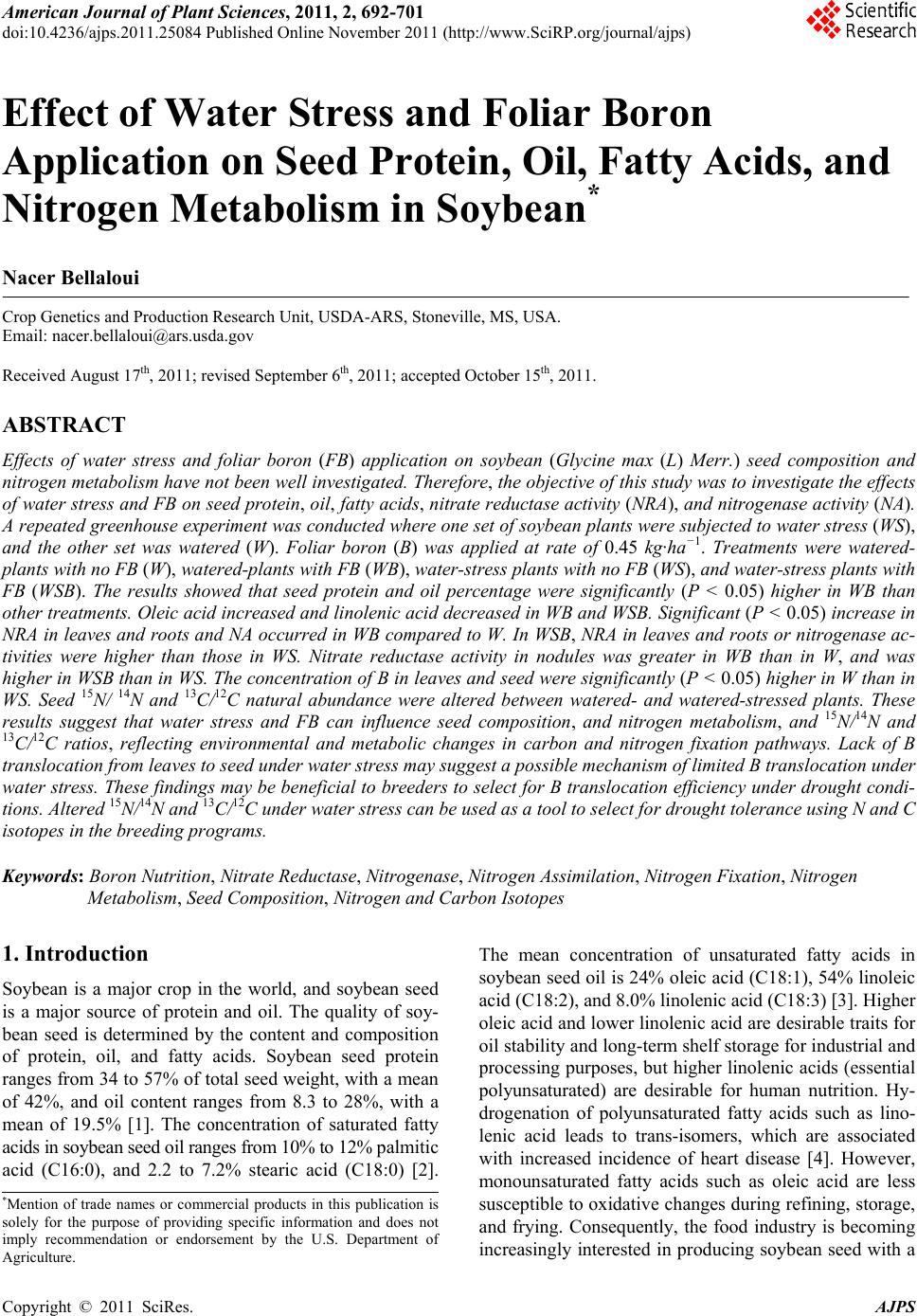 American Journal of Plant Sciences, 2011, 2, 692-701 doi:10.4236/ajps.2011.25084 Published Online November 2011 (http://www.SciRP.org/journal/ajps) Copyright © 2011 SciRes. AJPS Effect of Water Stress and Foliar Boron Application on Seed Protein, Oil, Fatty Acids, and Nitrogen Metabolism in Soybean* Nacer Bellaloui Crop Genetics and Production Research Unit, USDA-ARS, Stoneville, MS, USA. Email: nacer.bellaloui@ars.usda.gov Received August 17th, 2011; revised September 6th, 2011; accepted October 15th, 2011. ABSTRACT Effects of water stress and foliar boron (FB) application on soybean (Glycine max (L) Merr.) seed composition and nitrogen metabolism have not been well investigated. Therefore, the objective of this study was to investigate the effects of water stress and FB on seed protein, oil, fatty acids, nitrate reductase activity (NRA), and nitrogenase activity (NA). A repeated greenhouse experiment was conducted where one set of soybean plants were subjected to water stress (WS), and the other set was watered (W). Foliar boron (B) was applied at rate of 0.45 kg·ha−1. Treatments were watered- plants with no FB (W), watered-plants with FB (WB), water-stress plants with no FB (WS), and water-stress plants with FB (WSB). The results showed that seed protein and oil percentage were significantly (P < 0.05) higher in WB than other treatments. Oleic acid increased and linolenic acid decreased in WB and WSB. Significant (P < 0.05) increase in NRA in leaves and roots and NA occurred in WB compared to W. In WSB, NRA in leaves and roots or nitrogenase ac- tivities were higher than those in WS. Nitrate reductase activity in nodules was greater in WB than in W, and was higher in WSB than in WS. The concentration of B in leaves and seed were significantly (P < 0.05) higher in W than in WS. Seed 15N/ 14N and 13C/12C natural abundance were altered between watered- and watered-stressed plants. These results suggest that water stress and FB can influence seed composition, and nitrogen metabolism, and 15N/14N and 13C/12C ratios, reflecting environmental and metabolic changes in carbon and nitrogen fixation pathways. Lack of B translocation from leaves to seed under water stress may suggest a possible mechanism of limited B translocation under water stress. These findings may be beneficial to breeders to select for B translocation efficiency under drought condi- tions. Altered 15N/14N and 13C/12C under water stress can be used as a tool to select for drought tolerance using N and C isotopes in the breeding programs. Keywords: Boron Nutrition, Nitrate Reductase, Nitrogenase, Nitrogen Assimilation, Nitrogen Fixation, Nitrogen Metabolism, Seed Composition, Nitrogen and Carbon Isotopes 1. Introduction Soybean is a major crop in the world, and soybean seed is a major source of protein and oil. The quality of soy- bean seed is determined by the content and composition of protein, oil, and fatty acids. Soybean seed protein ranges from 34 to 57% of total seed weight, with a mean of 42%, and oil content ranges from 8.3 to 28%, with a mean of 19.5% [1]. The concentration of saturated fatty acids in soybean seed oil ranges from 10% to 12% palmitic acid (C16:0), and 2.2 to 7.2% stearic acid (C18:0) [2]. The mean concentration of unsaturated fatty acids in soybean seed oil is 24% oleic acid (C18:1), 54% linoleic acid (C18:2), and 8.0% linolenic acid (C18:3) [3]. Higher oleic acid and lower linolenic acid are desirable traits for oil stability and long-term shelf storage for industrial and processing purposes, but higher linolenic acids (essential polyunsaturated) are desirable for human nutrition. Hy- drogenation of polyunsaturated fatty acids such as lino- lenic acid leads to trans-isomers, which are associated with increased incidence of heart disease [4]. However, monounsaturated fatty acids such as oleic acid are less susceptible to oxidative changes during refining, storage, and frying. Consequently, the food industry is becoming increasingly interested in producing soybean seed with a *Mention of trade names or commercial products in this publication is solely for the purpose of providing specific information and does no imply recommendation or endorsement by the U.S. Department o A riculture.
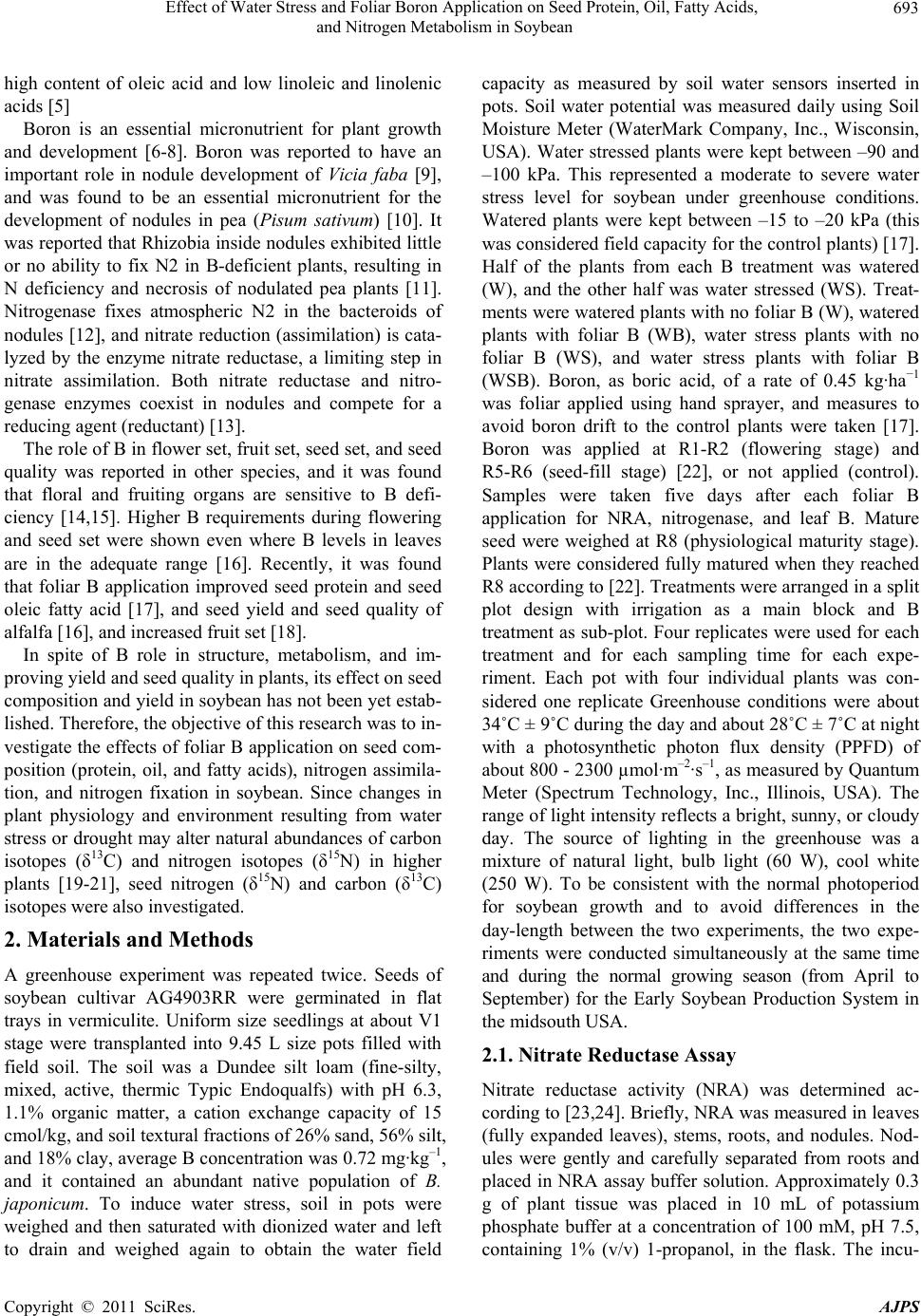 Effect of Water Stress and Foliar Boron Application on Seed Protein, Oil, Fatty Acids, 693 and Nitrogen Metabolism in Soybean high content of oleic acid and low linoleic and linolenic acids [5] Boron is an essential micronutrient for plant growth and development [6-8]. Boron was reported to have an important role in nodule development of Vicia faba [9], and was found to be an essential micronutrient for the development of nodules in pea (Pisum sativum) [10]. It was reported that Rhizobia inside nodules exhibited little or no ability to fix N2 in B-deficient plants, resulting in N deficiency and necrosis of nodulated pea plants [11]. Nitrogenase fixes atmospheric N2 in the bacteroids of nodules [12], and nitrate reduction (assimilation) is cata- lyzed by the enzyme nitrate reductase, a limiting step in nitrate assimilation. Both nitrate reductase and nitro- genase enzymes coexist in nodules and compete for a reducing agent (reductant) [13]. The role of B in flower set, fruit set, seed set, and seed quality was reported in other species, and it was found that floral and fruiting organs are sensitive to B defi- ciency [14,15]. Higher B requirements during flowering and seed set were shown even where B levels in leaves are in the adequate range [16]. Recently, it was found that foliar B application improved seed protein and seed oleic fatty acid [17], and seed yield and seed quality of alfalfa [16], and increased fruit set [18]. In spite of B role in structure, metabolism, and im- proving yield and seed quality in plants, its effect on seed composition and yield in soybean has not been yet estab- lished. Therefore, the objective of this research was to in- vestigate the effects of foliar B application on seed com- position (protein, oil, and fatty acids), nitrogen assimila- tion, and nitrogen fixation in soybean. Since changes in plant physiology and environment resulting from water stress or drought may alter natural abundances of carbon isotopes (δ13C) and nitrogen isotopes (δ15N) in higher plants [19-21], seed nitrogen (δ15N) and carbon (δ13C) isotopes were also investigated. 2. Materials and Methods A greenhouse experiment was repeated twice. Seeds of soybean cultivar AG4903RR were germinated in flat trays in vermiculite. Uniform size seedlings at about V1 stage were transplanted into 9.45 L size pots filled with field soil. The soil was a Dundee silt loam (fine-silty, mixed, active, thermic Typic Endoqualfs) with pH 6.3, 1.1% organic matter, a cation exchange capacity of 15 cmol/kg, and soil textural fractions of 26% sand, 56% silt, and 18% clay, average B concentration was 0.72 mg·kg–1, and it contained an abundant native population of B. japonicum. To induce water stress, soil in pots were weighed and then saturated with dionized water and left to drain and weighed again to obtain the water field capacity as measured by soil water sensors inserted in pots. Soil water potential was measured daily using Soil Moisture Meter (WaterMark Company, Inc., Wisconsin, USA). Water stressed plants were kept between –90 and –100 kPa. This represented a moderate to severe water stress level for soybean under greenhouse conditions. Watered plants were kept between –15 to –20 kPa (this was considered field capacity for the control plants) [17]. Half of the plants from each B treatment was watered (W), and the other half was water stressed (WS). Treat- ments were watered plants with no foliar B (W), watered plants with foliar B (WB), water stress plants with no foliar B (WS), and water stress plants with foliar B (WSB). Boron, as boric acid, of a rate of 0.45 kg·ha−1 was foliar applied using hand sprayer, and measures to avoid boron drift to the control plants were taken [17]. Boron was applied at R1-R2 (flowering stage) and R5-R6 (seed-fill stage) [22], or not applied (control). Samples were taken five days after each foliar B application for NRA, nitrogenase, and leaf B. Mature seed were weighed at R8 (physiological maturity stage). Plants were considered fully matured when they reached R8 according to [22]. Treatments were arranged in a split plot design with irrigation as a main block and B treatment as sub-plot. Four replicates were used for each treatment and for each sampling time for each expe- riment. Each pot with four individual plants was con- sidered one replicate Greenhouse conditions were about 34˚C ± 9˚C during the day and about 28˚C ± 7˚C at night with a photosynthetic photon flux density (PPFD) of about 800 - 2300 µmol·m–2·s–1, as measured by Quantum Meter (Spectrum Technology, Inc., Illinois, USA). The range of light intensity reflects a bright, sunny, or cloudy day. The source of lighting in the greenhouse was a mixture of natural light, bulb light (60 W), cool white (250 W). To be consistent with the normal photoperiod for soybean growth and to avoid differences in the day-length between the two experiments, the two expe- riments were conducted simultaneously at the same time and during the normal growing season (from April to September) for the Early Soybean Production System in the midsouth USA. 2.1. Nitrate Reductase Assay Nitrate reductase activity (NRA) was determined ac- cording to [23,24]. Briefly, NRA was measured in leaves (fully expanded leaves), stems, roots, and nodules. Nod- ules were gently and carefully separated from roots and placed in NRA assay buffer solution. Approximately 0.3 g of plant tissue was placed in 10 mL of potassium phosphate buffer at a concentration of 100 mM, pH 7.5, containing 1% (v/v) 1-propanol, in the flask. The incu- Copyright © 2011 SciRes. AJPS
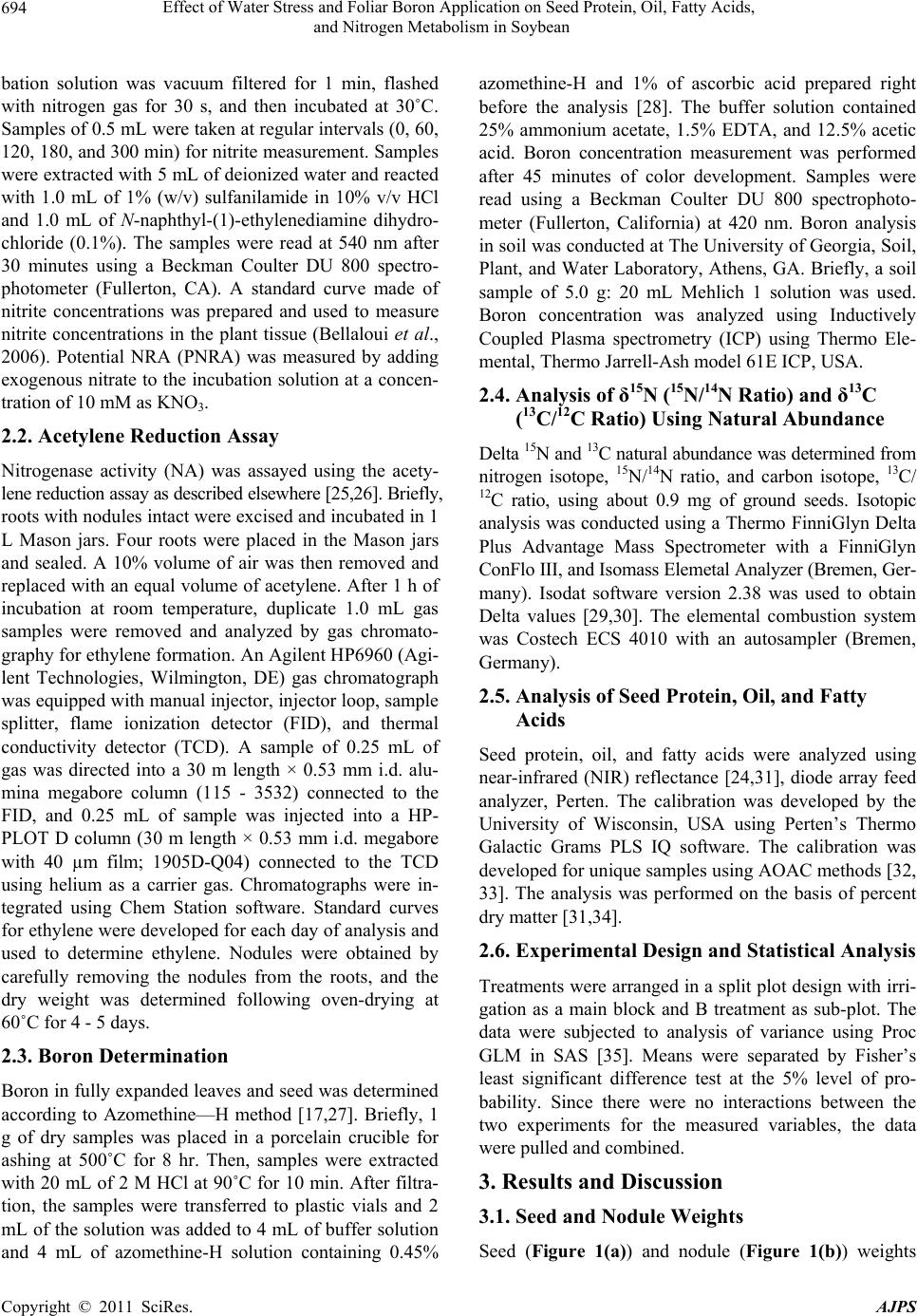 Effect of Water Stress and Foliar Boron Application on Seed Protein, Oil, Fatty Acids, 694 and Nitrogen Metabolism in Soybean bation solution was vacuum filtered for 1 min, flashed with nitrogen gas for 30 s, and then incubated at 30˚C. Samples of 0.5 mL were taken at regular intervals (0, 60, 120, 180, and 300 min) for nitrite measurement. Samples were extracted with 5 mL of deionized water and reacted with 1.0 mL of 1% (w/v) sulfanilamide in 10% v/v HCl and 1.0 mL of N-naphthyl-(1)-ethylenediamine dihydro- chloride (0.1%). The samples were read at 540 nm after 30 minutes using a Beckman Coulter DU 800 spectro- photometer (Fullerton, CA). A standard curve made of nitrite concentrations was prepared and used to measure nitrite concentrations in the plant tissue (Bellaloui et al., 2006). Potential NRA (PNRA) was measured by adding exogenous nitrate to the incubation solution at a concen- tration of 10 mM as KNO3. 2.2. Acetylene Reduction Assay Nitrogenase activity (NA) was assayed using the acety- lene reduction assay as described elsewhere [25,26]. Briefly, roots with nodules intact were excised and incubated in 1 L Mason jars. Four roots were placed in the Mason jars and sealed. A 10% volume of air was then removed and replaced with an equal volume of acetylene. After 1 h of incubation at room temperature, duplicate 1.0 mL gas samples were removed and analyzed by gas chromato- graphy for ethylene formation. An Agilent HP6960 (Agi- lent Technologies, Wilmington, DE) gas chromatograph was equipped with manual injector, injector loop, sample splitter, flame ionization detector (FID), and thermal conductivity detector (TCD). A sample of 0.25 mL of gas was directed into a 30 m length × 0.53 mm i.d. alu- mina megabore column (115 - 3532) connected to the FID, and 0.25 mL of sample was injected into a HP- PLOT D column (30 m length × 0.53 mm i.d. megabore with 40 µm film; 1905D-Q04) connected to the TCD using helium as a carrier gas. Chromatographs were in- tegrated using Chem Station software. Standard curves for ethylene were developed for each day of analysis and used to determine ethylene. Nodules were obtained by carefully removing the nodules from the roots, and the dry weight was determined following oven-drying at 60˚C for 4 - 5 days. 2.3. Boron Determination Boron in fully expanded leaves and seed was determined according to Azomethine—H method [17,27]. Briefly, 1 g of dry samples was placed in a porcelain crucible for ashing at 500˚C for 8 hr. Then, samples were extracted with 20 mL of 2 M HCl at 90˚C for 10 min. After filtra- tion, the samples were transferred to plastic vials and 2 mL of the solution was added to 4 mL of buffer solution and 4 mL of azomethine-H solution containing 0.45% azomethine-H and 1% of ascorbic acid prepared right before the analysis [28]. The buffer solution contained 25% ammonium acetate, 1.5% EDTA, and 12.5% acetic acid. Boron concentration measurement was performed after 45 minutes of color development. Samples were read using a Beckman Coulter DU 800 spectrophoto- meter (Fullerton, California) at 420 nm. Boron analysis in soil was conducted at The University of Georgia, Soil, Plant, and Water Laboratory, Athens, GA. Briefly, a soil sample of 5.0 g: 20 mL Mehlich 1 solution was used. Boron concentration was analyzed using Inductively Coupled Plasma spectrometry (ICP) using Thermo Ele- mental, Thermo Jarrell-Ash model 61E ICP, USA. 2.4. Analysis of δ15N (15N/14N Ratio) and δ13C (13C/12C Ratio) Using Natural Abundance Delta 15N and 13C natural abundance was determined from nitrogen isotope, 15N/14N ratio, and carbon isotope, 13C/ 12C ratio, using about 0.9 mg of ground seeds. Isotopic analysis was conducted using a Thermo FinniGlyn Delta Plus Advantage Mass Spectrometer with a FinniGlyn ConFlo III, and Isomass Elemetal Analyzer (Bremen, Ger- many). Isodat software version 2.38 was used to obtain Delta values [29,30]. The elemental combustion system was Costech ECS 4010 with an autosampler (Bremen, Germany). 2.5. Analysis of Seed Protein, Oil, and Fatty Acids Seed protein, oil, and fatty acids were analyzed using near-infrared (NIR) reflectance [24,31], diode array feed analyzer, Perten. The calibration was developed by the University of Wisconsin, USA using Perten’s Thermo Galactic Grams PLS IQ software. The calibration was developed for unique samples using AOAC methods [32, 33]. The analysis was performed on the basis of percent dry matter [31,34]. 2.6. Experimental Design and Statistical Analysis Treatments were arranged in a split plot design with irri- gation as a main block and B treatment as sub-plot. The data were subjected to analysis of variance using Proc GLM in SAS [35]. Means were separated by Fisher’s least significant difference test at the 5% level of pro- bability. Since there were no interactions between the two experiments for the measured variables, the data were pulled and combined. 3. Results and Discussion 3.1. Seed and Nodule Weights Seed (Figure 1(a)) and nodule (Figure 1(b)) weights Copyright © 2011 SciRes. AJPS
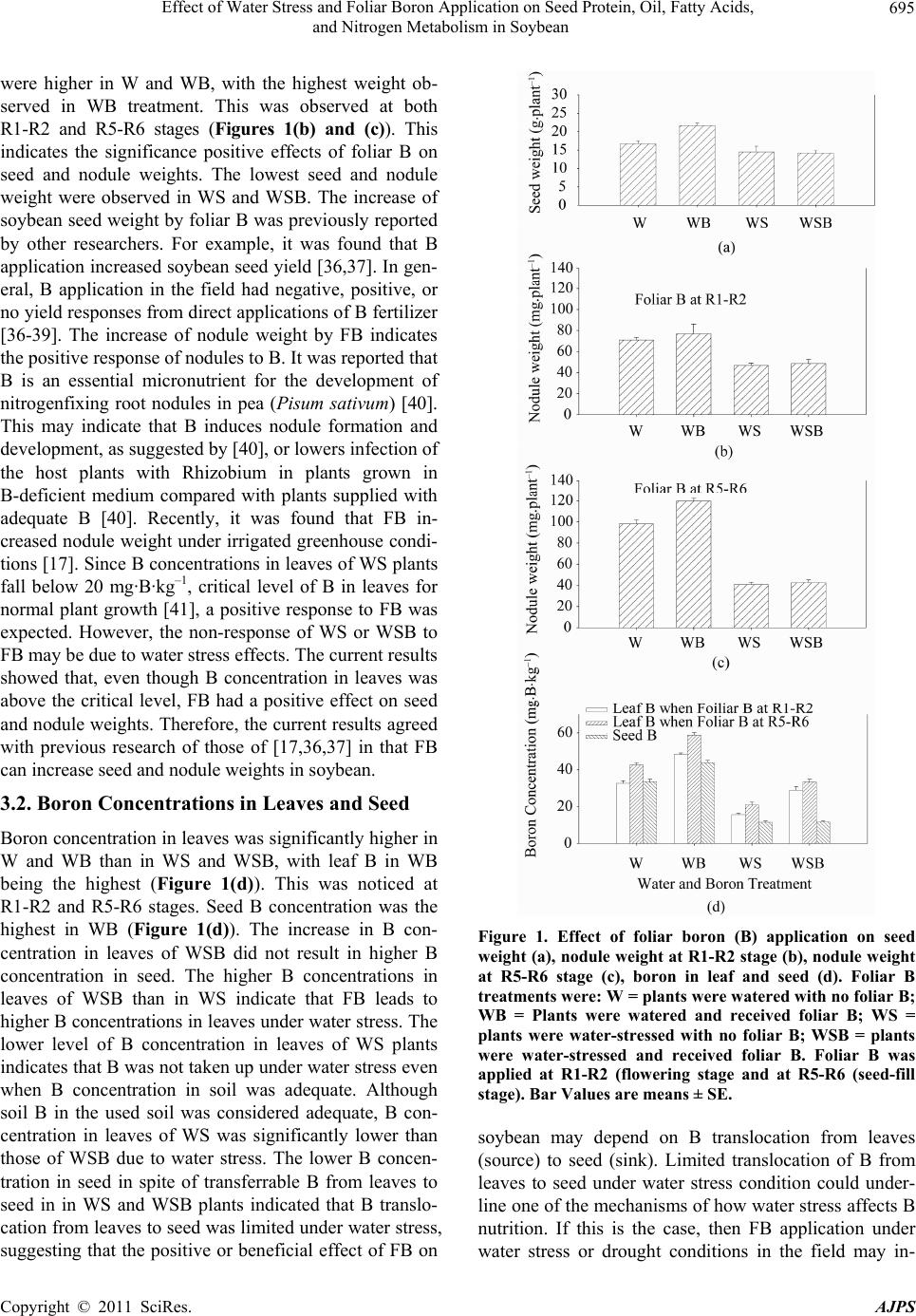 Effect of Water Stress and Foliar Boron Application on Seed Protein, Oil, Fatty Acids, 695 and Nitrogen Metabolism in Soybean were higher in W and WB, with the highest weight ob- served in WB treatment. This was observed at both R1-R2 and R5-R6 stages (Figures 1(b) and (c)). This indicates the significance positive effects of foliar B on seed and nodule weights. The lowest seed and nodule weight were observed in WS and WSB. The increase of soybean seed weight by foliar B was previously reported by other researchers. For example, it was found that B application increased soybean seed yield [36,37]. In gen- eral, B application in the field had negative, positive, or no yield responses from direct applications of B fertilizer [36-39]. The increase of nodule weight by FB indicates the positive response of nodules to B. It was reported that B is an essential micronutrient for the development of nitrogenfixing root nodules in pea (Pisum sativum) [40]. This may indicate that B induces nodule formation and development, as suggested by [40], or lowers infection of the host plants with Rhizobium in plants grown in B-deficient medium compared with plants supplied with adequate B [40]. Recently, it was found that FB in- creased nodule weight under irrigated greenhouse condi- tions [17]. Since B concentrations in leaves of WS plants fall below 20 mg·B·kg–1, critical level of B in leaves for normal plant growth [41], a positive response to FB was expected. However, the non-response of WS or WSB to FB may be due to water stress effects. The current results showed that, even though B concentration in leaves was above the critical level, FB had a positive effect on seed and nodule weights. Therefore, the current results agreed with previous research of those of [17,36,37] in that FB can increase seed and nodule weights in soybean. 3.2. Boron Concentrations in Leaves and Seed Boron concentration in leaves was significantly higher in W and WB than in WS and WSB, with leaf B in WB being the highest (Figure 1(d)). This was noticed at R1-R2 and R5-R6 stages. Seed B concentration was the highest in WB (Figure 1(d)). The increase in B con- centration in leaves of WSB did not result in higher B concentration in seed. The higher B concentrations in leaves of WSB than in WS indicate that FB leads to higher B concentrations in leaves under water stress. The lower level of B concentration in leaves of WS plants indicates that B was not taken up under water stress even when B concentration in soil was adequate. Although soil B in the used soil was considered adequate, B con- centration in leaves of WS was significantly lower than those of WSB due to water stress. The lower B concen- tration in seed in spite of transferrable B from leaves to seed in in WS and WSB plants indicated that B translo- cation from leaves to seed was limited under water stress, suggesting that the positive or beneficial effect of FB on Figure 1. Effect of foliar boron (B) application on seed weight (a), nodule weight at R1-R2 stage (b), nodule weight at R5-R6 stage (c), boron in leaf and seed (d). Foliar B treatments were: W = plants were watered with no foliar B; WB = Plants were watered and received foliar B; WS = plants were water-stressed with no foliar B; WSB = plants were water-stressed and received foliar B. Foliar B was applied at R1-R2 (flowering stage and at R5-R6 (seed-fill stage). Bar Values are means ± SE. soybean may depend on B translocation from leaves (source) to seed (sink). Limited translocation of B from leaves to seed under water stress condition could under- line one of the mechanisms of how water stress affects B nutrition. If this is the case, then FB application under water stress or drought conditions in the field may in- Copyright © 2011 SciRes. AJPS
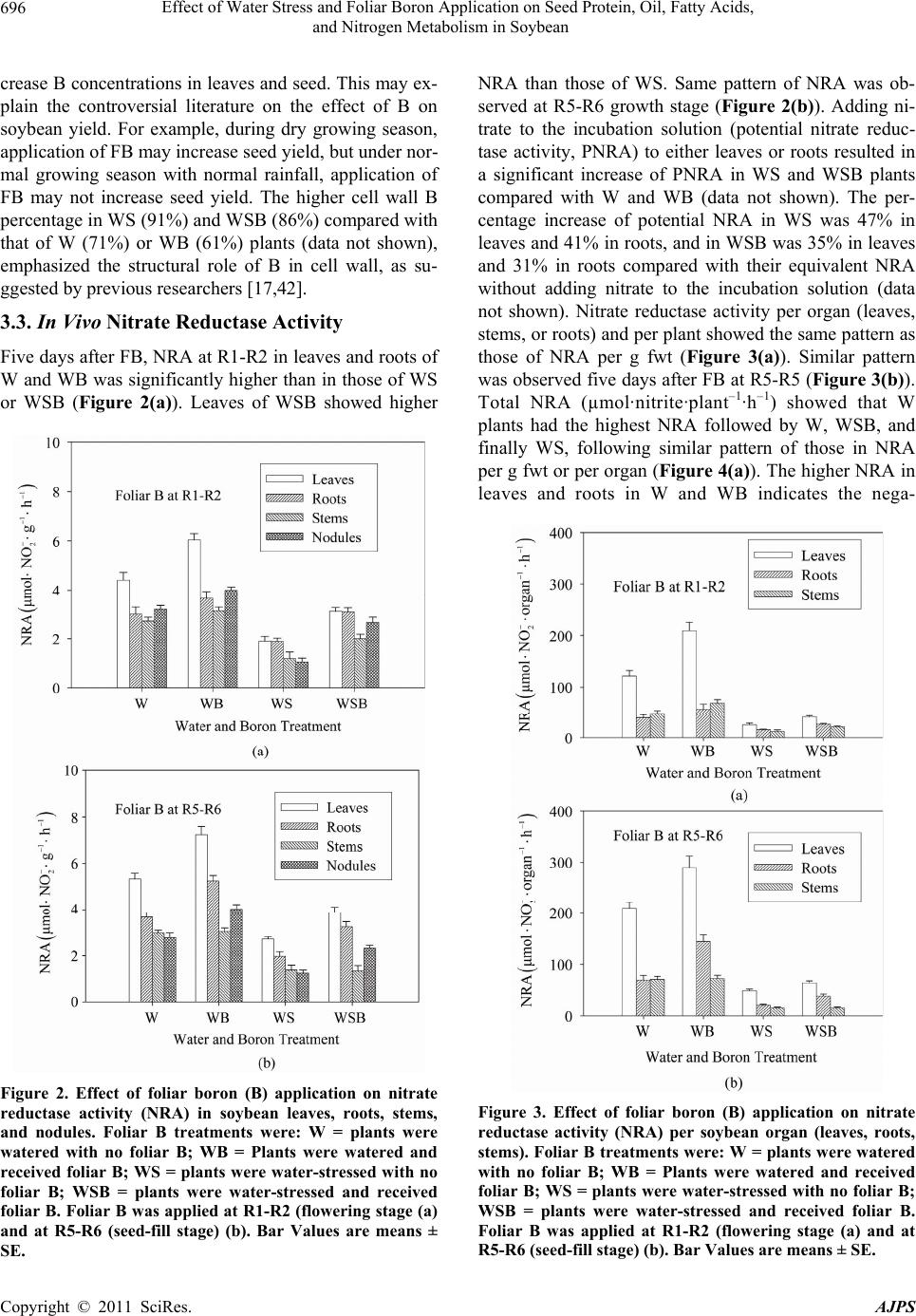 Effect of Water Stress and Foliar Boron Application on Seed Protein, Oil, Fatty Acids, 696 and Nitrogen Metabolism in Soybean crease B concentrations in leaves and seed. This may ex- plain the controversial literature on the effect of B on soybean yield. For example, during dry growing season, application of FB may increase seed yield, but under nor- mal growing season with normal rainfall, application of FB may not increase seed yield. The higher cell wall B percentage in WS (91%) and WSB (86%) compared with that of W (71%) or WB (61%) plants (data not shown), emphasized the structural role of B in cell wall, as su- ggested by previous researchers [17,42]. 3.3. In Vivo Nitrate Reductase Activity Five days after FB, NRA at R1-R2 in leaves and roots of W and WB was significantly higher than in those of WS or WSB (Figure 2(a)). Leaves of WSB showed higher Figure 2. Effect of foliar boron (B) application on nitrate reductase activity (NRA) in soybean leaves, roots, stems, and nodules. Foliar B treatments were: W = plants were watered with no foliar B; WB = Plants were watered and received foliar B; WS = plants were water-stressed with no foliar B; WSB = plants were water-stressed and received foliar B. Foliar B was applied at R1-R2 (flowering stage (a) and at R5-R6 (seed-fill stage) (b). Bar Values are means ± SE. NRA than those of WS. Same pattern of NRA was ob- served at R5-R6 growth stage (Figure 2(b)). Adding ni- trate to the incubation solution (potential nitrate reduc- tase activity, PNRA) to either leaves or roots resulted in a significant increase of PNRA in WS and WSB plants compared with W and WB (data not shown). The per- centage increase of potential NRA in WS was 47% in leaves and 41% in roots, and in WSB was 35% in leaves and 31% in roots compared with their equivalent NRA without adding nitrate to the incubation solution (data not shown). Nitrate reductase activity per organ (leaves, stems, or roots) and per plant showed the same pattern as those of NRA per g fwt (Figure 3(a)). Similar pattern was observed five days after FB at R5-R5 (Figure 3(b)). Total NRA (µmol·nitrite·plant–1·h–1) showed that W plants had the highest NRA followed by W, WSB, and finally WS, following similar pattern of those in NRA per g fwt or per organ (Figure 4(a)). The higher NRA in leaves and roots in W and WB indicates the nega- Figure 3. Effect of foliar boron (B) application on nitrate reductase activity (NRA) per soybean organ (leaves, roots, stems). Foliar B treatments were: W = plants were watered with no foliar B; WB = Plants were watered and received foliar B; WS = plants were water-stressed with no foliar B; WSB = plants were water-stressed and received foliar B. Foliar B was applied at R1-R2 (flowering stage (a) and at R5-R6 (seed-fill stage) (b). Bar Values are means ± SE. Copyright © 2011 SciRes. AJPS
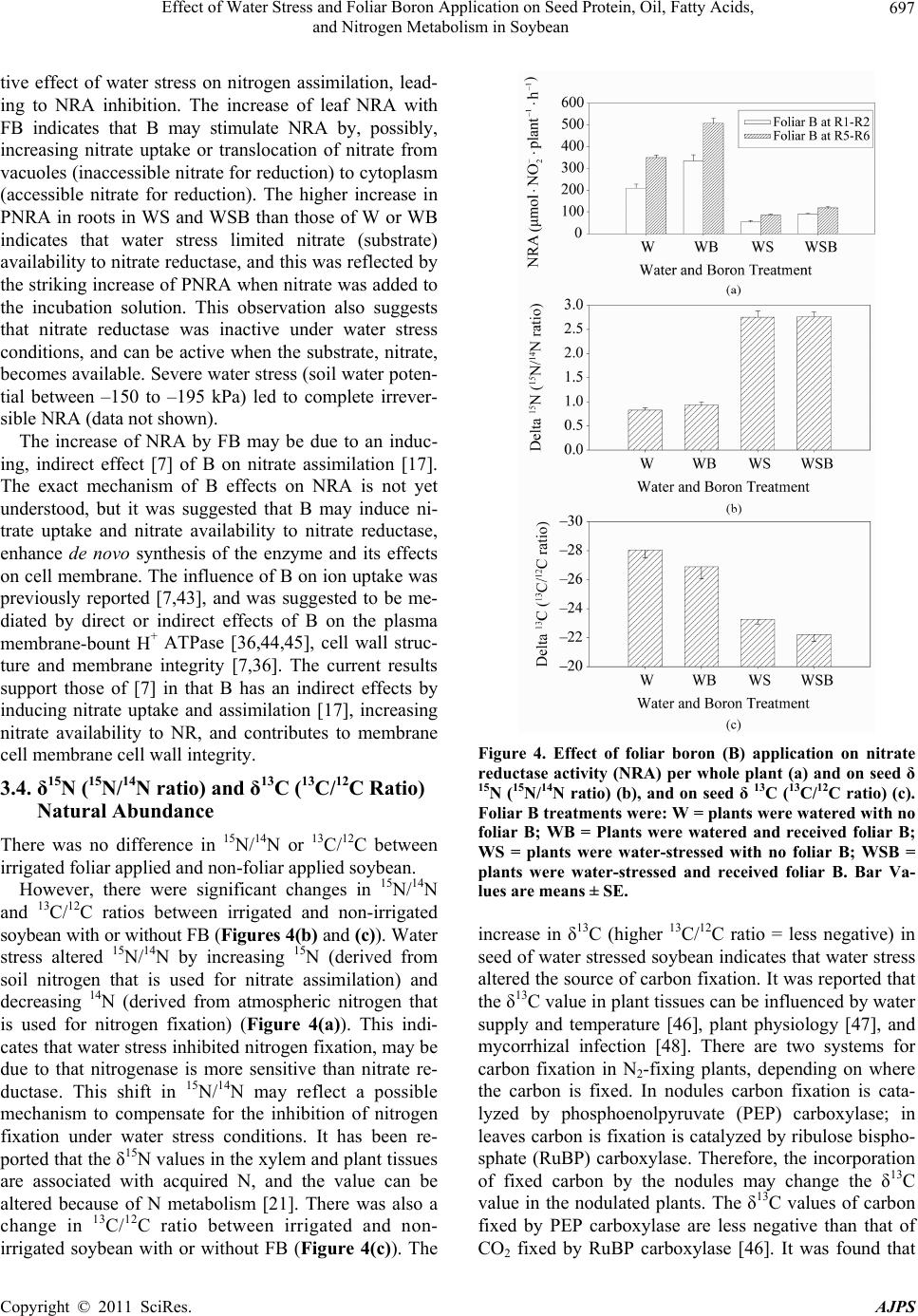 Effect of Water Stress and Foliar Boron Application on Seed Protein, Oil, Fatty Acids, 697 and Nitrogen Metabolism in Soybean tive effect of water stress on nitrogen assimilation, lead- ing to NRA inhibition. The increase of leaf NRA with FB indicates that B may stimulate NRA by, possibly, increasing nitrate uptake or translocation of nitrate from vacuoles (inaccessible nitrate for reduction) to cytoplasm (accessible nitrate for reduction). The higher increase in PNRA in roots in WS and WSB than those of W or WB indicates that water stress limited nitrate (substrate) availability to nitrate reductase, and this was reflected by the striking increase of PNRA when nitrate was added to the incubation solution. This observation also suggests that nitrate reductase was inactive under water stress conditions, and can be active when the substrate, nitrate, becomes available. Severe water stress (soil water poten- tial between –150 to –195 kPa) led to complete irrever- sible NRA (data not shown). The increase of NRA by FB may be due to an induc- ing, indirect effect [7] of B on nitrate assimilation [17]. The exact mechanism of B effects on NRA is not yet understood, but it was suggested that B may induce ni- trate uptake and nitrate availability to nitrate reductase, enhance de novo synthesis of the enzyme and its effects on cell membrane. The influence of B on ion uptake was previously reported [7,43], and was suggested to be me- diated by direct or indirect effects of B on the plasma membrane-bount H+ ATPase [36,44,45], cell wall struc- ture and membrane integrity [7,36]. The current results support those of [7] in that B has an indirect effects by inducing nitrate uptake and assimilation [17], increasing nitrate availability to NR, and contributes to membrane cell membrane cell wall integrity. 3.4. δ15N (15N/14N ratio) and δ13C (13C/12C Ratio) Natural Abundance There was no difference in 15N/14N or 13C/12C between irrigated foliar applied and non-foliar applied soybean. However, there were significant changes in 15N/14N and 13C/12C ratios between irrigated and non-irrigated soybean with or without FB (Figures 4(b) and (c)). Water stress altered 15N/14N by increasing 15N (derived from soil nitrogen that is used for nitrate assimilation) and decreasing 14N (derived from atmospheric nitrogen that is used for nitrogen fixation) (Figure 4(a)). This indi- cates that water stress inhibited nitrogen fixation, may be due to that nitrogenase is more sensitive than nitrate re- ductase. This shift in 15N/14N may reflect a possible mechanism to compensate for the inhibition of nitrogen fixation under water stress conditions. It has been re- ported that the δ15N values in the xylem and plant tissues are associated with acquired N, and the value can be altered because of N metabolism [21]. There was also a change in 13C/12C ratio between irrigated and non- irrigated soybean with or without FB (Figure 4(c)). The Figure 4. Effect of foliar boron (B) application on nitrate reductase activity (NRA) per whole plant (a) and on seed δ 15N (15N/14N ratio) (b), and on seed δ 13C (13C/12C ratio) (c). Foliar B treatments were: W = plants were watered with no foliar B; WB = Plants were watered and received foliar B; WS = plants were water-stressed with no foliar B; WSB = plants were water-stressed and received foliar B. Bar Va- lues are means ± SE. increase in δ13C (higher 13C/12C ratio = less negative) in seed of water stressed soybean indicates that water stress altered the source of carbon fixation. It was reported that the δ13C value in plant tissues can be influenced by water supply and temperature [46], plant physiology [47], and mycorrhizal infection [48]. There are two systems for carbon fixation in N2-fixing plants, depending on where the carbon is fixed. In nodules carbon fixation is cata- lyzed by phosphoenolpyruvate (PEP) carboxylase; in leaves carbon is fixation is catalyzed by ribulose bispho- sphate (RuBP) carboxylase. Therefore, the incorporation of fixed carbon by the nodules may change the δ13C value in the nodulated plants. The δ13C values of carbon fixed by PEP carboxylase are less negative than that of CO2 fixed by RuBP carboxylase [46]. It was found that Copyright © 2011 SciRes. AJPS
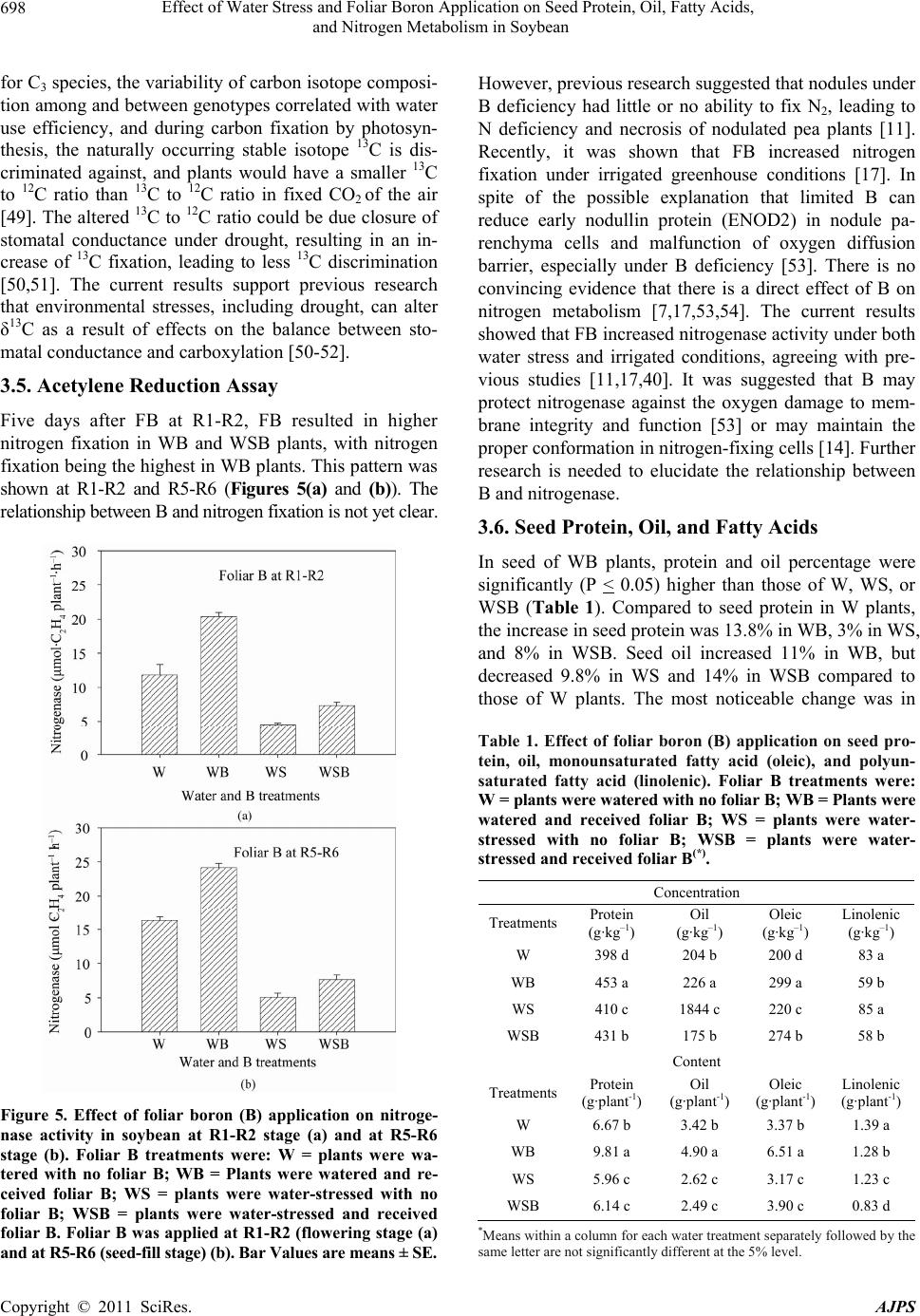 Effect of Water Stress and Foliar Boron Application on Seed Protein, Oil, Fatty Acids, 698 and Nitrogen Metabolism in Soybean for C3 species, the variability of carbon isotope composi- tion among and between genotypes correlated with water use efficiency, and during carbon fixation by photosyn- thesis, the naturally occurring stable isotope 13C is dis- criminated against, and plants would have a smaller 13C to 12C ratio than 13C to 12C ratio in fixed CO2 of the air [49]. The altered 13C to 12C ratio could be due closure of stomatal conductance under drought, resulting in an in- crease of 13C fixation, leading to less 13C discrimination [50,51]. The current results support previous research that environmental stresses, including drought, can alter δ13C as a result of effects on the balance between sto- matal conductance and carboxylation [50-52]. 3.5. Acetylene Reduction Assay Five days after FB at R1-R2, FB resulted in higher nitrogen fixation in WB and WSB plants, with nitrogen fixation being the highest in WB plants. This pattern was shown at R1-R2 and R5-R6 (Figures 5(a) and (b)). The relationship between B and nitrogen fixation is not yet clear. Figure 5. Effect of foliar boron (B) application on nitroge- nase activity in soybean at R1-R2 stage (a) and at R5-R6 stage (b). Foliar B treatments were: W = plants were wa- tered with no foliar B; WB = Plants were watered and re- ceived foliar B; WS = plants were water-stressed with no foliar B; WSB = plants were water-stressed and received foliar B. Foliar B was applied at R1-R2 (flowering stage (a) and at R5-R6 (seed-fill stage) (b). Bar Values are means ± SE. However, previous research suggested that nodules under B deficiency had little or no ability to fix N2, leading to N deficiency and necrosis of nodulated pea plants [11]. Recently, it was shown that FB increased nitrogen fixation under irrigated greenhouse conditions [17]. In spite of the possible explanation that limited B can reduce early nodullin protein (ENOD2) in nodule pa- renchyma cells and malfunction of oxygen diffusion barrier, especially under B deficiency [53]. There is no convincing evidence that there is a direct effect of B on nitrogen metabolism [7,17,53,54]. The current results showed that FB increased nitrogenase activity under both water stress and irrigated conditions, agreeing with pre- vious studies [11,17,40]. It was suggested that B may protect nitrogenase against the oxygen damage to mem- brane integrity and function [53] or may maintain the proper conformation in nitrogen-fixing cells [14]. Further research is needed to elucidate the relationship between B and nitrogenase. 3.6. Seed Protein, Oil, and Fatty Acids In seed of WB plants, protein and oil percentage were significantly (P < 0.05) higher than those of W, WS, or WSB (Table 1). Compared to seed protein in W plants, the increase in seed protein was 13.8% in WB, 3% in WS, and 8% in WSB. Seed oil increased 11% in WB, but decreased 9.8% in WS and 14% in WSB compared to those of W plants. The most noticeable change was in Table 1. Effect of foliar boron (B) application on seed pro- tein, oil, monounsaturated fatty acid (oleic), and polyun- saturated fatty acid (linolenic). Foliar B treatments were: W = plants were watered with no foliar B; WB = Plants were watered and received foliar B; WS = plants were water- stressed with no foliar B; WSB = plants were water- stressed and received foliar B(*). Concentration Treatments Protein (g·kg–1) Oil (g·kg–1) Oleic (g·kg–1) Linolenic (g·kg–1) W 398 d 204 b 200 d 83 a WB 453 a 226 a 299 a 59 b WS 410 c 1844 c 220 c 85 a WSB 431 b 175 b 274 b 58 b Content Treatments Protein (g·plant-1) Oil (g·plant-1) Oleic (g·plant-1) Linolenic (g·plant-1) W 6.67 b 3.42 b 3.37 b 1.39 a WB 9.81 a 4.90 a 6.51 a 1.28 b WS 5.96 c 2.62 c 3.17 c 1.23 c WSB 6.14 c 2.49 c 3.90 c 0.83 d *Means within a column for each water treatment separately followed by the same letter are not significantly different at the 5% level. Copyright © 2011 SciRes. AJPS
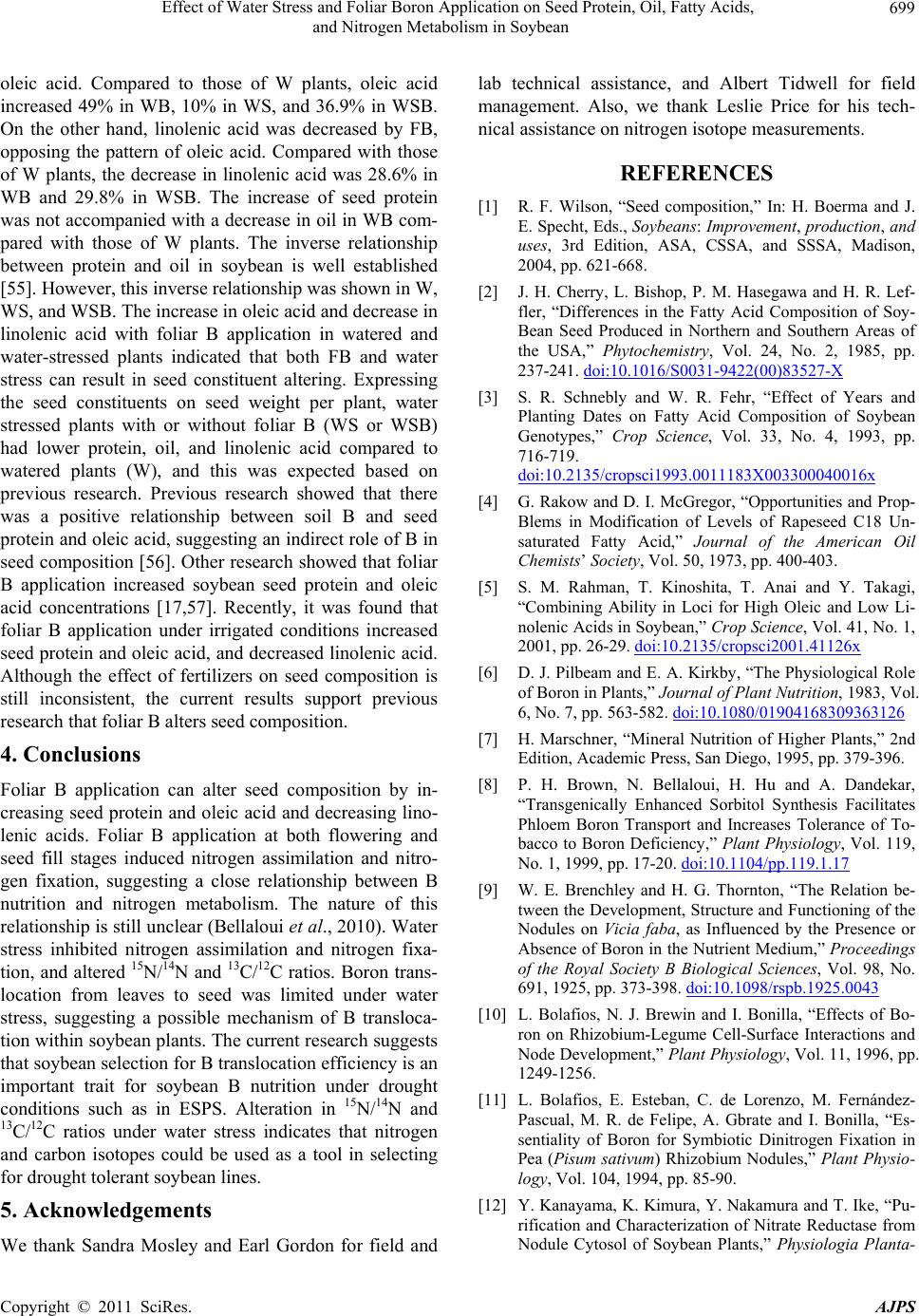 Effect of Water Stress and Foliar Boron Application on Seed Protein, Oil, Fatty Acids, 699 and Nitrogen Metabolism in Soybean oleic acid. Compared to those of W plants, oleic acid increased 49% in WB, 10% in WS, and 36.9% in WSB. On the other hand, linolenic acid was decreased by FB, opposing the pattern of oleic acid. Compared with those of W plants, the decrease in linolenic acid was 28.6% in WB and 29.8% in WSB. The increase of seed protein was not accompanied with a decrease in oil in WB com- pared with those of W plants. The inverse relationship between protein and oil in soybean is well established [55]. However, this inverse relationship was shown in W, WS, and WSB. The increase in oleic acid and decrease in linolenic acid with foliar B application in watered and water-stressed plants indicated that both FB and water stress can result in seed constituent altering. Expressing the seed constituents on seed weight per plant, water stressed plants with or without foliar B (WS or WSB) had lower protein, oil, and linolenic acid compared to watered plants (W), and this was expected based on previous research. Previous research showed that there was a positive relationship between soil B and seed protein and oleic acid, suggesting an indirect role of B in seed composition [56]. Other research showed that foliar B application increased soybean seed protein and oleic acid concentrations [17,57]. Recently, it was found that foliar B application under irrigated conditions increased seed protein and oleic acid, and decreased linolenic acid. Although the effect of fertilizers on seed composition is still inconsistent, the current results support previous research that foliar B alters seed composition. 4. Conclusions Foliar B application can alter seed composition by in- creasing seed protein and oleic acid and decreasing lino- lenic acids. Foliar B application at both flowering and seed fill stages induced nitrogen assimilation and nitro- gen fixation, suggesting a close relationship between B nutrition and nitrogen metabolism. The nature of this relationship is still unclear (Bellaloui et al., 2010). Water stress inhibited nitrogen assimilation and nitrogen fixa- tion, and altered 15N/14N and 13C/12C ratios. Boron trans- location from leaves to seed was limited under water stress, suggesting a possible mechanism of B transloca- tion within soybean plants. The current research suggests that soybean selection for B translocation efficiency is an important trait for soybean B nutrition under drought conditions such as in ESPS. Alteration in 15N/14N and 13C/12C ratios under water stress indicates that nitrogen and carbon isotopes could be used as a tool in selecting for drought tolerant soybean lines. 5. Acknowledgements We thank Sandra Mosley and Earl Gordon for field and lab technical assistance, and Albert Tidwell for field management. Also, we thank Leslie Price for his tech- nical assistance on nitrogen isotope measurements. REFERENCES [1] R. F. Wilson, “Seed composition,” In: H. Boerma and J. E. Specht, Eds., Soybeans: Improvement, production, and uses, 3rd Edition, ASA, CSSA, and SSSA, Madison, 2004, pp. 621-668. [2] J. H. Cherry, L. Bishop, P. M. Hasegawa and H. R. Lef- fler, “Differences in the Fatty Acid Composition of Soy- Bean Seed Produced in Northern and Southern Areas of the USA,” Phytochemistry, Vol. 24, No. 2, 1985, pp. 237-241. doi:10.1016/S0031-9422(00)83527-X [3] S. R. Schnebly and W. R. Fehr, “Effect of Years and Planting Dates on Fatty Acid Composition of Soybean Genotypes,” Crop Science, Vol. 33, No. 4, 1993, pp. 716-719. doi:10.2135/cropsci1993.0011183X003300040016x [4] G. Rakow and D. I. McGregor, “Opportunities and Prop- Blems in Modification of Levels of Rapeseed C18 Un- saturated Fatty Acid,” Journal of the American Oil Chemists’ Society, Vol. 50, 1973, pp. 400-403. [5] S. M. Rahman, T. Kinoshita, T. Anai and Y. Takagi, “Combining Ability in Loci for High Oleic and Low Li- nolenic Acids in Soybean,” Crop Science, Vol. 41, No. 1, 2001, pp. 26-29. doi:10.2135/cropsci2001.41126x [6] D. J. Pilbeam and E. A. Kirkby, “The Physiological Role of Boron in Plants,” Journal of Plant Nutrition, 1983, Vol. 6, No. 7, pp. 563-582. doi:10.1080/01904168309363126 [7] H. Marschner, “Mineral Nutrition of Higher Plants,” 2nd Edition, Academic Press, San Diego, 1995, pp. 379-396. [8] P. H. Brown, N. Bellaloui, H. Hu and A. Dandekar, “Transgenically Enhanced Sorbitol Synthesis Facilitates Phloem Boron Transport and Increases Tolerance of To- bacco to Boron Deficiency,” Plant Physiology, Vol. 119, No. 1, 1999, pp. 17-20. doi:10.1104/pp.119.1.17 [9] W. E. Brenchley and H. G. Thornton, “The Relation be- tween the Development, Structure and Functioning of the Nodules on Vicia faba, as Influenced by the Presence or Absence of Boron in the Nutrient Medium,” Proceedings of the Royal Society B Biological Sciences, Vol. 98, No. 691, 1925, pp. 373-398. doi:10.1098/rspb.1925.0043 [10] L. Bolafios, N. J. Brewin and I. Bonilla, “Effects of Bo- ron on Rhizobium-Legume Cell-Surface Interactions and Node Development,” Plant Physiology, Vol. 11, 1996, pp. 1249-1256. [11] L. Bolafios, E. Esteban, C. de Lorenzo, M. Fernández- Pascual, M. R. de Felipe, A. Gbrate and I. Bonilla, “Es- sentiality of Boron for Symbiotic Dinitrogen Fixation in Pea (Pisum sativum) Rhizobium Nodules,” Plant Physio- logy, Vol. 104, 1994, pp. 85-90. [12] Y. Kanayama, K. Kimura, Y. Nakamura and T. Ike, “Pu- rification and Characterization of Nitrate Reductase from Nodule Cytosol of Soybean Plants,” Physiologia Planta- Copyright © 2011 SciRes. AJPS
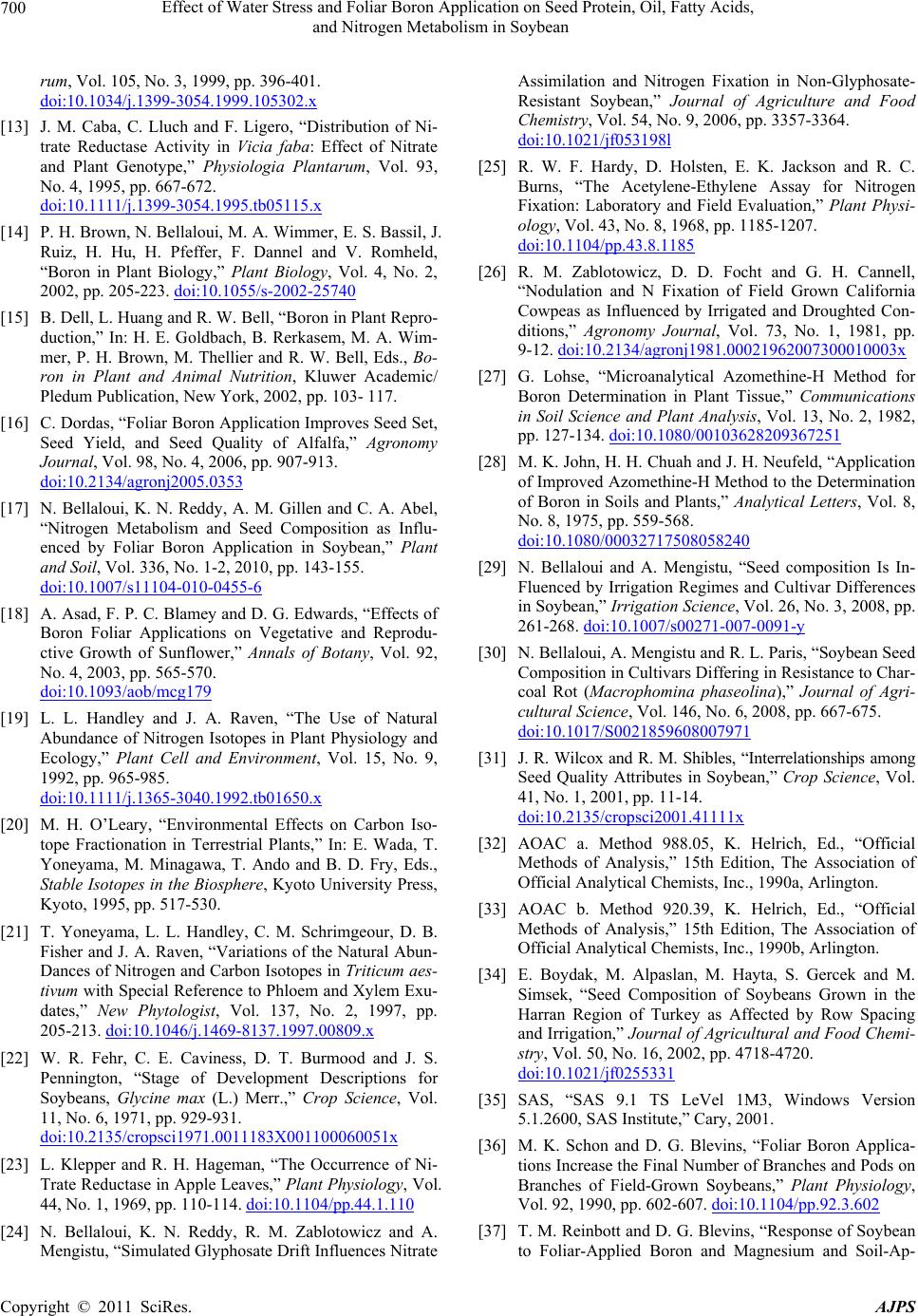 Effect of Water Stress and Foliar Boron Application on Seed Protein, Oil, Fatty Acids, 700 and Nitrogen Metabolism in Soybean rum, Vol. 105, No. 3, 1999, pp. 396-401. doi:10.1034/j.1399-3054.1999.105302.x [13] J. M. Caba, C. Lluch and F. Ligero, “Distribution of Ni- trate Reductase Activity in Vicia faba: Effect of Nitrate and Plant Genotype,” Physiologia Plantarum, Vol. 93, No. 4, 1995, pp. 667-672. doi:10.1111/j.1399-3054.1995.tb05115.x [14] P. H. Brown, N. Bellaloui, M. A. Wimmer, E. S. Bassil, J. Ruiz, H. Hu, H. Pfeffer, F. Dannel and V. Romheld, “Boron in Plant Biology,” Plant Biology, Vol. 4, No. 2, 2002, pp. 205-223. doi:10.1055/s-2002-25740 [15] B. Dell, L. Huang and R. W. Bell, “Boron in Plant Repro- duction,” In: H. E. Goldbach, B. Rerkasem, M. A. Wim- mer, P. H. Brown, M. Thellier and R. W. Bell, Eds., Bo- ron in Plant and Animal Nutrition, Kluwer Academic/ Pledum Publication, New York, 2002, pp. 103- 117. [16] C. Dordas, “Foliar Boron Application Improves Seed Set, Seed Yield, and Seed Quality of Alfalfa,” Agronomy Journal, Vol. 98, No. 4, 2006, pp. 907-913. doi:10.2134/agronj2005.0353 [17] N. Bellaloui, K. N. Reddy, A. M. Gillen and C. A. Abel, “Nitrogen Metabolism and Seed Composition as Influ- enced by Foliar Boron Application in Soybean,” Plant and Soil, Vol. 336, No. 1-2, 2010, pp. 143-155. doi:10.1007/s11104-010-0455-6 [18] A. Asad, F. P. C. Blamey and D. G. Edwards, “Effects of Boron Foliar Applications on Vegetative and Reprodu- ctive Growth of Sunflower,” Annals of Botany, Vol. 92, No. 4, 2003, pp. 565-570. doi:10.1093/aob/mcg179 [19] L. L. Handley and J. A. Raven, “The Use of Natural Abundance of Nitrogen Isotopes in Plant Physiology and Ecology,” Plant Cell and Environment, Vol. 15, No. 9, 1992, pp. 965-985. doi:10.1111/j.1365-3040.1992.tb01650.x [20] M. H. O’Leary, “Environmental Effects on Carbon Iso- tope Fractionation in Terrestrial Plants,” In: E. Wada, T. Yoneyama, M. Minagawa, T. Ando and B. D. Fry, Eds., Stable Isotopes in the Biosphere, Kyoto University Press, Kyoto, 1995, pp. 517-530. [21] T. Yoneyama, L. L. Handley, C. M. Schrimgeour, D. B. Fisher and J. A. Raven, “Variations of the Natural Abun- Dances of Nitrogen and Carbon Isotopes in Triticum aes- tivum with Special Reference to Phloem and Xylem Exu- dates,” New Phytologist, Vol. 137, No. 2, 1997, pp. 205-213. doi:10.1046/j.1469-8137.1997.00809.x [22] W. R. Fehr, C. E. Caviness, D. T. Burmood and J. S. Pennington, “Stage of Development Descriptions for Soybeans, Glycine max (L.) Merr.,” Crop Science, Vol. 11, No. 6, 1971, pp. 929-931. doi:10.2135/cropsci1971.0011183X001100060051x [23] L. Klepper and R. H. Hageman, “The Occurrence of Ni- Trate Reductase in Apple Leaves,” Plant Physiology, Vol. 44, No. 1, 1969, pp. 110-114. doi:10.1104/pp.44.1.110 [24] N. Bellaloui, K. N. Reddy, R. M. Zablotowicz and A. Mengistu, “Simulated Glyphosate Drift Influences Nitrate Assimilation and Nitrogen Fixation in Non-Glyphosate- Resistant Soybean,” Journal of Agriculture and Food Chemistry, Vol. 54, No. 9, 2006, pp. 3357-3364. doi:10.1021/jf053198l [25] R. W. F. Hardy, D. Holsten, E. K. Jackson and R. C. Burns, “The Acetylene-Ethylene Assay for Nitrogen Fixation: Laboratory and Field Evaluation,” Plant Physi- ology, Vol. 43, No. 8, 1968, pp. 1185-1207. doi:10.1104/pp.43.8.1185 [26] R. M. Zablotowicz, D. D. Focht and G. H. Cannell, “Nodulation and N Fixation of Field Grown California Cowpeas as Influenced by Irrigated and Droughted Con- ditions,” Agronomy Journal, Vol. 73, No. 1, 1981, pp. 9-12. doi:10.2134/agronj1981.00021962007300010003x [27] G. Lohse, “Microanalytical Azomethine-H Method for Boron Determination in Plant Tissue,” Communications in Soil Science and Plant Analysis, Vol. 13, No. 2, 1982, pp. 127-134. doi:10.1080/00103628209367251 [28] M. K. John, H. H. Chuah and J. H. Neufeld, “Application of Improved Azomethine-H Method to the Determination of Boron in Soils and Plants,” Analytical Letters, Vol. 8, No. 8, 1975, pp. 559-568. doi:10.1080/00032717508058240 [29] N. Bellaloui and A. Mengistu, “Seed composition Is In- Fluenced by Irrigation Regimes and Cultivar Differences in Soybean,” Irrigation Science, Vol. 26, No. 3, 2008, pp. 261-268. doi:10.1007/s00271-007-0091-y [30] N. Bellaloui, A. Mengistu and R. L. Paris, “Soybean Seed Composition in Cultivars Differing in Resistance to Char- coal Rot (Macrophomina phaseolina),” Journal of Agri- cultural Science, Vol. 146, No. 6, 2008, pp. 667-675. doi:10.1017/S0021859608007971 [31] J. R. Wilcox and R. M. Shibles, “Interrelationships among Seed Quality Attributes in Soybean,” Crop Science, Vol. 41, No. 1, 2001, pp. 11-14. doi:10.2135/cropsci2001.41111x [32] AOAC a. Method 988.05, K. Helrich, Ed., “Official Methods of Analysis,” 15th Edition, The Association of Official Analytical Chemists, Inc., 1990a, Arlington. [33] AOAC b. Method 920.39, K. Helrich, Ed., “Official Methods of Analysis,” 15th Edition, The Association of Official Analytical Chemists, Inc., 1990b, Arlington. [34] E. Boydak, M. Alpaslan, M. Hayta, S. Gercek and M. Simsek, “Seed Composition of Soybeans Grown in the Harran Region of Turkey as Affected by Row Spacing and Irrigation,” Journal of Agricultural and Food Chemi- stry, Vol. 50, No. 16, 2002, pp. 4718-4720. doi:10.1021/jf0255331 [35] SAS, “SAS 9.1 TS LeVel 1M3, Windows Version 5.1.2600, SAS Institute,” Cary, 2001. [36] M. K. Schon and D. G. Blevins, “Foliar Boron Applica- tions Increase the Final Number of Branches and Pods on Branches of Field-Grown Soybeans,” Plant Physiology, Vol. 92, 1990, pp. 602-607. doi:10.1104/pp.92.3.602 [37] T. M. Reinbott and D. G. Blevins, “Response of Soybean to Foliar-Applied Boron and Magnesium and Soil-Ap- Copyright © 2011 SciRes. AJPS
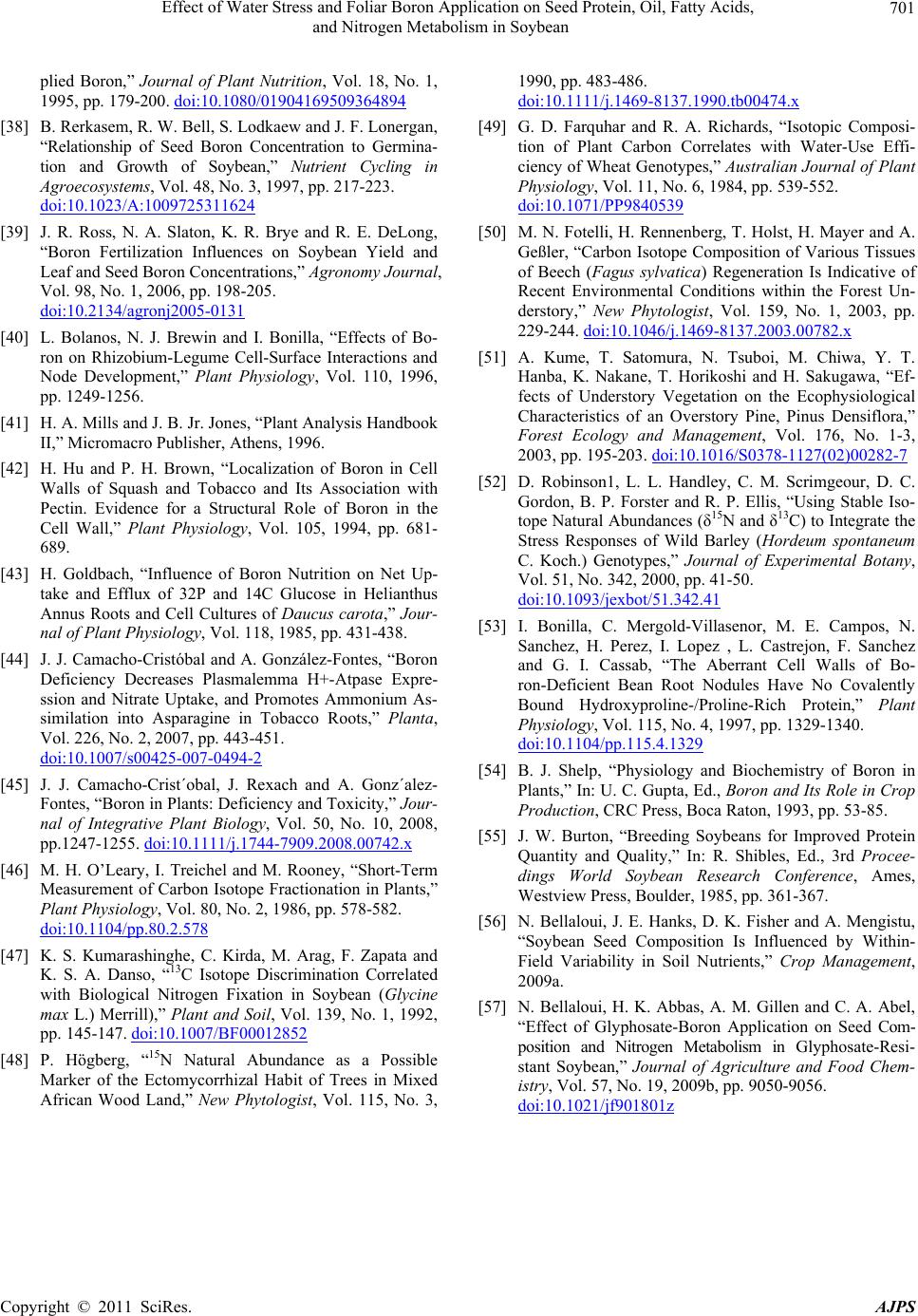 Effect of Water Stress and Foliar Boron Application on Seed Protein, Oil, Fatty Acids, and Nitrogen Metabolism in Soybean Copyright © 2011 SciRes. AJPS 701 plied Boron,” Journal of Plant Nutrition, Vol. 18, No. 1, 1995, pp. 179-200. doi:10.1080/01904169509364894 [38] B. Rerkasem, R. W. Bell, S. Lodkaew and J. F. Lonergan, “Relationship of Seed Boron Concentration to Germina- tion and Growth of Soybean,” Nutrient Cycling in Agroecosystems, Vol. 48, No. 3, 1997, pp. 217-223. doi:10.1023/A:1009725311624 [39] J. R. Ross, N. A. Slaton, K. R. Brye and R. E. DeLong, “Boron Fertilization Influences on Soybean Yield and Leaf and Seed Boron Concentrations,” Agronomy Journal, Vol. 98, No. 1, 2006, pp. 198-205. doi:10.2134/agronj2005-0131 [40] L. Bolanos, N. J. Brewin and I. Bonilla, “Effects of Bo- ron on Rhizobium-Legume Cell-Surface Interactions and Node Development,” Plant Physiology, Vol. 110, 1996, pp. 1249-1256. [41] H. A. Mills and J. B. Jr. Jones, “Plant Analysis Handbook II,” Micromacro Publisher, Athens, 1996. [42] H. Hu and P. H. Brown, “Localization of Boron in Cell Walls of Squash and Tobacco and Its Association with Pectin. Evidence for a Structural Role of Boron in the Cell Wall,” Plant Physiology, Vol. 105, 1994, pp. 681- 689. [43] H. Goldbach, “Influence of Boron Nutrition on Net Up- take and Efflux of 32P and 14C Glucose in Helianthus Annus Roots and Cell Cultures of Daucus carota,” Jour- nal of Plant Physiology, Vol. 118, 1985, pp. 431-438. [44] J. J. Camacho-Cristóbal and A. González-Fontes, “Boron Deficiency Decreases Plasmalemma H+-Atpase Expre- ssion and Nitrate Uptake, and Promotes Ammonium As- similation into Asparagine in Tobacco Roots,” Planta, Vol. 226, No. 2, 2007, pp. 443-451. doi:10.1007/s00425-007-0494-2 [45] J. J. Camacho-Crist´obal, J. Rexach and A. Gonz´alez- Fontes, “Boron in Plants: Deficiency and Toxicity,” Jour- nal of Integrative Plant Biology, Vol. 50, No. 10, 2008, pp.1247-1255. doi:10.1111/j.1744-7909.2008.00742.x [46] M. H. O’Leary, I. Treichel and M. Rooney, “Short-Term Measurement of Carbon Isotope Fractionation in Plants,” Plant Physiology, Vol. 80, No. 2, 1986, pp. 578-582. doi:10.1104/pp.80.2.578 [47] K. S. Kumarashinghe, C. Kirda, M. Arag, F. Zapata and K. S. A. Danso, “13C Isotope Discrimination Correlated with Biological Nitrogen Fixation in Soybean (Glycine max L.) Merrill),” Plant and Soil, Vol. 139, No. 1, 1992, pp. 145-147. doi:10.1007/BF00012852 [48] P. Högberg, “15N Natural Abundance as a Possible Marker of the Ectomycorrhizal Habit of Trees in Mixed African Wood Land,” New Phytologist, Vol. 115, No. 3, 1990, pp. 483-486. doi:10.1111/j.1469-8137.1990.tb00474.x [49] G. D. Farquhar and R. A. Richards, “Isotopic Composi- tion of Plant Carbon Correlates with Water-Use Effi- ciency of Wheat Genotypes,” Australian Journal of Plant Physiology, Vol. 11, No. 6, 1984, pp. 539-552. doi:10.1071/PP9840539 [50] M. N. Fotelli, H. Rennenberg, T. Holst, H. Mayer and A. Geßler, “Carbon Isotope Composition of Various Tissues of Beech (Fagus sylvatica) Regeneration Is Indicative of Recent Environmental Conditions within the Forest Un- derstory,” New Phytologist, Vol. 159, No. 1, 2003, pp. 229-244. doi:10.1046/j.1469-8137.2003.00782.x [51] A. Kume, T. Satomura, N. Tsuboi, M. Chiwa, Y. T. Hanba, K. Nakane, T. Horikoshi and H. Sakugawa, “Ef- fects of Understory Vegetation on the Ecophysiological Characteristics of an Overstory Pine, Pinus Densiflora,” Forest Ecology and Management, Vol. 176, No. 1-3, 2003, pp. 195-203. doi:10.1016/S0378-1127(02)00282-7 [52] D. Robinson1, L. L. Handley, C. M. Scrimgeour, D. C. Gordon, B. P. Forster and R. P. Ellis, “Using Stable Iso- tope Natural Abundances (δ15N and δ13C) to Integrate the Stress Responses of Wild Barley (Hordeum spontaneum C. Koch.) Genotypes,” Journal of Experimental Botany, Vol. 51, No. 342, 2000, pp. 41-50. doi:10.1093/jexbot/51.342.41 [53] I. Bonilla, C. Mergold-Villasenor, M. E. Campos, N. Sanchez, H. Perez, I. Lopez , L. Castrejon, F. Sanchez and G. I. Cassab, “The Aberrant Cell Walls of Bo- ron-Deficient Bean Root Nodules Have No Covalently Bound Hydroxyproline-/Proline-Rich Protein,” Plant Physiology, Vol. 115, No. 4, 1997, pp. 1329-1340. doi:10.1104/pp.115.4.1329 [54] B. J. Shelp, “Physiology and Biochemistry of Boron in Plants,” In: U. C. Gupta, Ed., Boron and Its Role in Crop Production, CRC Press, Boca Raton, 1993, pp. 53-85. [55] J. W. Burton, “Breeding Soybeans for Improved Protein Quantity and Quality,” In: R. Shibles, Ed., 3rd Procee- dings World Soybean Research Conference, Ames, Westview Press, Boulder, 1985, pp. 361-367. [56] N. Bellaloui, J. E. Hanks, D. K. Fisher and A. Mengistu, “Soybean Seed Composition Is Influenced by Within- Field Variability in Soil Nutrients,” Crop Management, 2009a. [57] N. Bellaloui, H. K. Abbas, A. M. Gillen and C. A. Abel, “Effect of Glyphosate-Boron Application on Seed Com- position and Nitrogen Metabolism in Glyphosate-Resi- stant Soybean,” Journal of Agriculture and Food Chem- istry, Vol. 57, No. 19, 2009b, pp. 9050-9056. doi:10.1021/jf901801z
|