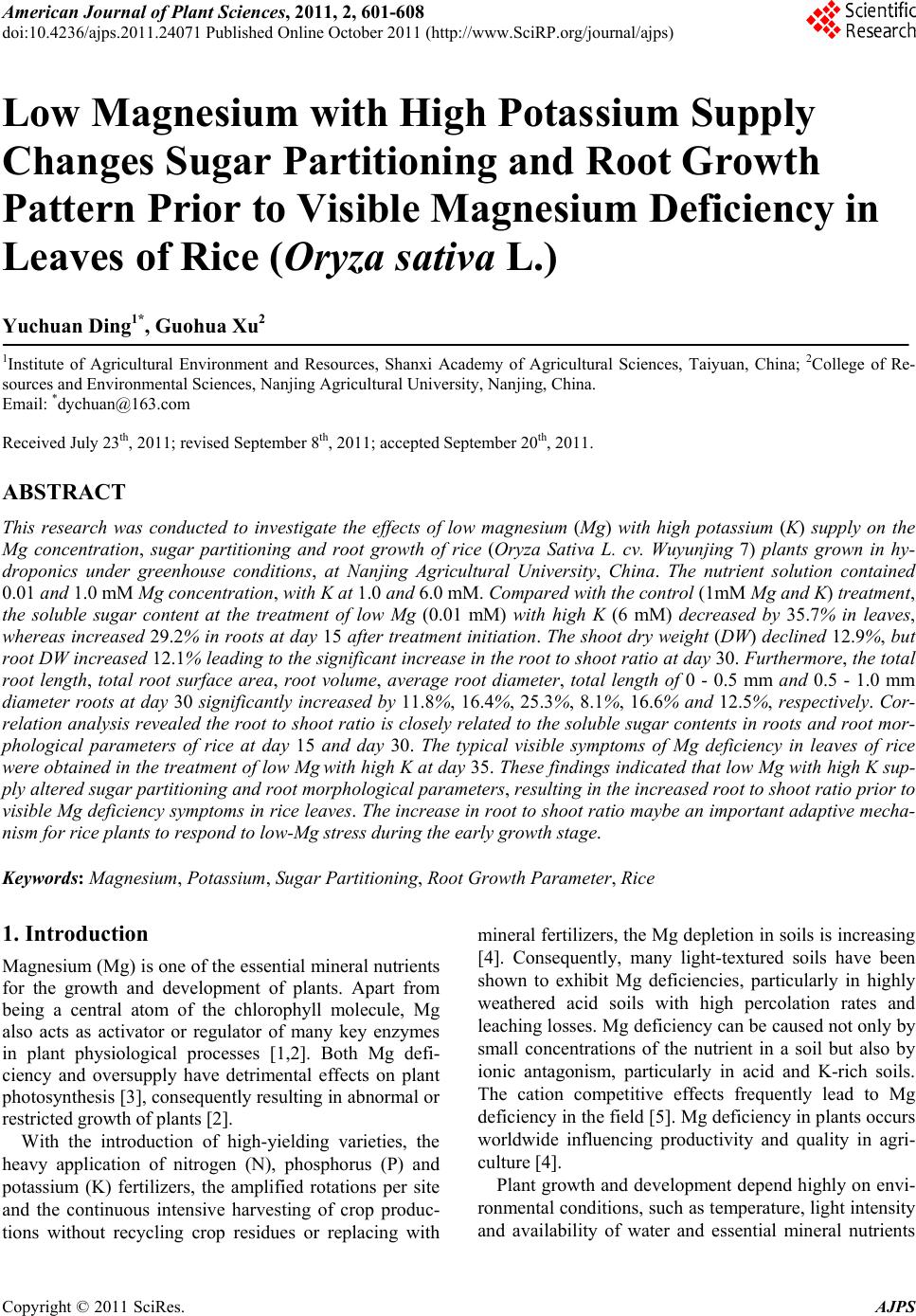 American Journal of Plant Sciences, 2011, 2, 601-608 doi:10.4236/ajps.2011.24071 Published Online October 2011 (http://www.SciRP.org/journal/ajps) Copyright © 2011 SciRes. AJPS 601 Low Magnesium with High Potassium Supply Changes Sugar Partitioning and Root Growth Pattern Prior to Visible Magnesium Deficiency in Leaves of Rice (Oryza sativa L.) Yuchuan Ding1*, Guohua Xu2 1Institute of Agricultural Environment and Resources, Shanxi Academy of Agricultural Sciences, Taiyuan, China; 2College of Re- sources and Environmental Sciences, Nanjing Agricultural University, Nanjing, China. Email: *dychuan@163.com Received July 23th, 2011; revised September 8th, 2011; accepted September 20th, 2011. ABSTRACT This research was conducted to investigate the effects of low magnesium (Mg) with high potassium (K) supply on the Mg concentration, sugar partitioning and root growth of rice (Oryza Sativa L. cv. Wuyunjing 7) plants grown in hy- droponics under greenhouse conditions, at Nanjing Agricultural University, China. The nutrient solution contained 0.01 and 1.0 mM Mg concentration, with K at 1.0 and 6.0 mM. Compared with the co n trol (1mM Mg and K) treatment, the soluble sugar content at the treatment of low Mg (0.01 mM) with high K (6 mM) decreased by 35.7% in leaves, whereas increased 29.2% in roots at day 15 after treatment initiation. The shoot dry weight (DW) declined 12.9%, but root DW increased 12.1% lead ing to the significan t increase in the ro ot to shoot ratio at da y 30. Furthermore, the total root length, total root surface area, root volume, average root diameter, total length of 0 - 0.5 mm and 0.5 - 1.0 mm diameter roots at day 30 significantly increased by 11.8%, 16.4%, 25.3%, 8.1%, 16.6% and 12.5%, respectively. Cor- relation analysis revealed the root to shoot ratio is closely related to the soluble sugar contents in roots and root mor- phological parameters of rice at day 15 and day 30. The typical visible symptoms of Mg deficiency in leaves of rice were obtained in the trea tment of low Mg with high K at day 35. These findings indicated that low Mg with high K sup- ply altered sugar pa rtition ing and roo t morpho logica l pa rameters, resultin g in th e in crea sed root to sho o t ra tio pr io r to visible Mg deficiency symptoms in rice leaves. The increase in root to shoot ratio maybe an important adaptive mecha- nism for rice plants to respond to low-Mg stress during the early growth stag e. Keywords: Magnesium, Potassium, Sugar Partitioning, Root Growth Parameter, Rice 1. Introduction Magnesium (Mg) is one of the essential mineral nutrients for the growth and development of plants. Apart from being a central atom of the chlorophyll molecule, Mg also acts as activator or regulator of many key enzymes in plant physiological processes [1,2]. Both Mg defi- ciency and oversupply have detrimental effects on plant photosynthesis [3], consequently resulting in abnormal or restricted growth of plants [2]. With the introduction of high-yielding varieties, the heavy application of nitrogen (N), phosphorus (P) and potassium (K) fertilizers, the amplified rotations per site and the continuous intensive harvesting of crop produc- tions without recycling crop residues or replacing with mineral fertilizers, the Mg depletion in soils is increasing [4]. Consequently, many light-textured soils have been shown to exhibit Mg deficiencies, particularly in highly weathered acid soils with high percolation rates and leaching losses. Mg deficiency can be caused not only by small concentrations of the nutrient in a soil but also by ionic antagonism, particularly in acid and K-rich soils. The cation competitive effects frequently lead to Mg deficiency in the field [5]. Mg deficiency in plants occurs worldwide influencing productivity and quality in agri- culture [4]. Plant growth and development depend highly on envi- ronmental conditions, such as temperature, light intensity and availability of water and essential mineral nutrients
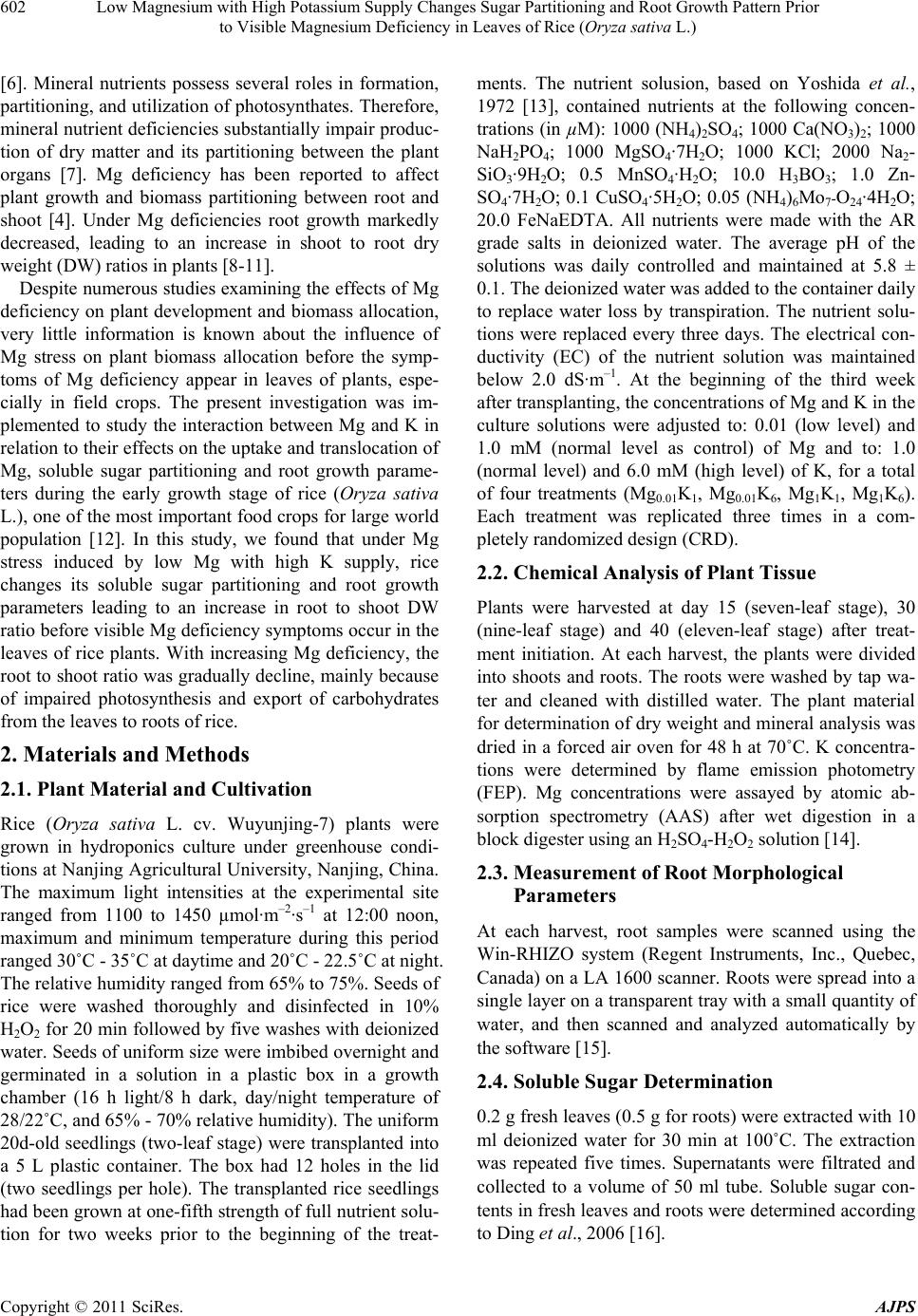 Low Magnesium with High Potassium Supply Changes Sugar Partitioning and Root Growth Pattern Prior 602 to Visible Magnesium Deficiency in Leaves of Rice (Oryza sativa L.) [6]. Mineral nutrients possess several roles in formation, partitioning, and utilization of photosynthates. Therefore, mineral nutrient deficiencies substantially impair produc- tion of dry matter and its partitioning between the plant organs [7]. Mg deficiency has been reported to affect plant growth and biomass partitioning between root and shoot [4]. Under Mg deficiencies root growth markedly decreased, leading to an increase in shoot to root dry weight (DW) ratios in plants [8-11]. Despite numerous studies examining the effects of Mg deficiency on plant development and biomass allocation, very little information is known about the influence of Mg stress on plant biomass allocation before the symp- toms of Mg deficiency appear in leaves of plants, espe- cially in field crops. The present investigation was im- plemented to study the interaction between Mg and K in relation to their effects on the uptake and translocation of Mg, soluble sugar partitioning and root growth parame- ters during the early growth stage of rice (Oryza sativa L.), one of the most important food crops for large world population [12]. In this study, we found that under Mg stress induced by low Mg with high K supply, rice changes its soluble sugar partitioning and root growth parameters leading to an increase in root to shoot DW ratio before visible Mg deficiency symptoms occur in the leaves of rice plants. With increasing Mg deficiency, the root to shoot ratio was gradually decline, mainly because of impaired photosynthesis and export of carbohydrates from the leaves to roots of rice. 2. Materials and Methods 2.1. Plant Material and Cultivation Rice (Oryza sativa L. cv. Wuyunjing-7) plants were grown in hydroponics culture under greenhouse condi- tions at Nanjing Agricultural University, Nanjing, China. The maximum light intensities at the experimental site ranged from 1100 to 1450 µmol·m–2·s–1 at 12:00 noon, maximum and minimum temperature during this period ranged 30˚C - 35˚C at daytime and 20˚C - 22.5˚C at night. The relative humidity ranged from 65% to 75%. Seeds of rice were washed thoroughly and disinfected in 10% H2O2 for 20 min followed by five washes with deionized water. Seeds of uniform size were imbibed overnight and germinated in a solution in a plastic box in a growth chamber (16 h light/8 h dark, day/night temperature of 28/22˚C, and 65% - 70% relative humidity). The uniform 20d-old seedlings (two-leaf stage) were transplanted into a 5 L plastic container. The box had 12 holes in the lid (two seedlings per hole). The transplanted rice seedlings had been grown at one-fifth strength of full nutrient solu- tion for two weeks prior to the beginning of the treat- ments. The nutrient solusion, based on Yoshida et al., 1972 [13], contained nutrients at the following concen- trations (in µM): 1000 (NH4)2SO4; 1000 Ca(NO3)2; 1000 NaH2PO4; 1000 MgSO4·7H2O; 1000 KCl; 2000 Na2- SiO3·9H2O; 0.5 MnSO4·H2O; 10.0 H3BO3; 1.0 Zn- SO4·7H2O; 0.1 CuSO4·5H2O; 0.05 (NH4)6Mo7-O24·4H2O; 20.0 FeNaEDTA. All nutrients were made with the AR grade salts in deionized water. The average pH of the solutions was daily controlled and maintained at 5.8 ± 0.1. The deionized water was added to the container daily to replace water loss by transpiration. The nutrient solu- tions were replaced every three days. The electrical con- ductivity (EC) of the nutrient solution was maintained below 2.0 dS·m–1. At the beginning of the third week after transplanting, the concentrations of Mg and K in the culture solutions were adjusted to: 0.01 (low level) and 1.0 mM (normal level as control) of Mg and to: 1.0 (normal level) and 6.0 mM (high level) of K, for a total of four treatments (Mg0.01K1, Mg0.01K6, Mg1K1, Mg1K6). Each treatment was replicated three times in a com- pletely randomized design (CRD). 2.2. Chemical Analysis of Plant Tissue Plants were harvested at day 15 (seven-leaf stage), 30 (nine-leaf stage) and 40 (eleven-leaf stage) after treat- ment initiation. At each harvest, the plants were divided into shoots and roots. The roots were washed by tap wa- ter and cleaned with distilled water. The plant material for determination of dry weight and mineral analysis was dried in a forced air oven for 48 h at 70˚C. K concentra- tions were determined by flame emission photometry (FEP). Mg concentrations were assayed by atomic ab- sorption spectrometry (AAS) after wet digestion in a block digester using an H2SO4-H2O2 solution [14]. 2.3. Measurement of Root Morphological Parameters At each harvest, root samples were scanned using the Win-RHIZO system (Regent Instruments, Inc., Quebec, Canada) on a LA 1600 scanner. Roots were spread into a single layer on a transparent tray with a small quantity of water, and then scanned and analyzed automatically by the software [15]. 2.4. Soluble Sugar Determination 0.2 g fresh leaves (0.5 g for roots) were extracted with 10 ml deionized water for 30 min at 100˚C. The extraction was repeated five times. Supernatants were filtrated and collected to a volume of 50 ml tube. Soluble sugar con- tents in fresh leaves and roots were determined according to Ding et al., 2006 [16]. Copyright © 2011 SciRes. AJPS
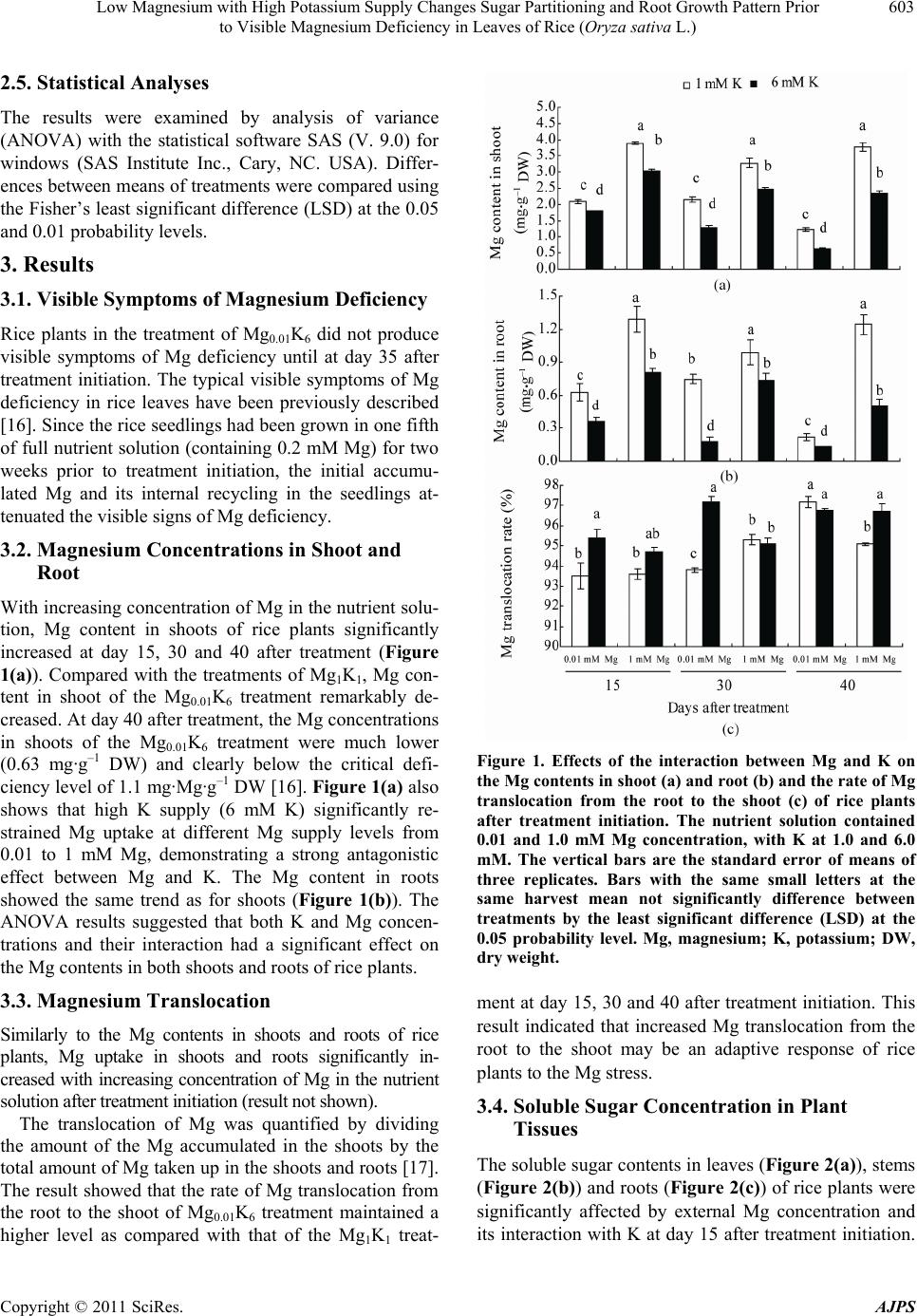 Low Magnesium with High Potassium Supply Changes Sugar Partitioning and Root Growth Pattern Prior 603 to Visible Magnesium Deficiency in Leaves of Rice (Oryza sativa L.) 2.5. Statistical Analyses The results were examined by analysis of variance (ANOVA) with the statistical software SAS (V. 9.0) for windows (SAS Institute Inc., Cary, NC. USA). Differ- ences between means of treatments were compared using the Fisher’s least significant difference (LSD) at the 0.05 and 0.01 probability levels. 3. Results 3.1. Visible Symptoms of Magnesium Deficiency Rice plants in the treatment of Mg0.01K6 did not produce visible symptoms of Mg deficiency until at day 35 after treatment initiation. The typical visible symptoms of Mg deficiency in rice leaves have been previously described [16]. Since the rice seedlings had been grown in one fifth of full nutrient solution (containing 0.2 mM Mg) for two weeks prior to treatment initiation, the initial accumu- lated Mg and its internal recycling in the seedlings at- tenuated the visible signs of Mg deficiency. 3.2. Magnesium Concentrations in Shoot and Root With increasing concentration of Mg in the nutrient solu- tion, Mg content in shoots of rice plants significantly increased at day 15, 30 and 40 after treatment (Figure 1(a)). Compared with the treatments of Mg1K1, Mg con- tent in shoot of the Mg0.01K6 treatment remarkably de- creased. At day 40 after treatment, the Mg concentrations in shoots of the Mg0.01K6 treatment were much lower (0.63 mg·g–1 DW) and clearly below the critical defi- ciency level of 1.1 mg·Mg·g–1 DW [16]. Figure 1(a) also shows that high K supply (6 mM K) significantly re- strained Mg uptake at different Mg supply levels from 0.01 to 1 mM Mg, demonstrating a strong antagonistic effect between Mg and K. The Mg content in roots showed the same trend as for shoots (Figure 1(b)). The ANOVA results suggested that both K and Mg concen- trations and their interaction had a significant effect on the Mg contents in both shoots and roots of rice plants. 3.3. Magnesium Translocation Similarly to the Mg contents in shoots and roots of rice plants, Mg uptake in shoots and roots significantly in- creased with increasing concentration of Mg in the nutrient solution after treatment initiation (result not shown). The translocation of Mg was quantified by dividing the amount of the Mg accumulated in the shoots by the total amount of Mg taken up in the shoots and roots [17]. The result showed that the rate of Mg translocation from the root to the shoot of Mg0.01K6 treatment maintained a higher level as compared with that of the Mg1K1 treat- Figure 1. Effects of the interaction between Mg and K on the Mg contents in shoot (a) and root (b) and the rate of Mg translocation from the root to the shoot (c) of rice plants after treatment initiation. The nutrient solution contained 0.01 and 1.0 mM Mg concentration, with K at 1.0 and 6.0 mM. The vertical bars are the standard error of means of three replicates. Bars with the same small letters at the same harvest mean not significantly difference between treatments by the least significant difference (LSD) at the 0.05 probability level. Mg, magnesium; K, potassium; DW, dry weight. ment at day 15, 30 and 40 after treatment initiation. This result indicated that increased Mg translocation from the root to the shoot may be an adaptive response of rice plants to the Mg stress. 3.4. Soluble Sugar Concentration in Plant Tissues The soluble sugar contents in leaves (Figure 2(a)), stems (Figure 2(b)) and roots (Figure 2(c)) of rice plants were significantly affected by external Mg concentration and its interaction with K at day 15 after treatment initiation. Copyright © 2011 SciRes. AJPS
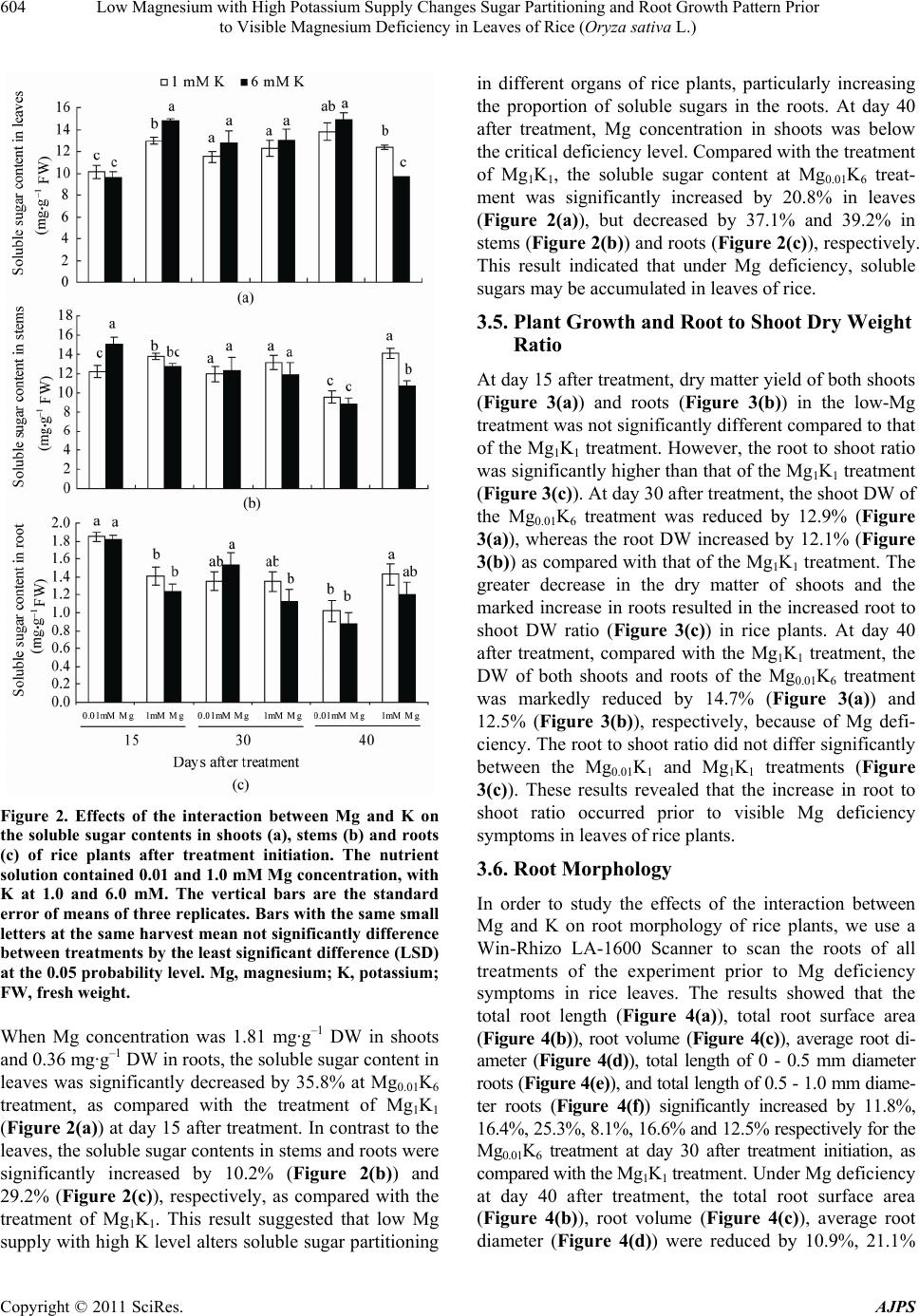 Low Magnesium with High Potassium Supply Changes Sugar Partitioning and Root Growth Pattern Prior 604 to Visible Magnesium Deficiency in Leaves of Rice (Oryza sativa L.) Figure 2. Effects of the interaction between Mg and K on the soluble sugar contents in shoots (a), stems (b) and roots (c) of rice plants after treatment initiation. The nutrient solution contained 0.01 and 1.0 mM Mg concentration, with K at 1.0 and 6.0 mM. The vertical bars are the standard error of means of three replicates. Bars with the same small letters at the same harvest mean not significantly difference between treatments by the least significant difference (LSD) at the 0.05 probability level. Mg, magnesium; K, potassium; FW, fresh we ight. When Mg concentration was 1.81 mg·g–1 DW in shoots and 0.36 mg·g–1 DW in roots, the soluble sugar content in leaves was significantly decreased by 35.8% at Mg0.01K6 treatment, as compared with the treatment of Mg1K1 (Figure 2(a)) at day 15 after treatment. In contrast to the leaves, the soluble sugar contents in stems and roots were significantly increased by 10.2% (Figure 2(b)) and 29.2% (Figure 2(c)), respectively, as compared with the treatment of Mg1K1. This result suggested that low Mg supply with high K level alters soluble sugar partitioning in different organs of rice plants, particularly increasing the proportion of soluble sugars in the roots. At day 40 after treatment, Mg concentration in shoots was below the critical deficiency level. Compared with the treatment of Mg1K1, the soluble sugar content at Mg0.01K6 treat- ment was significantly increased by 20.8% in leaves (Figure 2(a)), but decreased by 37.1% and 39.2% in stems (Figure 2(b)) and roots (Figure 2(c) ), respectively. This result indicated that under Mg deficiency, soluble sugars may be accumulated in leaves of rice. 3.5. Plant Growth and Root to Shoot Dry Weight Ratio At day 15 after treatment, dry matter yield of both shoots (Figure 3(a)) and roots (Figure 3(b)) in the low-Mg treatment was not significantly different compared to that of the Mg1K1 treatment. However, the root to shoot ratio was significantly higher than that of the Mg1K1 treatment (Figure 3(c)). At day 30 after treatment, the shoot DW of the Mg0.01K6 treatment was reduced by 12.9% (Figure 3(a)), whereas the root DW increased by 12.1% (Figure 3(b)) as compared with that of the Mg1K1 treatment. The greater decrease in the dry matter of shoots and the marked increase in roots resulted in the increased root to shoot DW ratio (Figure 3(c)) in rice plants. At day 40 after treatment, compared with the Mg1K1 treatment, the DW of both shoots and roots of the Mg0.01K6 treatment was markedly reduced by 14.7% (Figure 3(a)) and 12.5% (Figure 3(b)), respectively, because of Mg defi- ciency. The root to shoot ratio did not differ significantly between the Mg0.01K1 and Mg1K1 treatments (Figure 3(c)). These results revealed that the increase in root to shoot ratio occurred prior to visible Mg deficiency symptoms in leaves of rice plants. 3.6. Root Morphology In order to study the effects of the interaction between Mg and K on root morphology of rice plants, we use a Win-Rhizo LA-1600 Scanner to scan the roots of all treatments of the experiment prior to Mg deficiency symptoms in rice leaves. The results showed that the total root length (Figure 4(a)), total root surface area (Figure 4(b)), root volume (Figure 4(c)), average root di- ameter (Figure 4(d)), total length of 0 - 0.5 mm diameter roots (Figure 4(e)), and total length of 0.5 - 1.0 mm diame- ter roots (Figure 4(f)) significantly increased by 11.8%, 16.4%, 25.3%, 8.1%, 16.6% and 12.5% respectively for the Mg0.01K6 treatment at day 30 after treatment initiation, as compared with the Mg1K1 treatment. Under Mg deficiency at day 40 after treatment, the total root surface area (Figure 4(b)), root volume (Figure 4(c)), average root diameter (Figure 4(d)) were reduced by 10.9%, 21.1% Copyright © 2011 SciRes. AJPS
 Low Magnesium with High Potassium Supply Changes Sugar Partitioning and Root Growth Pattern Prior 605 to Visible Magnesium Deficiency in Leaves of Rice (Oryza sativa L.) Figure 3. Effects of the interaction between Mg and K on dry matter yield of shoot (a) and root (b), and root to shoot DW ratio (c) of rice plants after treatment initiation. The nutrient solution contained 0.01 - 1.0 mM Mg concentra- tion, with K at 1.0 - 6.0 mM. The vertical bars are the stan- dard error of means of three replicates. Bars with the same small letters at the same harvest mean not significantly dif- ference between treatments by the least significant differ- ence (LSD) at the 0.05 probability level. Mg, magnesium; K, potassium; DW, dry weight. and 11.4% respectively, except the total length of 0 - 0.5 mm diameter roots (Figure 4(e)), and total length of 0.5 - 1.0 mm diameter roots (Figure 4(f)) were increased by 13.9% and 5.0%, respectively. 3.7. Correlation Analysis Regression analysis was performed to examine relation- ships between the soluble sugar contents in roots, root to shoot DW ratio and root morphological parameters of rice plants. Table 1 shows that the soluble sugar contents in leaves at day 15 after treatment initiation had a highly posi- tive correlation with the total root length, total root surface area, root volume, number of root forks, total length of 0 - 0.5 mm and 0.5 - 1.0 mm diameter roots and the root to shoot ratio of rice plants at day 30 after treatment initia- tion. Moreover, these root morphological parameters had a highly positive correlation with the root to shoot ratio at day 30 after treatment initiation. These results indicate that the root to shoot ratio is closely related to the soluble sugar contents in roots and root morphological parame- ters of rice plants. 4. Discussion In almost all higher plants, the principle end products of leaf photosynthates are sucrose and starch. However, partitioning of sucrose and starch and their effect on dry matter distribution is influenced by several environ- mental factors, such as low temperature, drought and essential mineral nutrients [18,19]. Mineral nutritional status of plants has a considerable impact on partitioning of carbohydrates and dry matter between shoots and roots [1,7,20,21]. It has long been known that deficien- cies of essential macronutrients (N, P, K and Mg) result in an accumulation of carbohydrates in leaves and roots, and modify the root to shoot biomass ratio [6]. Mg defi- ciency leads to the accumulation of sugars and starch in young source leaves [8,16,22-25], but they rarely in- crease their root growth because of impaired export of carbohydrates from source to sink sites [6]. In the present study, Mg nutritional status had a stronger effect on both root and shoot growth and dry matter distribution of rice plants. At day 30 after treatment initiation, shoot growth for the Mg0.01K6 treatment was severely affected by low Mg stress because the Mg concentration in shoots was 1.27 mg·g–1 DW (Figure 1(a)), which was near the criti- cal deficiency level of 1.1 mg Mg·g–1 DW [16]. However, the root growth significantly increased (Figure 3(b)) indicating that a considerable proportion of dry matter was partitioned to the roots, leading to an increase in root to shoot DW ratio before Mg deficiency symptoms were visible in leaves of rice plants (Figure 3(c)). This finding was consistent with that of Hermans et al. [6] who ob- tained an increase in the root to shoot ratio in Arabidoop- sis thaliana plants. We suggest that the increase in root to shoot ratio may be closely associated with the concentra- tions and distribution of soluble sugars in different tis- sues of plants because there is a close relationship be- tween shoot to root DW ratios and relative distribution of total carbohydrates in shoots and roots [23]. The increase of root to shoot ratio may be an important adaptive mechanism for rice plants to low-Mg stress before visible Mg deficiency signs in the leaves. Previous studies sug- gest that under Mg deficiencies root growth markedly ecreases, leading to an increase in shoot to root DW d Copyright © 2011 SciRes. AJPS
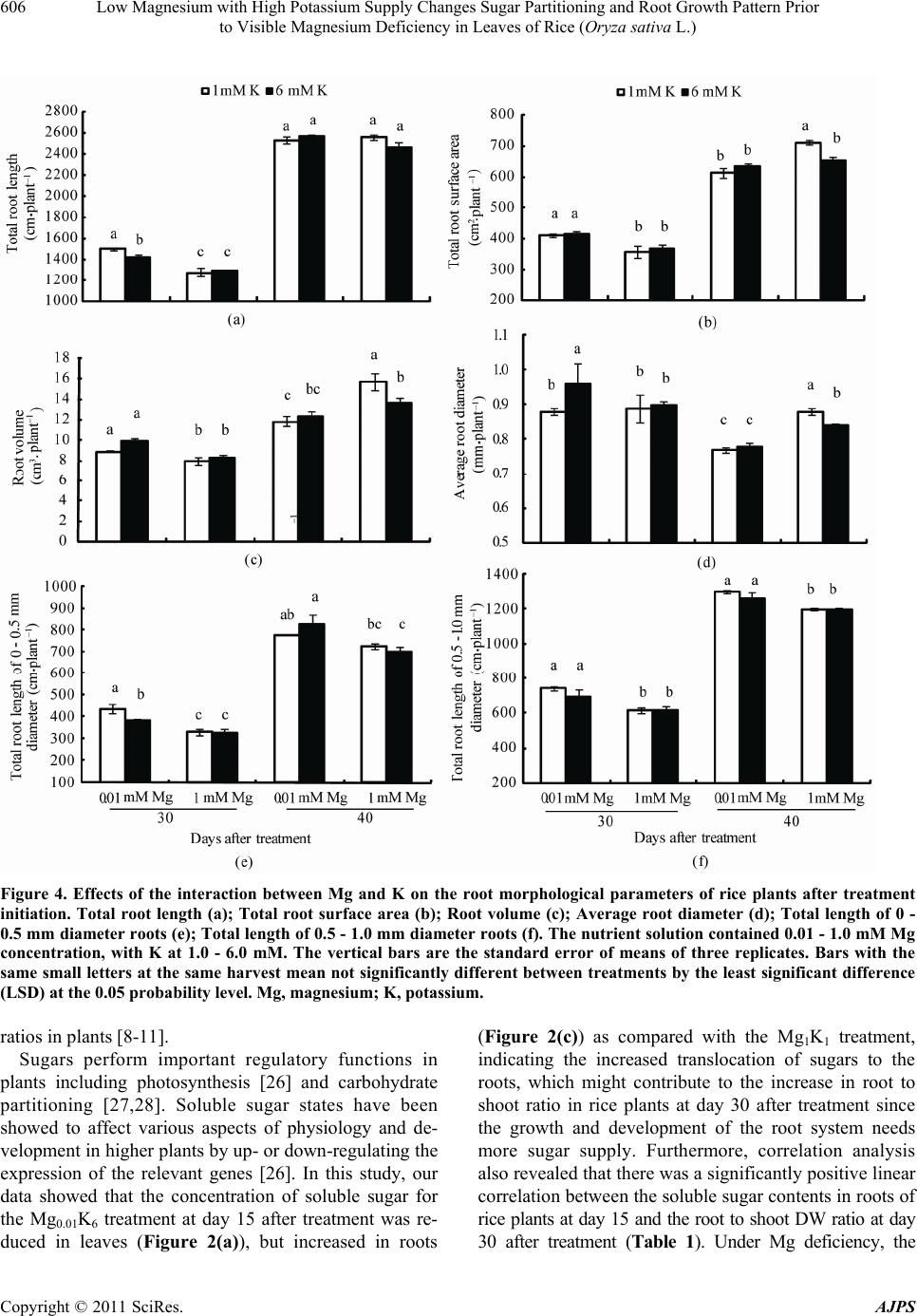 Low Magnesium with High Potassium Supply Changes Sugar Partitioning and Root Growth Pattern Prior to Visible Magnesium Deficiency in Leaves of Rice (Oryza sativa L.) Copyright © 2011 SciRes. AJPS 606 Figure 4. Effects of the interaction between Mg and K on the root morphological parameters of rice plants after treatment initiation. Total root length (a); Total root surface area (b); Root volume (c); Average root diameter (d); Total length of 0 - 0.5 mm diameter roots (e); Total length of 0.5 - 1.0 mm diameter roots (f). The nutrient solution contained 0.01 - 1.0 mM Mg concentration, with K at 1.0 - 6.0 mM. The vertical bars are the standard error of means of three replicates. Bars with the same small letters at the same harvest mean not significantly different between treatments by the least significant difference (LSD) at the 0.05 probability level. Mg, magnesium; K, potassium. ratios in plants [8-11]. Sugars perform important regulatory functions in plants including photosynthesis [26] and carbohydrate partitioning [27,28]. Soluble sugar states have been showed to affect various aspects of physiology and de- velopment in higher plants by up- or down-regulating the expression of the relevant genes [26]. In this study, our data showed that the concentration of soluble sugar for the Mg0.01K6 treatment at day 15 after treatment was re- duced in leaves (Figure 2(a)), but increased in roots (Figure 2(c)) as compared with the Mg1K1 treatment, indicating the increased translocation of sugars to the roots, which might contribute to the increase in root to shoot ratio in rice plants at day 30 after treatment since the growth and development of the root system needs more sugar supply. Furthermore, correlation analysis also revealed that there was a significantly positive linear correlation between the soluble sugar contents in roots of rice plants at day 15 and the root to shoot DW ratio at day 0 after treatment (Table 1). Under Mg deficiency, the 3
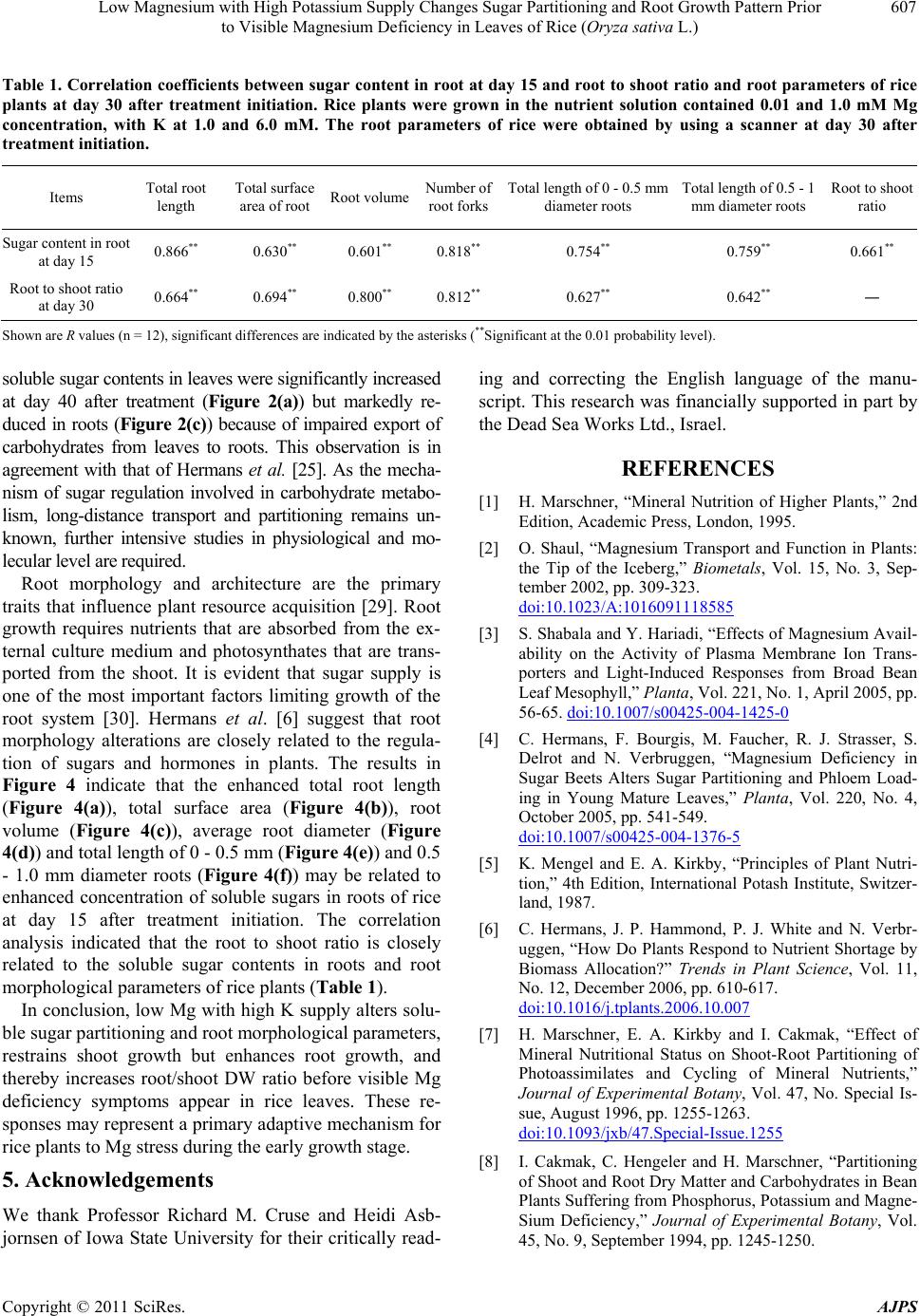 Low Magnesium with High Potassium Supply Changes Sugar Partitioning and Root Growth Pattern Prior 607 to Visible Magnesium Deficiency in Leaves of Rice (Oryza sativa L.) Table 1. Correlation coefficients between sugar content in root at day 15 and root to shoot ratio and root parameters of rice plants at day 30 after treatment initiation. Rice plants were grown in the nutrient solution contained 0.01 and 1.0 mM Mg concentration, with K at 1.0 and 6.0 mM. The root parameters of rice were obtained by using a scanner at day 30 after treatment initiation. Items Total root length Total surface area of root Root volumeNumber of root forks Total length of 0 - 0.5 mm diameter roots Total length of 0.5 - 1 mm diameter roots Root to shoot ratio Sugar content in root at day 15 0.866** 0.630** 0.601** 0.818** 0.754** 0.759** 0.661** Root to shoot ratio at day 30 0.664** 0.694** 0.800** 0.812** 0.627** 0.642** ― Shown are R values (n = 12), significant differences are indicated by the asterisks (**Significant at the 0.01 probability level). soluble sugar contents in leaves were significantly increased at day 40 after treatment (Figure 2(a)) but markedly re- duced in roots (Figure 2(c)) because of impaired export of carbohydrates from leaves to roots. This observation is in agreement with that of Hermans et al. [25]. As the mecha- nism of sugar regulation involved in carbohydrate metabo- lism, long-distance transport and partitioning remains un- known, further intensive studies in physiological and mo- lecular level are required. Root morphology and architecture are the primary traits that influence plant resource acquisition [29]. Root growth requires nutrients that are absorbed from the ex- ternal culture medium and photosynthates that are trans- ported from the shoot. It is evident that sugar supply is one of the most important factors limiting growth of the root system [30]. Hermans et al. [6] suggest that root morphology alterations are closely related to the regula- tion of sugars and hormones in plants. The results in Figure 4 indicate that the enhanced total root length (Figure 4(a)), total surface area (Figure 4(b)), root volume (Figure 4(c)), average root diameter (Figure 4(d)) and total length of 0 - 0.5 mm (Figure 4(e)) and 0.5 - 1.0 mm diameter roots (Figure 4(f)) may be related to enhanced concentration of soluble sugars in roots of rice at day 15 after treatment initiation. The correlation analysis indicated that the root to shoot ratio is closely related to the soluble sugar contents in roots and root morphological parameters of rice plants (Table 1). In conclusion, low Mg with high K supply alters solu- ble sugar partitioning and root morphological parameters, restrains shoot growth but enhances root growth, and thereby increases root/shoot DW ratio before visible Mg deficiency symptoms appear in rice leaves. These re- sponses may represent a primary adaptive mechanism for rice plants to Mg stress during the early growth stage. 5. Acknowledgements We thank Professor Richard M. Cruse and Heidi Asb- jornsen of Iowa State University for their critically read- ing and correcting the English language of the manu- script. This research was financially supported in part by the Dead Sea Works Ltd., Israel. REFERENCES [1] H. Marschner, “Mineral Nutrition of Higher Plants,” 2nd Edition, Academic Press, London, 1995. [2] O. Shaul, “Magnesium Transport and Function in Plants: the Tip of the Iceberg,” Biometals, Vol. 15, No. 3, Sep- tember 2002, pp. 309-323. doi:10.1023/A:1016091118585 [3] S. Shabala and Y. Hariadi, “Effects of Magnesium Avail- ability on the Activity of Plasma Membrane Ion Trans- porters and Light-Induced Responses from Broad Bean Leaf Mesophyll,” Planta, Vol. 221, No. 1, April 2005, pp. 56-65. doi:10.1007/s00425-004-1425-0 [4] C. Hermans, F. Bourgis, M. Faucher, R. J. Strasser, S. Delrot and N. Verbruggen, “Magnesium Deficiency in Sugar Beets Alters Sugar Partitioning and Phloem Load- ing in Young Mature Leaves,” Planta, Vol. 220, No. 4, October 2005, pp. 541-549. doi:10.1007/s00425-004-1376-5 [5] K. Mengel and E. A. Kirkby, “Principles of Plant Nutri- tion,” 4th Edition, International Potash Institute, Switzer- land, 1987. [6] C. Hermans, J. P. Hammond, P. J. White and N. Verbr- uggen, “How Do Plants Respond to Nutrient Shortage by Biomass Allocation?” Trends in Plant Science, Vol. 11, No. 12, December 2006, pp. 610-617. doi:10.1016/j.tplants.2006.10.007 [7] H. Marschner, E. A. Kirkby and I. Cakmak, “Effect of Mineral Nutritional Status on Shoot-Root Partitioning of Photoassimilates and Cycling of Mineral Nutrients,” Journal of Experimental Botany, Vol. 47, No. Special Is- sue, August 1996, pp. 1255-1263. doi:10.1093/jxb/47.Special-Issue.1255 [8] I. Cakmak, C. Hengeler and H. Marschner, “Partitioning of Shoot and Root Dry Matter and Carbohydrates in Bean Plants Suffering from Phosphorus, Potassium and Magne- Sium Deficiency,” Journal of Experimental Botany, Vol. 45, No. 9, September 1994, pp. 1245-1250. Copyright © 2011 SciRes. AJPS
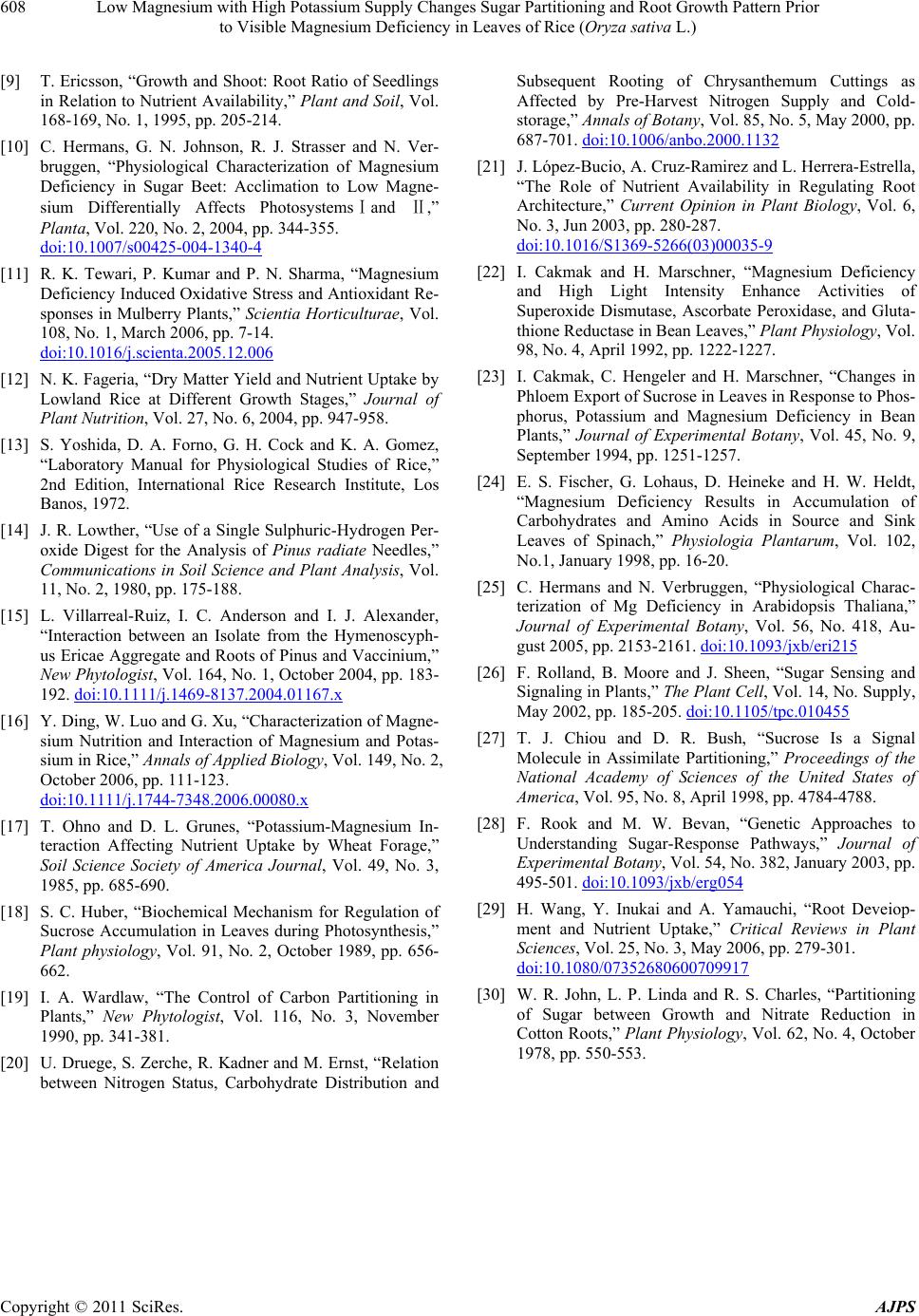 Low Magnesium with High Potassium Supply Changes Sugar Partitioning and Root Growth Pattern Prior 608 to Visible Magnesium Deficiency in Leaves of Rice (Oryza sativa L.) [9] T. Ericsson, “Growth and Shoot: Root Ratio of Seedlings in Relation to Nutrient Availability,” Plant and Soil, Vol. 168-169, No. 1, 1995, pp. 205-214. [10] C. Hermans, G. N. Johnson, R. J. Strasser and N. Ver- bruggen, “Physiological Characterization of Magnesium Deficiency in Sugar Beet: Acclimation to Low Magne- sium Differentially Affects PhotosystemsⅠand Ⅱ,” Planta, Vol. 220, No. 2, 2004, pp. 344-355. doi:10.1007/s00425-004-1340-4 [11] R. K. Tewari, P. Kumar and P. N. Sharma, “Magnesium Deficiency Induced Oxidative Stress and Antioxidant Re- sponses in Mulberry Plants,” Scientia Horticulturae, Vol. 108, No. 1, March 2006, pp. 7-14. doi:10.1016/j.scienta.2005.12.006 [12] N. K. Fageria, “Dry Matter Yield and Nutrient Uptake by Lowland Rice at Different Growth Stages,” Journal of Plant Nutrition, Vol. 27, No. 6, 2004, pp. 947-958. [13] S. Yoshida, D. A. Forno, G. H. Cock and K. A. Gomez, “Laboratory Manual for Physiological Studies of Rice,” 2nd Edition, International Rice Research Institute, Los Banos, 1972. [14] J. R. Lowther, “Use of a Single Sulphuric-Hydrogen Per- oxide Digest for the Analysis of Pinus radiate Needles,” Communications in Soil Science and Plant Analysis, Vol. 11, No. 2, 1980, pp. 175-188. [15] L. Villarreal-Ruiz, I. C. Anderson and I. J. Alexander, “Interaction between an Isolate from the Hymenoscyph- us Ericae Aggregate and Roots of Pinus and Vaccinium,” New Phytologist, Vol. 164, No. 1, October 2004, pp. 183- 192. doi:10.1111/j.1469-8137.2004.01167.x [16] Y. Ding, W. Luo and G. Xu, “Characterization of Magne- sium Nutrition and Interaction of Magnesium and Potas- sium in Rice,” Annals of Applied Biology, Vol. 149, No. 2, October 2006, pp. 111-123. doi:10.1111/j.1744-7348.2006.00080.x [17] T. Ohno and D. L. Grunes, “Potassium-Magnesium In- teraction Affecting Nutrient Uptake by Wheat Forage,” Soil Science Society of America Journal, Vol. 49, No. 3, 1985, pp. 685-690. [18] S. C. Huber, “Biochemical Mechanism for Regulation of Sucrose Accumulation in Leaves during Photosynthesis,” Plant physiology, Vol. 91, No. 2, October 1989, pp. 656- 662. [19] I. A. Wardlaw, “The Control of Carbon Partitioning in Plants,” New Phytologist, Vol. 116, No. 3, November 1990, pp. 341-381. [20] U. Druege, S. Zerche, R. Kadner and M. Ernst, “Relation between Nitrogen Status, Carbohydrate Distribution and Subsequent Rooting of Chrysanthemum Cuttings as Affected by Pre-Harvest Nitrogen Supply and Cold- storage,” Annals of Botany, Vol. 85, No. 5, May 2000, pp. 687-701. doi:10.1006/anbo.2000.1132 [21] J. López-Bucio, A. Cruz-Ramirez and L. Herrera-Estrella, “The Role of Nutrient Availability in Regulating Root Architecture,” Current Opinion in Plant Biology, Vol. 6, No. 3, Jun 2003, pp. 280-287. doi:10.1016/S1369-5266(03)00035-9 [22] I. Cakmak and H. Marschner, “Magnesium Deficiency and High Light Intensity Enhance Activities of Superoxide Dismutase, Ascorbate Peroxidase, and Gluta- thione Reductase in Bean Leaves,” Plant Physiology, Vol. 98, No. 4, April 1992, pp. 1222-1227. [23] I. Cakmak, C. Hengeler and H. Marschner, “Changes in Phloem Export of Sucrose in Leaves in Response to Phos- phorus, Potassium and Magnesium Deficiency in Bean Plants,” Journal of Experimental Botany, Vol. 45, No. 9, September 1994, pp. 1251-1257. [24] E. S. Fischer, G. Lohaus, D. Heineke and H. W. Heldt, “Magnesium Deficiency Results in Accumulation of Carbohydrates and Amino Acids in Source and Sink Leaves of Spinach,” Physiologia Plantarum, Vol. 102, No.1, January 1998, pp. 16-20. [25] C. Hermans and N. Verbruggen, “Physiological Charac- terization of Mg Deficiency in Arabidopsis Thaliana,” Journal of Experimental Botany, Vol. 56, No. 418, Au- gust 2005, pp. 2153-2161. doi:10.1093/jxb/eri215 [26] F. Rolland, B. Moore and J. Sheen, “Sugar Sensing and Signaling in Plants,” The Plant Cell, Vol. 14, No. Supply, May 2002, pp. 185-205. doi:10.1105/tpc.010455 [27] T. J. Chiou and D. R. Bush, “Sucrose Is a Signal Molecule in Assimilate Partitioning,” Proceedings of the National Academy of Sciences of the United States of America, Vol. 95, No. 8, April 1998, pp. 4784-4788. [28] F. Rook and M. W. Bevan, “Genetic Approaches to Understanding Sugar-Response Pathways,” Journal of Experimental Botany, Vol. 54, No. 382, January 2003, pp. 495-501. doi:10.1093/jxb/erg054 [29] H. Wang, Y. Inukai and A. Yamauchi, “Root Deveiop- ment and Nutrient Uptake,” Critical Reviews in Plant Sciences, Vol. 25, No. 3, May 2006, pp. 279-301. doi:10.1080/07352680600709917 [30] W. R. John, L. P. Linda and R. S. Charles, “Partitioning of Sugar between Growth and Nitrate Reduction in Cotton Roots,” Plant Physiology, Vol. 62, No. 4, October 1978, pp. 550-553. Copyright © 2011 SciRes. AJPS
|