 Materials Science s a nd Applications, 2011, 2, 1529-1541 doi:10.4236/msa.2011.211206 Published Online November 2011 (http://www.SciRP.org/journal/msa) Copyright © 2011 SciRes. MSA 1529 Microstructural and Mechanical Characterization after Thermomechanical Treatments in 6063 Aluminum Alloy Waldemar A. Monteiro1,2*, Iara M. Espósito1, Ricardo B. Ferrari1, Sidnei J. Buso1 1Science and Technology Materials Center, Nuclear and Energetic Research Institute, IPEN, São Paulo, Brazil; 2Science and Hu- manities Center, Presbyterian Mackenzie University, UPM, São Paulo, Brazil. Email: *tecnologia@mackenzie.br Received S eptember 5th, 2011; revised October 16th, 201 1; accepted October 24th, 2011. ABSTRACT The aim of this work is the mechanical and microstructural characterization by optical and electron microscopy as well as microhardness of Al 6063 alloy after mechanical and thermal treatment. Al-Mg based alloys have special attention due to the lightness of the material and certain mechanical properties and recyclability. Such alloys produce good me- chanical properties in moderate mechanical efforts (up to 700 MPa) and good resistance to the corrosion. Cold rolling steps (30%, 60% and 90% in area reduction) in Al 6063 alloy were employed for th e recrystallizatio n studies, followed by thermal treatment using four isothermal heating (423 K, 523 K, 623 K and 723 K) during 1800, 3600, 5400 and 7200 s. The direct observation and chemical microanalysis were made in a JEOL200C and JEOL2010 transmission electron microscopes combined with mechanical characterization utilizing Vickers microhardness measurements. Normally classified as non-heat-treatable these alloys obtain higher strength either by strain-hardening or by solid solution. The nucleation of new grains is a non stability of the deformed microstructure, depending on subgrain size heterogeneities present as potential embryos in the deformed state adjacent to high local misorientation. The results indicate a significant effect of second-phase particles on recrystallization and how to control the resulting microstruc- ture and texture by the use of particles. It may be a preferential growth in the early stage due to their local environment or a selection of certain orientations from among those produced by particles stimulated nucleation or a preferential nucleation at particles in favored sites such as grain boundaries. Keywords: 6063 Aluminum Alloy, Optical Microscopy, Electron Microscopy, Hardness 1. Introduction The first register of production of an Al-6000 alloy was 1921 when was produced the Al 6051 alloy with levels of 1 wt% Si and 0.5 wt% Mg, that is one of the earliest aluminum alloy aged without copper. The Al 6063 alloy, containing much smaller levels of magnesi um and silico n (Table 1), was first produced after 23 years of the first series. This is an alloy that has a good potential of hardening by precipitation (heat treatable) and has a high ductility creep providing elevated capacity using extrusion work. This alloy, as in all of 6000 series, the presence of in- termetallic compound, Mg2Si, is responsible for the hard- ening of these alloys. This hardening occurs via solution treatment and artificial aging, which provides growth control and a consistently composed in aluminum-rich matrix [1-4]. However, if there is excessive growth of these pre- cipitates through treatments at high temperatures or very long times, facilitating movement that take place from inconsistencies in the alloy softening. This treatment is well known by over aging. Thermomechanical processing consists in cold or hot-deformed state on material and subsequently by heat treatment that changes its microstructure and conse- quently its physical properties such as hardness, thermal, electrical conductivity and corrosion resistance. This is particularly important for Al 6063 alloy where small par- ticles of second stage (normally Mg2Si) dispersed within the matrix which changes their mechanical stre n gth. When metals are subjected to the plastic deformation process (tension or compression, shear, for example) the stored energy is largely lost as heat and only two to ten
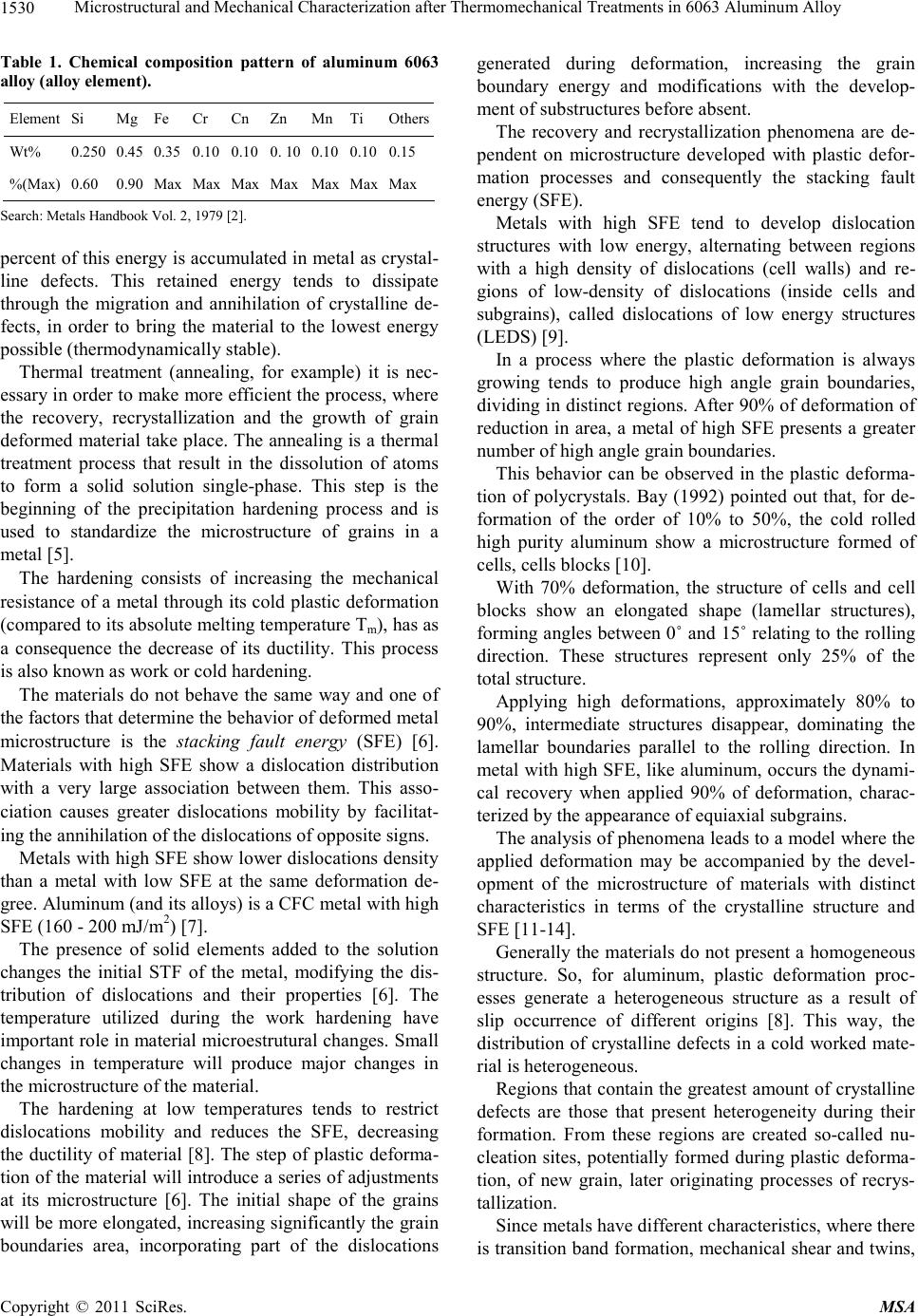 Microst ructural and Mechanical Characterizatio n after Thermomechani cal Treatments in 6063 Aluminum Alloy 1530 Table 1. Chemical composition pattern of aluminum 6063 alloy (alloy element). Element Si Mg Fe Cr Cn Zn Mn Ti Others Wt% 0.250 0.45 0.35 0.10 0.10 0. 10 0.10 0.100.15 %(Max) 0.60 0.90 Max Max Max Max Max Max Max Search: Metals Handbook Vol. 2, 1979 [2]. percent of this energy is accumulated in metal as crystal- line defects. This retained energy tends to dissipate through the migration and annihilation of crystalline de- fects, in order to bring the material to the lowest energy possible (thermodynamically stable). Thermal treatment (annealing, for example) it is nec- essary in order to make more efficient the process, where the recovery, recrystallization and the growth of grain deformed material take place. The annealing is a thermal treatment process that result in the dissolution of atoms to form a solid solution single-phase. This step is the beginning of the precipitation hardening process and is used to standardize the microstructure of grains in a metal [5]. The hardening consists of increasing the mechanical resistance of a metal through its cold plastic deformation (compared to its absolute melting temperature Tm), has as a consequence the decrease of its ductility. This process is also known as work or cold hardeni ng. The materials do not behave the same way and one of the factors that determine the behavior of deformed metal microstructure is the stacking fault energy (SFE) [6]. Materials with high SFE show a dislocation distribution with a very large association between them. This asso- ciation causes greater dislocations mobility by facilitat- ing the annihilation of the dislocations of opposite signs. Metals with high S FE show lower dislocations de nsity than a metal with low SFE at the same deformation de- gree. Aluminum (and its alloys) is a CFC metal with high SFE (160 - 200 mJ/m2) [7]. The presence of solid elements added to the solution changes the initial STF of the metal, modifying the dis- tribution of dislocations and their properties [6]. The temperature utilized during the work hardening have important role in material microestrutural changes. Small changes in temperature will produce major changes in the microstructure of the material. The hardening at low temperatures tends to restrict dislocations mobility and reduces the SFE, decreasing the ductility of material [8]. The step of plastic deforma- tion of the material will introduce a series of adjustments at its microstructure [6]. The initial shape of the grains will be more elongated, increasing significantly the grain boundaries area, incorporating part of the dislocations generated during deformation, increasing the grain boundary energy and modifications with the develop- ment of substructures before absent. The recovery and recrystallization phenomena are de- pendent on microstructure developed with plastic defor- mation processes and consequently the stacking fault energy (SFE). Metals with high SFE tend to develop dislocation structures with low energy, alternating between regions with a high density of dislocations (cell walls) and re- gions of low-density of dislocations (inside cells and subgrains), called dislocations of low energy structures (LEDS) [9]. In a process where the plastic deformation is always growing tends to produce high angle grain boundaries, dividing in distinct regions. After 90% of deformation of reduction in area, a metal of high SFE presents a greater number of high a ngle grain bound aries. This behavior can be observed in the plastic deforma- tion of polycrystals. Bay (1992) pointed out that, for de- formation of the order of 10% to 50%, the cold rolled high purity aluminum show a microstructure formed of cells, cells blocks [10]. With 70% deformation, the structure of cells and cell blocks show an elongated shape (lamellar structures), forming angles between 0˚ and 15˚ relating to the rolling direction. These structures represent only 25% of the total structure. Applying high deformations, approximately 80% to 90%, intermediate structures disappear, dominating the lamellar boundaries parallel to the rolling direction. In metal with high SFE, like aluminum, occurs the dynami- cal recovery when applied 90% of deformation, charac- terized by the appearance of equiaxial subgrains. The analysis of phenomena leads to a model where the applied deformation may be accompanied by the devel- opment of the microstructure of materials with distinct characteristics in terms of the crystalline structure and SFE [11-14]. Gener ally the materia ls do no t pre sent a ho moge neous structure. So, for aluminum, plastic deformation proc- esses generate a heterogeneous structure as a result of slip occurrence of different origins [8]. This way, the distribution of crystalline defects in a cold worked mate- rial is heterogeneous. Regions that contain the greatest amount of crystalline defects are those that present heterogeneity during their formation. From these regions are created so-called nu- cleation sites, potentially formed during plastic deforma- tion, of new grain, later originating processes of recrys- tallization. Since metals have different characteristics, where there is transition band formation, mechanical shear and twins, Copyright © 2011 SciRes. MSA
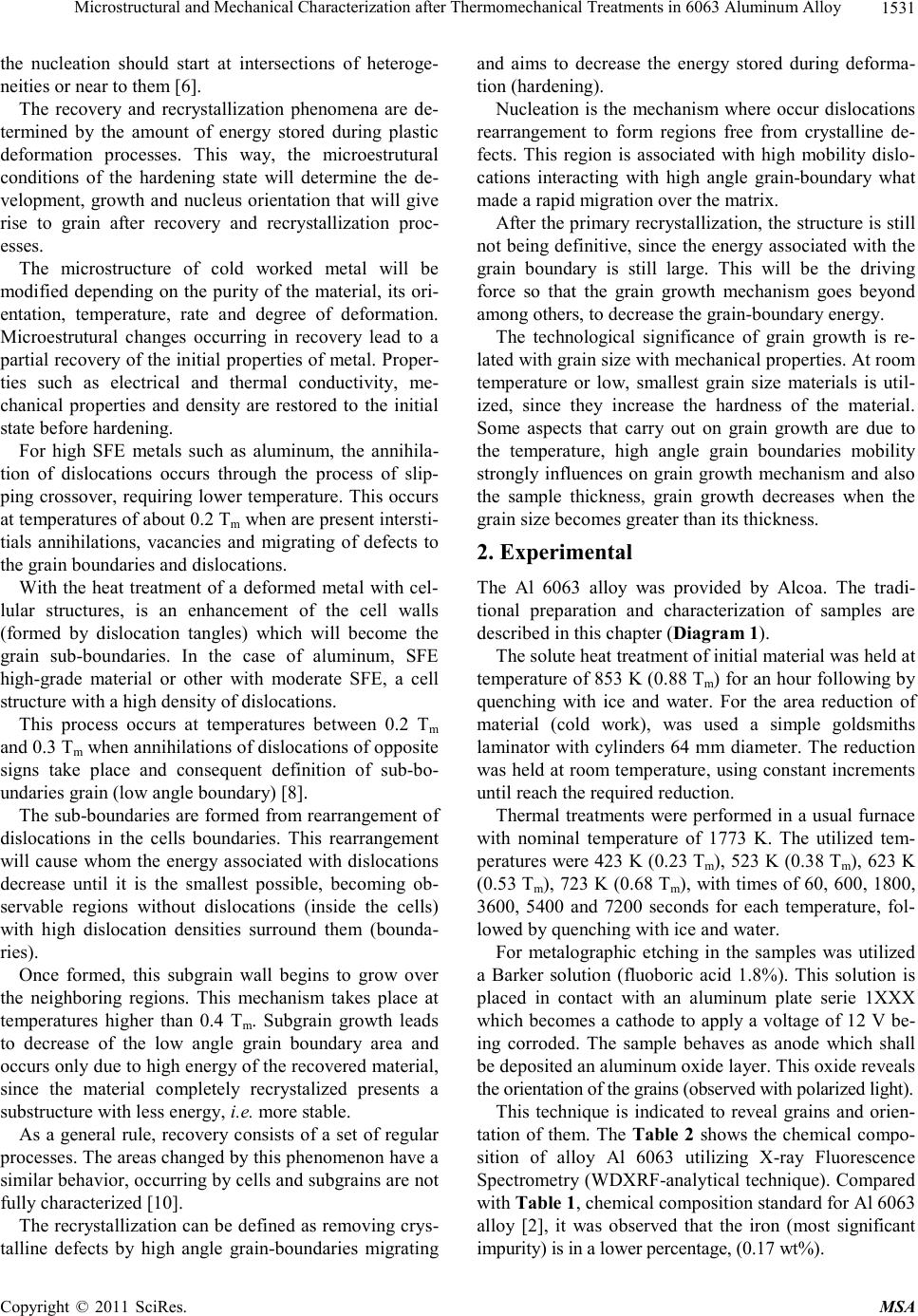 Microst ructural and Mechanical Characterizatio n after Thermomechani cal Treatments in 6063 Aluminum Alloy1531 the nucleation should start at intersections of heteroge- neities or near to them [6]. The recovery and recrystallization phenomena are de- termined by the amount of energy stored during plastic deformation processes. This way, the microestrutural conditions of the hardening state will determine the de- velop ment, gro wth and nuc leus orientation t hat will give rise to grain after recovery and recrystallization proc- esses. The microstructure of cold worked metal will be modified depending on the purity of the material, its ori- entation, temperature, rate and degree of deformation. Microestrutural changes occurring in recovery lead to a partial recovery of the initial properties of metal. Proper- ties such as electrical and thermal conductivity, me- chanical properties and density are restored to the initial state before hardening. For high SFE metals such as aluminum, the annihila- tion of dislocations occurs through the process of slip- ping crossover, requiring lower temperature. This occurs at temperatures of about 0.2 Tm when are present intersti- tials annihilations, vacancies and migrating of defects to the gra in boundar i es and dislo catio ns. With the heat treatment of a deformed metal with cel- lular structures, is an enhancement of the cell walls (formed by dislocation tangles) which will become the grain sub-boundaries. In the case of aluminum, SFE high-grade material or other with moderate SFE, a cell structure with a high density of dislocations. This process occurs at temperatures between 0.2 Tm and 0.3 Tm when annihilations of dislocations of opposite signs take place and consequent definition of sub-bo- undaries grain (low angle boundary) [8]. The sub-boundaries are formed from rearrangement of dislocations in the cells boundaries. This rearrangement will cause whom the energy associated with dislocations decrease until it is the smallest possible, becoming ob- servable regions without dislocations (inside the cells) with high dislocation densities surround them (bounda- ries). Once formed, this subgrain wall begins to grow over the neighboring regions. This mechanism takes place at temperatures higher than 0.4 Tm. Subgrain growth leads to decrease of the low angle grain boundary area and occurs only due to high energy of the recovered material, since the material completely recrystalized presents a substructure with less energy, i.e. more stable. As a general rule, recovery consists of a set of regular processes. The areas changed by this phenomenon have a similar behavior, occurring by cells and subgrains are not fully characterized [10]. The recrystallization can be defined as removing crys- talline defects by high angle grain-boundaries migrating and aims to decrease the energy stored during deforma- tion (hardening). Nucleation is the mechanism where occur dislocations rearrangement to form regions free from crystalline de- fects. This region is associated with high mobility dislo- cations interacting with high angle grain-boundary what made a rapid migration over the matrix. After the primary recrystallization, the structure is still not being definitive, since the energy associated with the grain boundary is still large. This will be the driving force so that the grain growth mechanism goes beyond among others, to decrease the grain-boundary energy. The technological significance of grain growth is re- lated with grain size with mechanical properties. At room temperature or low, smallest grain size materials is util- ized, since they increase the hardness of the material. Some aspects that carry out on grain growth are due to the temperature, high angle grain boundaries mobility strongly influences on grain growth mechanism and also the sample thickness, grain growth decreases when the grain size becomes greater than its thickness. 2. Experimental The Al 6063 alloy was provided by Alcoa. The tradi- tional preparation and characterization of samples are described in this chapter (Diagram 1). The solute heat treatment of initial material was held at temperature of 853 K (0.88 Tm) for an hour follo wing b y quenching with ice and water. For the area reduction of material (cold work), was used a simple goldsmiths laminator with cylinders 64 mm diameter. The reduction was held at room temperature, using constant increments until reach the required reduction. Thermal treatments were performed in a usual furnace with nominal temperature of 1773 K. The utilized tem- peratures were 423 K (0.23 Tm), 523 K (0.38 Tm), 623 K (0.53 Tm), 723 K (0.68 Tm), with times of 60, 600, 1800, 3600, 5400 and 7200 seconds for each temperature, fol- lowed by quenching with ice and water. For metalographic etching in the samples was utilized a Barker solution (fluoboric acid 1.8%). This solution is placed in contact with an aluminum plate serie 1XXX which becomes a cathode to apply a voltage of 12 V be- ing corroded. The sample behaves as anode which shall be deposited an aluminum oxide layer. This oxide reveals the orientation of th e gr ain s ( observ ed with polarized ligh t) . This technique is indicated to reveal grains and orien- tation of them. The Table 2 shows the chemical compo- sition of alloy Al 6063 utilizing X-ray Fluorescence Spectrometry (WDXRF-analytical technique). Compared with Table 1 , chemical composition standard for Al 6063 alloy [2], it was observed that the iron (most significant impurity) is in a lower percentage, (0.17 wt%). Copyright © 2011 SciRes. MSA
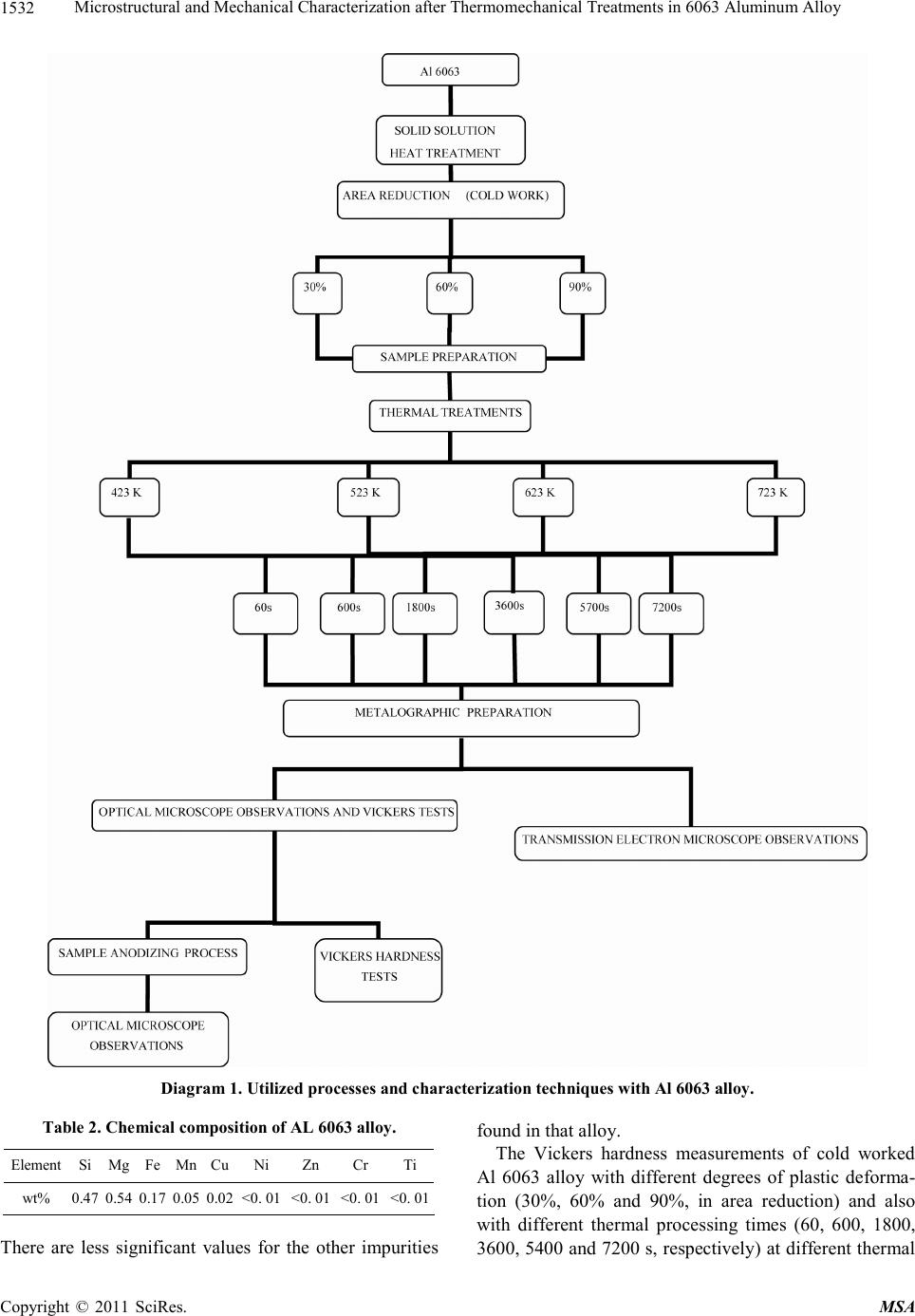 Microst ructural and Mechanical Characterizatio n after Thermomechani cal Treatments in 6063 Aluminum Alloy Copyright © 2011 SciRes. MSA 1532 Diagram 1. Uti lized processes and characterization tech niques with Al 6063 alloy. Table 2. Chemical composition of AL 6063 alloy. Element Si Mg Fe Mn CuNi Zn Cr Ti wt% 0.47 0.54 0.17 0.05 0.02<0. 01 <0. 01 <0. 01<0. 01 There are less significant values for the other impurities found in that alloy. The Vickers hardness measurements of cold worked Al 6063 alloy with different degrees of plastic deforma- tion (30%, 60% and 90%, in area reduction) and also with different thermal processing times (60, 600, 1800, 3600, 5400 and 7200 s, respectively) at different thermal
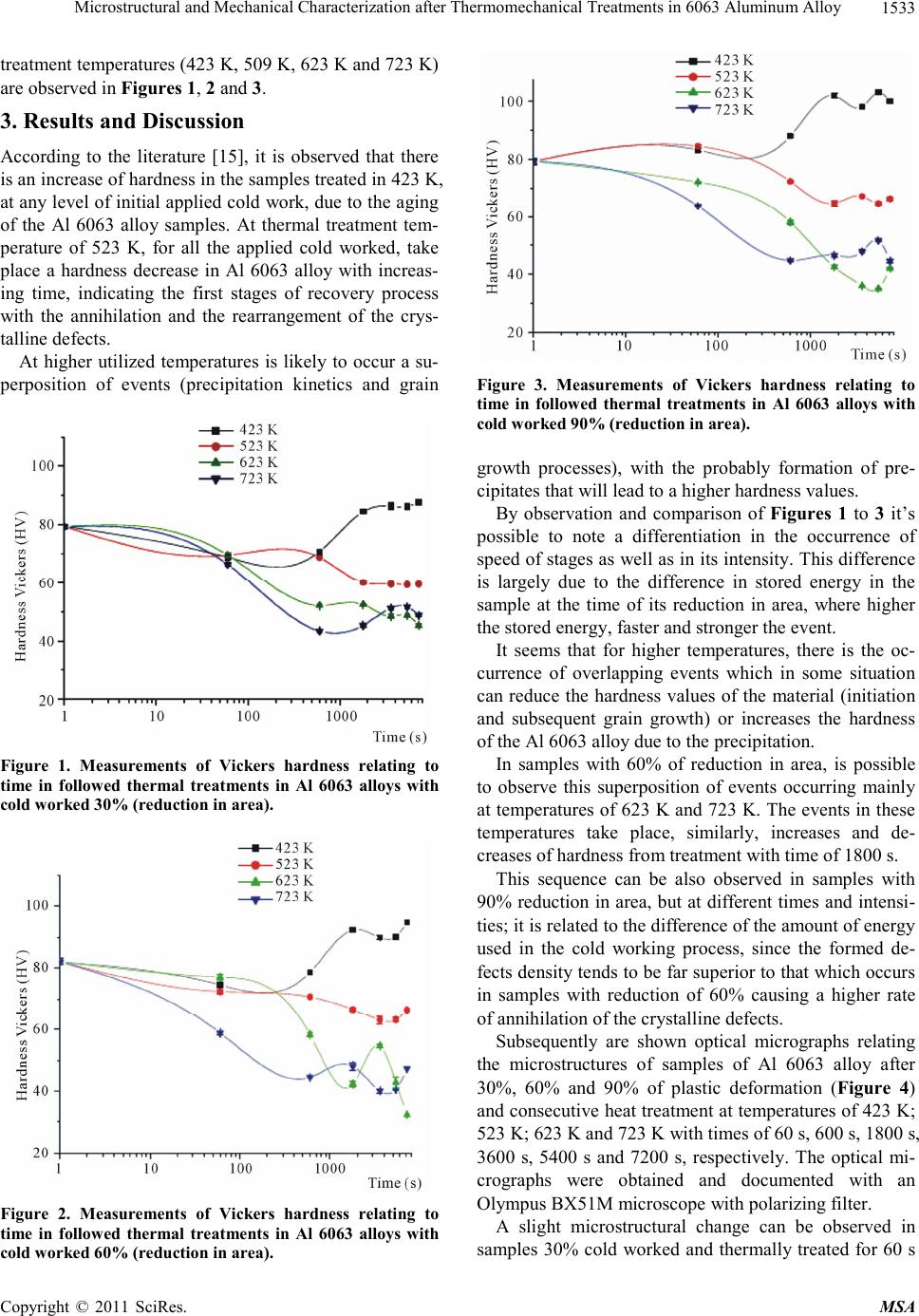 Microst ructural and Mechanical Characterizatio n after Thermomechani cal Treatments in 6063 Aluminum Alloy1533 treatment temperatures (423 K, 509 K, 623 K and 723 K) are observed in Figures 1, 2 and 3. 3. Results and Discussion According to the literature [15], it is observed that there is an increase of hardness in the samples treated in 423 K, at any level of initial applied cold work, due to the aging of the Al 6063 alloy samples. At thermal treatment tem- perature of 523 K, for all the applied cold worked, take place a hardness decrease in Al 6063 alloy with increas- ing time, indicating the first stages of recovery process with the annihilation and the rearrangement of the crys- talline defects. At higher utilized temperatures is likely to occur a su- perposition of events (precipitation kinetics and grain Figure 1. Measurements of Vickers hardness relating to time in followed thermal treatments in Al 6063 alloys with cold worked 30% (reduction in area). Figure 2. Measurements of Vickers hardness relating to time in followed thermal treatments in Al 6063 alloys with cold worked 60% (reduction in area). Figure 3. Measurements of Vickers hardness relating to time in followed thermal treatments in Al 6063 alloys with cold worked 90% (reduction in area). growth processes), with the probably formation of pre- cipitates that will lead to a higher hardness values. By observation and comparison of Figures 1 to 3 it’s possible to note a differentiation in the occurrence of speed of stages as well as in its intensity. This difference is largely due to the difference in stored energy in the sample at the time of its reduction in area, where higher the stored energy, faster and stronger the event. It seems that for higher temperatures, there is the oc- currence of overlapping events which in some situation can reduce the hardness values of the material (initiation and subsequent grain growth) or increases the hardness of the Al 6063 alloy due to the precipitation. In samples with 60% of reduction in area, is possible to observe this superposition of events occurring mainly at temperatures of 623 K and 723 K. The events in these temperatures take place, similarly, increases and de- creases of hardness from treatment with time of 1800 s. This sequence can be also observed in samples with 90% reduction in area, but at different times and intensi- ties; it is related to the difference of the amount of energy used in the cold working process, since the formed de- fects density tends to be far sup erior to that which occur s in samples with reduction of 60% causing a higher rate of annihilation of the crystalline defects. Subsequently are shown optical micrographs relating the microstructures of samples of Al 6063 alloy after 30%, 60% and 90% of plastic deformation (Figure 4) and consecutive heat treatment at temperatures of 423 K; 523 K; 623 K and 723 K with times of 60 s, 600 s, 1800 s, 3600 s, 5400 s and 7200 s, respectively. The optical mi- crographs were obtained and documented with an Olympus BX51M microscope with polarizing filter. A slight microstructural change can be observed in samples 30% cold worked and thermally treated for 60 s Copyright © 2011 SciRes. MSA
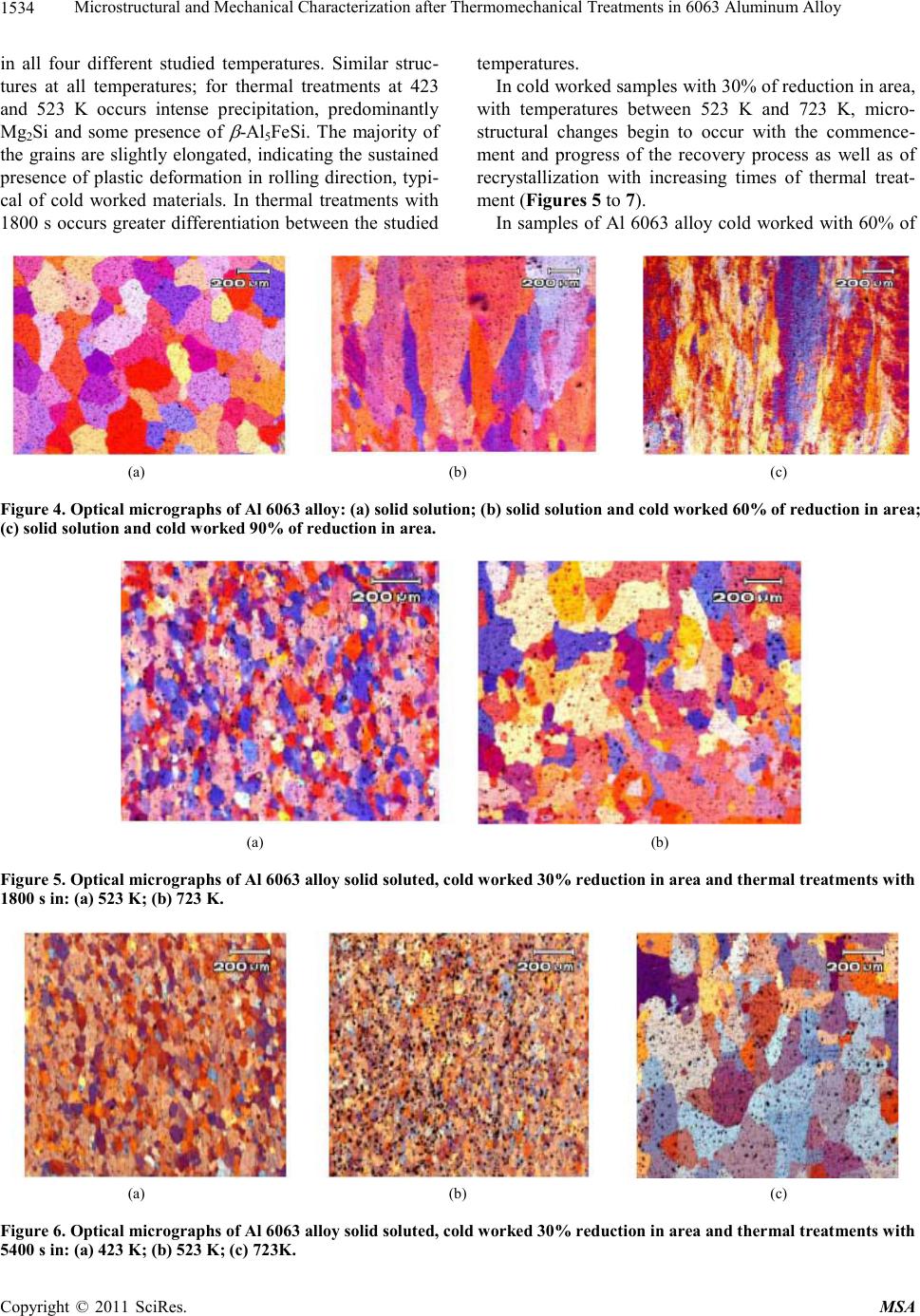 Microst ructural and Mechanical Characterizatio n after Thermomechani cal Treatments in 6063 Aluminum Alloy Copyright © 2011 SciRes. MSA 1534 in all four different studied temperatures. Similar struc- tures at all temperatures; for thermal treatments at 423 and 523 K occurs intense precipitation, predominantly Mg2Si and some presence of -Al5FeSi. The majority of the grains are slightly elongated, indicating the sustained presence of plastic deformation in rolling direction, typi- cal of cold worked materials. In thermal treatments with 1800 s occurs greater differentiation between the studied temperatures. In cold worked samples with 30% of reduction in area, with temperatures between 523 K and 723 K, micro- structural changes begin to occur with the commence- ment and progress of the recovery process as well as of recrystallization with increasing times of thermal treat- ment (Figures 5 to 7). In samples of Al 6063 alloy cold worked with 60% of (a) (b) (c) Figure 4. Optical micrographs of Al 6063 alloy: (a) solid solution; (b) solid solution and cold worked 60% of reduction in area; (c) solid solution a nd cold w orked 90% of reduction in area. (a) (b) Figure 5. Optical micrographs of Al 6063 alloy solid soluted, cold worked 30% reduction in area and thermal treatments with 1800 s in: (a) 523 K; (b) 723 K. (a) (b) (c) Figure 6. Optical micrographs of Al 6063 alloy solid soluted, cold worked 30% reduction in area and thermal treatments with 400 s in: (a) 423 K; (b) 523 K; (c) 723K. 5
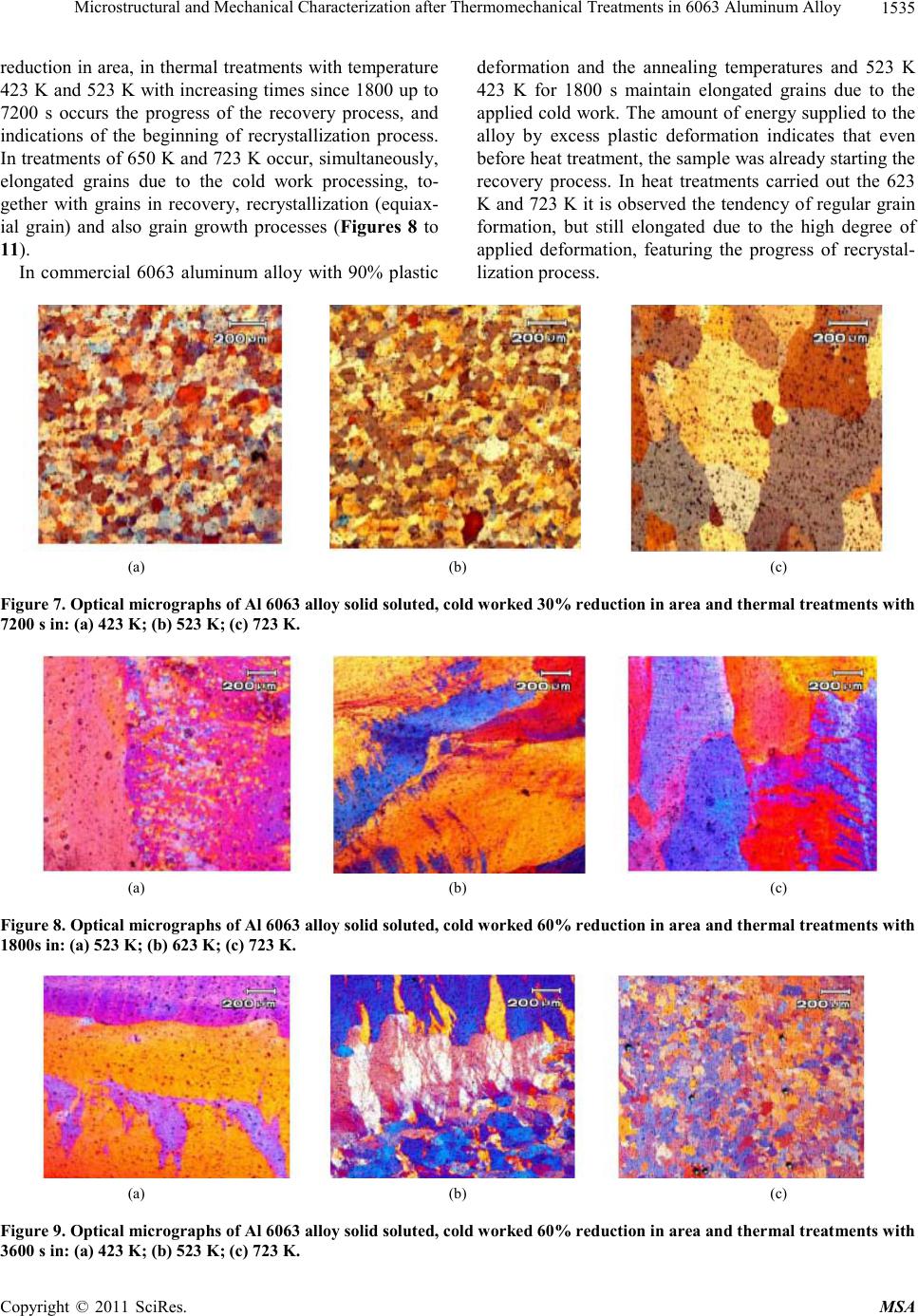 Microst ructural and Mechanical Characterizatio n after Thermomechani cal Treatments in 6063 Aluminum Alloy1535 reduction in area, in thermal treatments with temperature 423 K and 523 K with increasing times since 1800 up to 7200 s occurs the progress of the recovery process, and indications of the beginning of recrystallization process. In treatments of 650 K and 723 K occur, simultaneously, elongated grains due to the cold work processing, to- gether with grains in recovery, recrystallization (equiax- ial grain) and also grain growth processes (Figures 8 to 11). In commercial 6063 aluminum alloy with 90% plastic deformation and the annealing temperatures and 523 K 423 K for 1800 s maintain elongated grains due to the applied cold work. The amount of energy supplied to the alloy by excess plastic deformation indicates that even before heat treatment, the sample was already starting the recovery process. In heat treatments carried out the 623 K and 723 K it is observed the tendency of regular grain formation, but still elongated due to the high degree of applied deformation, featuring the progress of recrystal- lization process. (a) (b) (c) Figure 7. Optical micrographs of Al 6063 alloy solid soluted, cold worked 30% reduction in area and thermal treatments with 7200 s in: (a) 423 K; (b) 523 K; (c) 723 K. (a) (b) (c) Figure 8. Optical micrographs of Al 6063 alloy solid soluted, cold worked 60% reduction in area and thermal treatments with 1800s in: (a) 523 K; (b) 623 K; (c) 723 K. (a) (b) (c) Figure 9. Optical micrographs of Al 6063 alloy solid soluted, cold worked 60% reduction in area and thermal treatments with 3600 s in: (a) 423 K; (b) 523 K; (c) 723 K. Copyright © 2011 SciRes. MSA
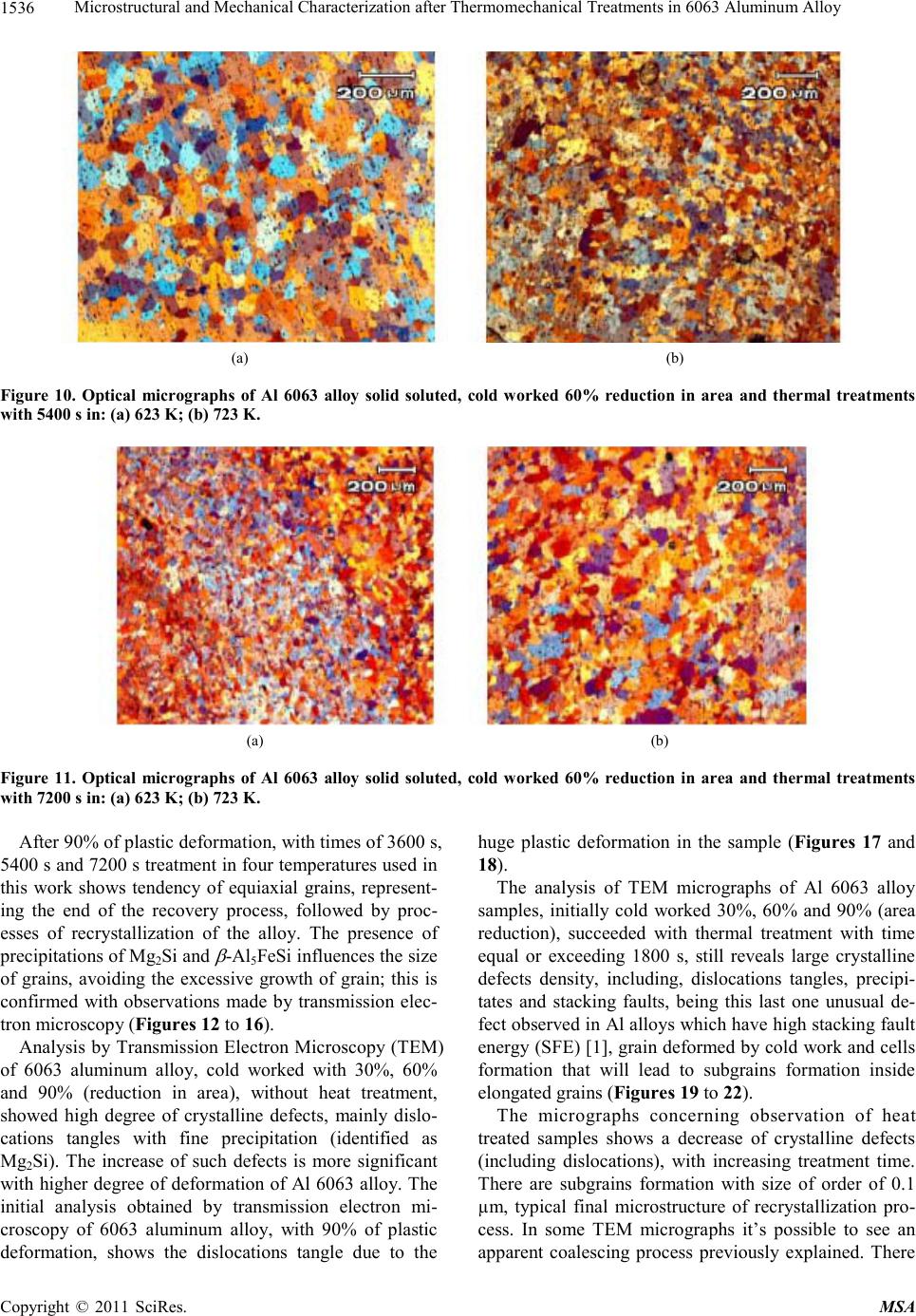 Microst ructural and Mechanical Characterizatio n after Thermomechani cal Treatments in 6063 Aluminum Alloy 1536 (a) (b) Figure 10. Optical micrographs of Al 6063 alloy solid soluted, cold worked 60% reduction in area and thermal treatments with 5400 s in: (a) 623 K; (b) 723 K. (a) (b) Figure 11. Optical micrographs of Al 6063 alloy solid soluted, cold worked 60% reduction in area and thermal treatments with 7200 s in: (a) 623 K; (b) 723 K. After 90% of plastic deformation, with times of 3600 s, 5400 s and 7200 s treatment in four temperatures used in this work shows tendency of equiaxial grains, represent- ing the end of the recovery process, followed by proc- esses of recrystallization of the alloy. The presence of precipitations of Mg2Si a nd -Al5FeSi in fluence s the size of grains, avoiding the excessive growth of grain; this is confirmed with observations made by transmission elec- tron microscopy (Figures 12 to 16). Analysis by Transmission Electron Microscopy (TEM) of 6063 aluminum alloy, cold worked with 30%, 60% and 90% (reduction in area), without heat treatment, showed high degree of crystalline defects, mainly dislo- cations tangles with fine precipitation (identified as Mg2Si). The increase of such defects is more significant with higher degree of deformation of Al 6063 alloy. The initial analysis obtained by transmission electron mi- croscopy of 6063 aluminum alloy, with 90% of plastic deformation, shows the dislocations tangle due to the huge plastic deformation in the sample (Figures 17 and 18). The analysis of TEM micrographs of Al 6063 alloy samples, initially cold worked 30%, 60% and 90% (area reduction), succeeded with thermal treatment with time equal or exceeding 1800 s, still reveals large crystalline defects density, including, dislocations tangles, precipi- tates and stacking faults, being this last one unusual de- fect ob served in Al allo ys which ha ve high sta ckin g fault energy (SFE) [1], grain deformed by cold work and cells formation that will lead to subgrains formation inside elongated grai ns (Figures 19 to 22). The micrographs concerning observation of heat treated samples shows a decrease of crystalline defects (including dislocations), with increasing treatment time. There are subgrains formation with size of order of 0.1 µm, typical final microstructure of recrystallization pro- cess. In some TEM micrographs it’s possible to see an apparent coalescing process previously explained. There Copyright © 2011 SciRes. MSA
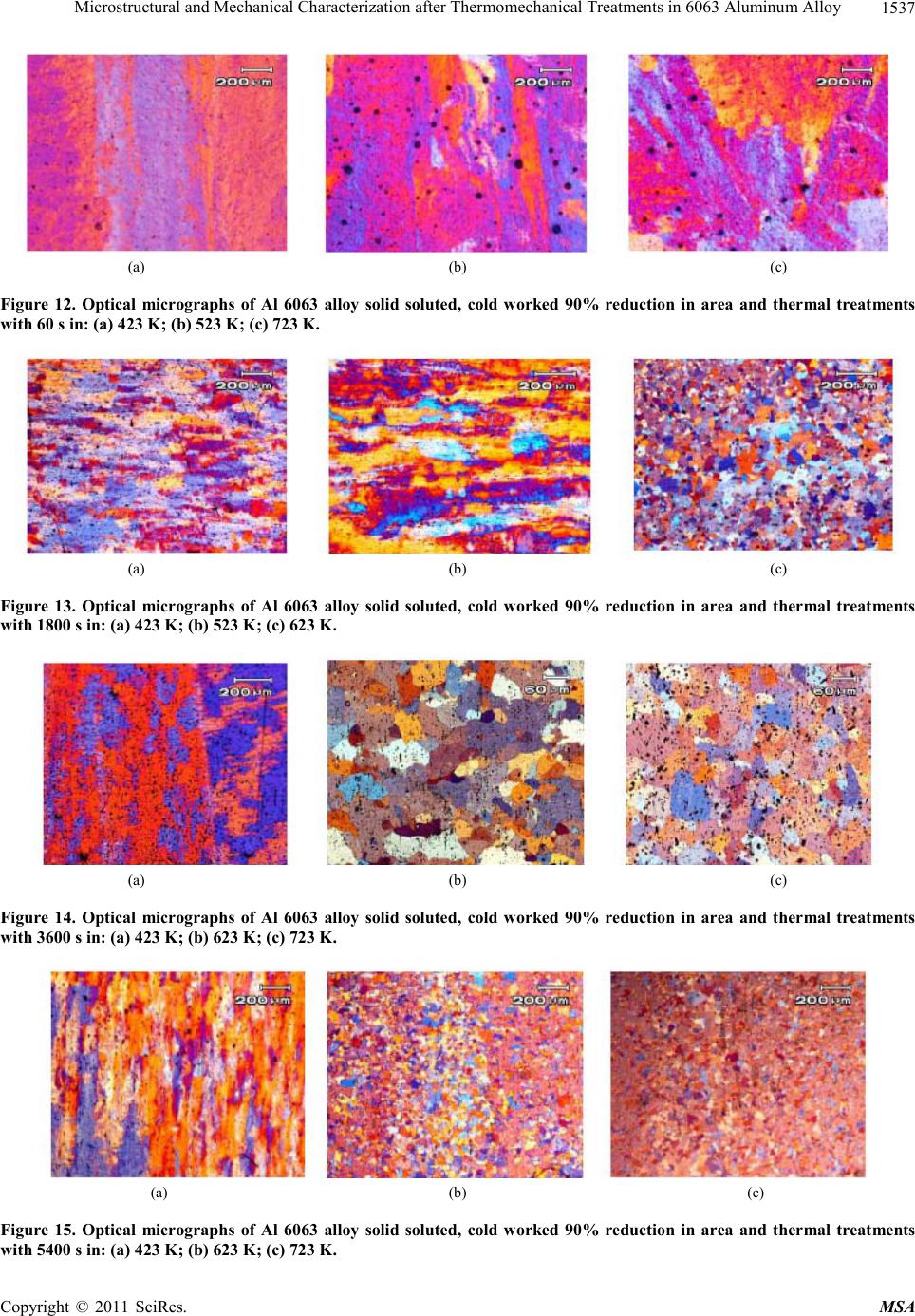 Microst ructural and Mechanical Characterizatio n after Thermomechani cal Treatments in 6063 Aluminum Alloy1537 (a) (b) (c) Figure 12. Optical micrographs of Al 6063 alloy solid soluted, cold worked 90% reduction in area and thermal treatments with 60 s in: (a) 423 K; (b) 523 K; (c) 723 K. (a) (b) (c) Figure 13. Optical micrographs of Al 6063 alloy solid soluted, cold worked 90% reduction in area and thermal treatments with 1800 s in: (a) 423 K; (b) 523 K; (c) 623 K. (a) (b) (c) Figure 14. Optical micrographs of Al 6063 alloy solid soluted, cold worked 90% reduction in area and thermal treatments with 3600 s in: (a) 423 K; (b) 623 K; (c) 723 K. (a) (b) (c) Figure 15. Optical micrographs of Al 6063 alloy solid soluted, cold worked 90% reduction in area and thermal treatments with 5400 s in: (a) 423 K; (b) 623 K; (c) 723 K. Copyright © 2011 SciRes. MSA
 Microst ructural and Mechanical Characterizatio n after Thermomechani cal Treatments in 6063 Aluminum Alloy 1538 (a) (b) Figure 16. Optical micrographs of Al 6063 alloy solid soluted, cold worked 90% reduction in area and thermal treatments with 7200 s in: (a) 623 K; (b) 723 K. Figure 17. Electron micrographs (TEM) of Al 6063 alloy, cold worked 30% of reduction in area. Presence of elon- gated (deformed) grains, dislocation tangles inside them and also small precipitates. Figure 18. Electron micrographs (TEM) of Al 6063 alloy cold worked 90% of reduction in area. Presence of disloca- tion tangles insi de the elongate d grains; there ar e also some cells in differe nt regions. Figure 19. TEM micrograph of Al 6063 alloy after reduc- tion in area of 30% e followed by ther mal treatment of 423 K for 1800 s. Presence of precipitates in grain boundary and dis location tangles i nside grain s. Figure 20. TEM micrograph of Al 6063 alloy after reduc- tion in area of 30% followed by thermal treatment of 423 K for 3600 s. Presence of subgrains, dislocations tangle and small precipitates. Copyright © 2011 SciRes. MSA
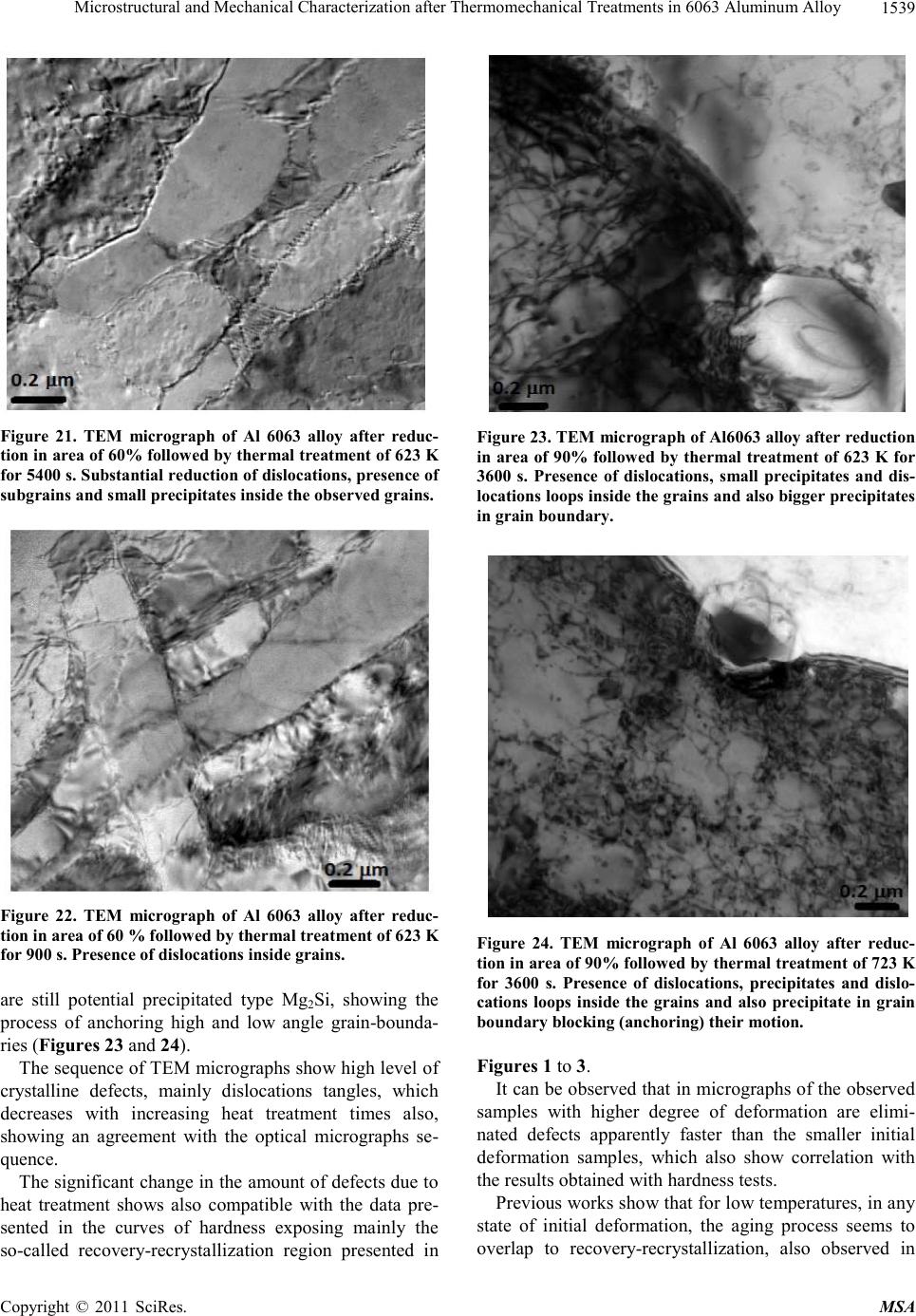 Microst ructural and Mechanical Characterizatio n after Thermomechani cal Treatments in 6063 Aluminum Alloy1539 Figure 21. TEM micrograph of Al 6063 alloy after reduc- tion in area of 60% followed by thermal treatment of 623 K for 5 400 s. Substa ntial redu cti on of di sloc atio ns, prese nce of subgrains and small precipitates insid e the observe d g rains. Figure 22. TEM micrograph of Al 6063 alloy after reduc- tion in area of 60 % followed by thermal treatment of 623 K for 900 s. Presence of dislocations inside grains. are still potential precipitated type Mg2Si, showing the process of anchoring high and low angle grain-bounda- ries (Figures 23 and 24). The sequence of TEM micrographs show high level of crystalline defects, mainly dislocations tangles, which decreases with increasing heat treatment times also, showing an agreement with the optical micrographs se- quence. The significant change in the amount of defects due to heat treatment shows also compatible with the data pre- sented in the curves of hardness exposing mainly the so-called recovery-recrystallization region presented in Figure 23. TEM micrograph of Al6063 alloy after reduction in area of 90% followed by thermal treatment of 623 K for 3600 s. Presence of dislocations, small precipitates and dis- locati o ns l oo ps ins i de the gr ai ns an d als o big g er pr e cipi t at es in gr ain boundary. Figure 24. TEM micrograph of Al 6063 alloy after reduc- tion in area of 90% followed by thermal treatment of 723 K for 3600 s. Presence of dislocations, precipitates and dislo- cations loops inside the grains and also precipitate in grain boundary blocking (anchoring) their motion. Figures 1 to 3. It can be observed that in micrographs of the observed samples with higher degree of deformation are elimi- nated defects apparently faster than the smaller initial deformation samples, which also show correlation with the results obtained with hardness tests. Previous works show that for low temperatures, in any state of initial deformation, the aging process seems to overlap to recovery-recrystallization, also observed in Copyright © 2011 SciRes. MSA
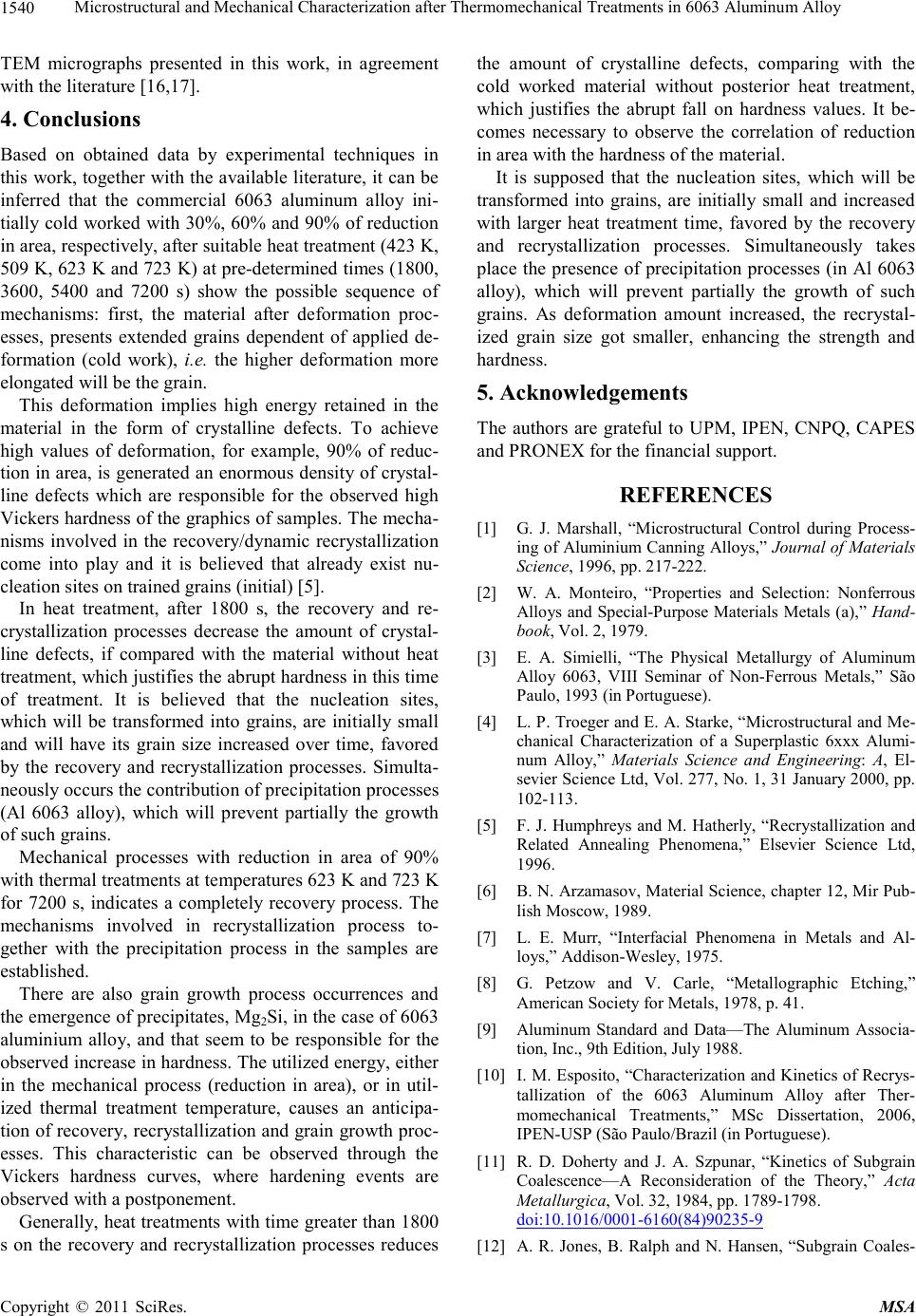 Microst ructural and Mechanical Characterizatio n after Thermomechani cal Treatments in 6063 Aluminum Alloy 1540 TEM micrographs presented in this work, in agreement with the literature [16,17]. 4. Conclusions Based on obtained data by experimental techniques in this work, together with the available literature, it can be inferred that the commercial 6063 aluminum alloy ini- tially cold worked with 30%, 60% and 90% of reduction in area, respectively, after suitable heat treatment (423 K, 509 K, 623 K and 723 K) at pre-determined times (1800, 3600, 5400 and 7200 s) show the possible sequence of mechanisms: first, the material after deformation proc- esses, presents extended grains dependent of applied de- formation (cold work), i.e. the higher deformation more elongated will be the grain. This deformation implies high energy retained in the material in the form of crystalline defects. To achieve high values of deformation, for example, 90% of reduc- tion in area, is generated an enormous density of crystal- line defects which are responsible for the observed high Vickers hardness of the graphics of samples. The mecha- nisms involved in the recovery/dynamic recrystallization come into play and it is believed that already exist nu- cleation sites on trained grains (initial) [5]. In heat treatment, after 1800 s, the recovery and re- crystallization processes decrease the amount of crystal- line defects, if compared with the material without heat treatment, which justifies the abrupt hardness in this ti me of treatment. It is believed that the nucleation sites, which will be transformed into grains, are initially small and will have its grain size increased over time, favored by the recovery and recrystallization processes. Simulta- neously occurs the contribution of precipitation processes (Al 6063 alloy), which will prevent partially the growth of such grains. Mechanical processes with reduction in area of 90% with thermal treatments at temperatures 623 K and 723 K for 7200 s, indicates a completely recovery process. The mechanisms involved in recrystallization process to- gether with the precipitation process in the samples are established. There are also grain growth process occurrences and the emergence of precipitates, Mg2Si, in the case of 6063 aluminium alloy, and that seem to be responsible for the observed increase in hardness. The utilized energy, either in the mechanical process (reduction in area), or in util- ized thermal treatment temperature, causes an anticipa- tion of recovery, recrystallization and grain growth proc- esses. This characteristic can be observed through the Vickers hardness curves, where hardening events are observed with a postponement. Generally, heat treatments with time greater than 1800 s on the recovery and recrystallization processes reduces the amount of crystalline defects, comparing with the cold worked material without posterior heat treatment, which justifies the abrupt fall on hardness values. It be- comes necessary to observe the correlation of reduction in area with the hardness of the material. It is supposed that the nucleation sites, which will be transformed into grains, are initially small and increased with larger heat treatment time, favored by the recovery and recrystallization processes. Simultaneously takes place the presence of precipitation processes (in Al 6063 alloy), which will prevent partially the growth of such grains. As deformation amount increased, the recrystal- ized grain size got smaller, enhancing the strength and hardness. 5. Acknowledgements The authors are grateful to UPM, IPEN, CNPQ, CAPES and PRONEX for the financial support. REFERENCES [1] G. J. Marshall, “Microstructural Control during Process- ing of Aluminium Canning Alloys,” Journal of Materials Science, 1996, pp. 217-222. [2] W. A. Monteiro, “Properties and Selection: Nonferrous Alloys and Special-Purpose Materials Metals (a),” Hand - book, Vol. 2, 1979. [3] E. A. Simielli, “The Physical Metallurgy of Aluminum Alloy 6063, VIII Seminar of Non-Ferrous Metals,” São Paulo, 1993 (in Portuguese). [4] L. P. Troeger and E. A. Starke, “Microstructural and Me- chanical Characterization of a Superplastic 6xxx Alumi- num Alloy,” Materials Science and Engineering: A, El- sevier Science Ltd, Vol. 277, No. 1, 31 January 2000, pp. 102-113. [5] F. J. Humphreys and M. Hatherly, “Recrystallization and Related Annealing Phenomena,” Elsevier Science Ltd, 1996. [6] B. N. Arzamasov, Material S cience, chapter 12 , Mir Pub- lish Moscow, 1989. [7] L. E. Murr, “Interfacial Phenomena in Metals and Al- loys,” Addison-Wesley, 1975. [8] G. Petzow and V. Carle, “Metallographic Etching,” American Society for Metal s, 1978, p. 41. [9] Aluminum Standard and Data—The Aluminum Associa- tion, Inc., 9th Edition, July 1988. [10] I. M. Espo sito, “Characterizatio n and Kinetics of Recr ys- tallization of the 6063 Aluminum Alloy after Ther- momechanical Treatments,” MSc Dissertation, 2006, IPEN-USP (São Paulo/Brazil (in Portuguese). [11] R. D. Doherty and J. A. Szpunar, “Kinetics of Subgrain Coalescence—A Reconsideration of the Theory,” Acta Metallurgica, Vol. 32, 19 84 , pp. 178 9 -1798. do i:10.1016/ 0001-6160(84)90 235-9 [12] A. R. Jones, B. Ralph and N. Hansen, “Subgrain Coales- Copyright © 2011 SciRes. MSA
 Microst ructural and Mechanical Characterizatio n after Thermomechani cal Treatments in 6063 Aluminum Alloy Copyright © 2011 SciRes. MSA 1541 cence and the Nucleation of Recrystallization at Grain Boundaries in Aluminum,” Proceedings of the Royal So- ciety of London, Vol. 368A, 19 76, pp. 345-357. [13] A. R. Jones, B. Ralph and N. Hansen, “Nucleation of Recrystallization in Aluminium Containing Dispersion of Alumina,” Metal Science, Vol. 13, 1979, pp. 149-154. [14] D. A. Hughes and N. Hansen, “High Angle Boundaries and Orientation Distributions at Large Strains,” Scripta Metallurgica et Materialia, Vol. 33, N o. 2, 19 95. doi:10.1016/0956-716X(95)00143-J [15] K. Uttarask, S. Ngernbamrung and N. Sirikulrat, “Micro- structures and Mechanical Properties of Heat Treated Aluminium Alloy 6063,” 33nd Congress on Science and Technology of Tha iland, 18-20 October, 2007. [16] E. Tan and B. Ögel, “Influence o f Heat Treat ment on the Mechanical Properties AA6066 Alloy,” Turkish Journal of Engineering and Environmental Sciences, Vol. 31, 2007, pp 53- 6 0. [17] G. Al-Marahleh, “Effect of Heat Treatment Parameters on Distribution and Volume Fraction of Mg2Si in the Struc- tural Al 6063 Alloy,” American Journal of Applied Sci- ences, Vol. 3, No. 5, 2006, pp. 1819-1823. do i:10.3844/ajassp.2006.1819 .1823
|