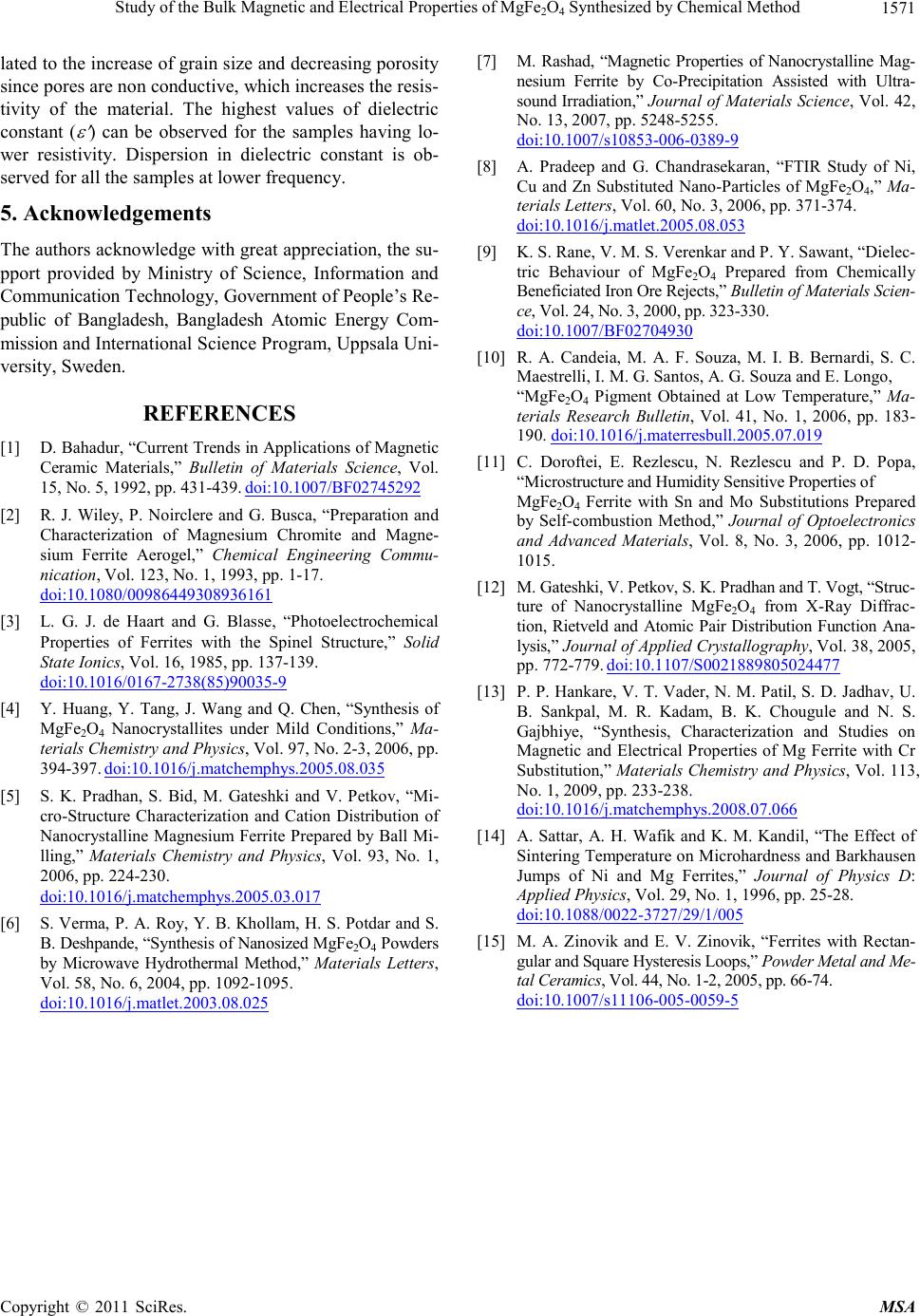
Study of the Bulk Magnetic and Electrical Properties of MgFe2O4 Synthesized by Chemical Method
Copyright © 2011 SciRes. MSA
1571
on, the su-
of Science, Informatio
Government of People’s Re-
Current Trends in Applications of Magnetic
Ceramic Materials Science, Vol
15, No. 5, 19907/BF02745
lated to the increase of grain size and decreasing porosity
since pores are non conductive, which increases the resis-
tivity of the material. The highest values of dielectric
constant (
) can be observed for the samples having lo-
wer resistivity. Dispersion in dielectric constant is ob-
served for all the samples at lower frequency.
5. Acknowledgements
The authors acknowledge with great appreciati
pport provided by Ministry
Communication Technology,
n and
public of Bangladesh, Bangladesh Atomic Energy Com-
mission and International Science Program, Uppsala Uni-
versity, Sweden.
REFERENCES
[1] D. Bahadur, “
. rials,” Bulletin of Mate
2, pp. 431-439. doi:10.10 292
[2] R. J. Wiley, P. Noirclere and G. Busca, “Preparation and
Characterization of Magnesium Chromite and Magne-
sium Ferrite Aerogel,” Chemical Engineering Commu-
nication, Vol. 123, No. 1, 1993, pp. 1-17.
doi:10.1080/00986449308936161
[3] L. G. J. de Haart and G. Blasse, “Photoelectrochemical
Properties of Ferrites with the Spinel St
State Ionics
ructure,” Solid
-139.
, Vol. 16, 1985, pp. 137
doi:10.1016/0167-2738(85)90035-9
[4] Y. Huang, Y. Tang, J. Wang and Q. Chen, “Synthesis of
MgFe2O4 Nanocrystallites under Mild Conditions,” Ma-
terials Chemistry and Physics, Vol. 97, No. 2-3, 2006, pp.
394-397. doi:10.1016/j.matchemphys.2005.08.035
[5] S. K. Pradhan, S. Bid, M. Gateshki and V. Petkov, “Mi-
cro-Structure Characterization and Cation Distribution of
Nanocrystalline Magnesium Ferrite Prepared by Ball Mi-
lling,” Materials Chemistry and Physics, Vol. 93, No. 1,
2006, pp. 224-230.
doi:10.1016/j.matchemphys.2005.03.017
[6] S. Verma, P. A. Roy, Y. B. Khollam, H. S. Potdar and S.
B. Deshpande, “Synthe
by Microwave Hydrothermal Method,” M
sis of Nanosized MgFe2O4 Powders
aterials Le
tters,
Vol. 58, No. 6, 2004, pp. 1092-1095.
doi:10.1016/j.matlet.2003.08.025
[7] M. Rashad, “Magnetic Properties of Nanocrystalline Mag-
nesium Ferrite by Co-Precipitation Assisted with Ultra-
sound Irradiation,” Journal of Materials Science, Vol. 42,
No. 13, 2007, pp. 5248-5255.
doi:10.1007/s10853-006-0389-9
[8] A. Pradeep and G. Chandrasekaran, “FTIR Study of Ni,
Cu and Zn Substituted Nano-Particles of MgFe2O4,” Ma-
terials Letters, Vol. 60, No. 3, 2006, pp. 371-374.
doi:10.1016/j.matlet.2005.08.053
[9] K. S. Rane, V. M. S. Verenkar and P. Y. Sawant, “Dielec-
tric Behaviour of MgFe2O4 Prepared from Chemically
Beneficiated Iron Ore Rejects,” Bulletin of Materials Scien-
ce, Vol. 24, No. 3, 2000, pp. 323-330.
doi:10.1007/BF02704930
[10] R. A. Candeia, M. A. F. Souza, M. I. B. Bernardi, S. C.
Maestrelli, I. M. G. Santos, A. G. Souza and E. Longo,
“MgFe2O4 Pigment Obtained at Low Temperature,” Ma-
terials Research Bulletin, Vol. 41, No. 1, 2006, pp. 183-
190. doi:10.1016/j.materresbull.2005.07.019
[11] C. Doroftei, E. Rezlescu, N. Rezlescu and P. D. Popa,
hki, V. Petkov, S. K. Pradhan and T. Vogt, “Struc-
“Microstructure and Humidity Sensitive Properties of
MgFe2O4 Ferrite with Sn and Mo Substitutions Prepared
by Self-combustion Method,” Journal of Optoelectronics
and Advanced Materials, Vol. 8, No. 3, 2006, pp. 1012-
1015.
[12] M. Gates
ture of Nanocrystalline MgFe2O4 from X-Ray Diffrac-
tion, Rietveld and Atomic Pair Distribution Function Ana-
lysis,” Journal of Applied Crystallography, Vol. 38, 2005,
pp. 772-779. doi:10.1107/S0021889805024477
[13] P. P. Hankare, V. T. Vader, N. M. Patil, S. D. Jadhav, U.
B. Sankpal, M. R. Kadam, B. K. Chougule and N. S.
Gajbhiye, “Synthesis, Characterization and Studies on
Magnetic and Electrical Properties of Mg Ferrite with Cr
Substitution,” Materials Chemistry and Physics, Vol. 113,
No. 1, 2009, pp. 233-238.
doi:10.1016/j.matchemphys.2008.07.066
[14] A. Sattar, A. H. Wafik and K. M. Kandil, “The Effect of
Sintering Temperature on Microhardness and Barkhausen
Jumps of Ni and Mg Ferrites,” Journal of Physics D:
Applied Physics, Vol. 29, No. 1, 1996, pp. 25-28.
doi:10.1088/0022-3727/29/1/005
[15] M. A. Zinovik and E. V. Zinovik, “Ferrites with Rectan-
gular and Square Hysteresis Loops,” Powder Metal and Me-
tal Ceramics, Vol. 44, No. 1-2, 2005, pp. 66-74.
doi:10.1007/s11106-005-0059-5