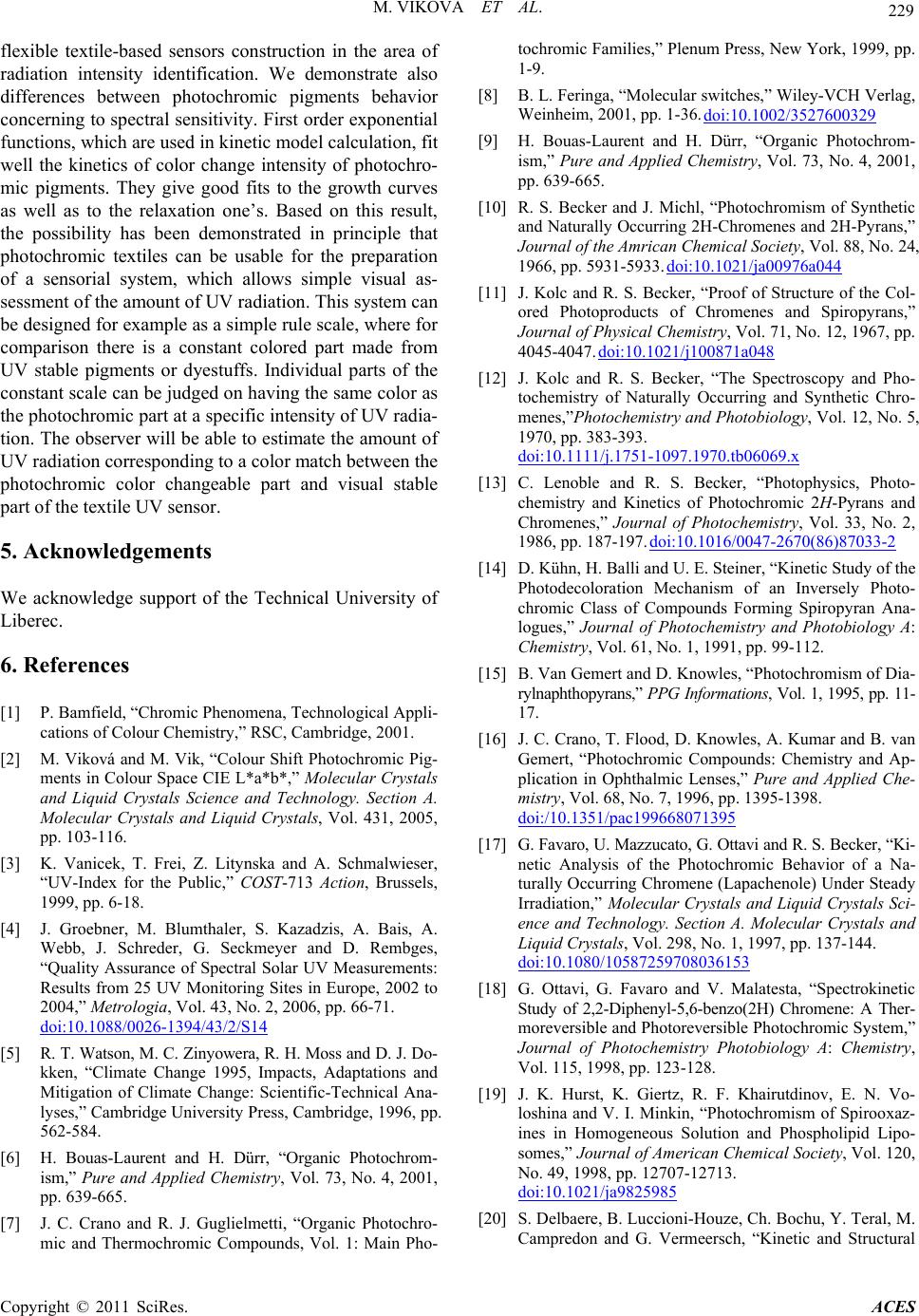
M. VIKOVA ET AL.
229
flexible textile-based sensors construction in the area of
radiation intensity identification. We demonstrate also
differences between photochromic pigments behavior
concerning to spectral sensitivity. First order exponential
functions, which are used in kinetic model calculation, fit
well the kinetics of color change intensity of photochro-
mic pigments. They give good fits to the growth curves
as well as to the relaxation one’s. Based on this result,
the possibility has been demonstrated in principle that
photochromic textiles can be usable for the preparation
of a sensorial system, which allows simple visual as-
sessment of the amount of UV radiation. This system can
be designed for example as a simple rule scale, where for
comparison there is a constant colored part made from
UV stable pigments or dyestuffs. Individual parts of the
constant scale can be judged on having the same color as
the photochromic part at a specific intensity of UV radia-
tion. The observer will be able to estimate the amount of
UV radiation corresponding to a color match between the
photochromic color changeable part and visual stable
part of the textile UV sensor.
5. Acknowledgements
We acknowledge support of the Technical University of
Liberec.
6. References
[1] P. Bamfield, “Chromic Phenomena, Technological Appli-
cations of Colour Chemistry,” RSC, Cambridge, 2001.
[2] M. Viková and M. Vik, “Colour Shift Photochromic Pig-
ments in Colour Space CIE L*a*b*,” Molecular Crystals
and Liquid Crystals Science and Technology. Section A.
Molecular Crystals and Liquid Crystals, Vol. 431, 2005,
pp. 103-116.
[3] K. Vanicek, T. Frei, Z. Litynska and A. Schmalwieser,
“UV-Index for the Public,” COST-713 Action, Brussels,
1999, pp. 6-18.
[4] J. Groebner, M. Blumthaler, S. Kazadzis, A. Bais, A.
Webb, J. Schreder, G. Seckmeyer and D. Rembges,
“Quality Assurance of Spectral Solar UV Measurements:
Results from 25 UV Monitoring Sites in Europe, 2002 to
2004,” Metrologia, Vol. 43, No. 2, 2006, pp. 66-71.
doi:10.1088/0026-1394/43/2/S14
[5] R. T. Watson, M. C. Zinyowera, R. H. Moss and D. J. Do-
kken, “Climate Change 1995, Impacts, Adaptations and
Mitigation of Climate Change: Scientific-Technical Ana-
lyses,” Cambridge University Press, Cambridge, 1996, pp.
562-584.
[6] H. Bouas-Laurent and H. Dürr, “Organic Photochrom-
ism,” Pure and Applied Chemistry, Vol. 73, No. 4, 2001,
pp. 639-665.
[7] J. C. Crano and R. J. Guglielmetti, “Organic Photochro-
mic and Thermochromic Compounds, Vol. 1: Main Pho-
tochromic Families,” Plenum Press, New York, 1999, pp.
1-9.
[8] B. L. Feringa, “Molecular switches,” Wiley-VCH Verlag,
Weinheim, 2001, pp. 1-36. doi:10.1002/3527600329
[9] H. Bouas-Laurent and H. Dürr, “Organic Photochrom-
ism,” Pure and Applied Chemistry, Vol. 73, No. 4, 2001,
pp. 639-665.
[10] R. S. Becker and J. Michl, “Photochromism of Synthetic
and Naturally Occurring 2H-Chromenes and 2H-Pyrans,”
Journal of the Amrican Chemical Society, Vol. 88, No. 24,
1966, pp. 5931-5933. doi:10.1021/ja00976a044
[11] J. Kolc and R. S. Becker, “Proof of Structure of the Col-
ored Photoproducts of Chromenes and Spiropyrans,”
Journal of Physical Chemistry, Vol. 71, No. 12, 1967, pp.
4045-4047. doi:10.1021/j100871a048
[12] J. Kolc and R. S. Becker, “The Spectroscopy and Pho-
tochemistry of Naturally Occurring and Synthetic Chro-
menes,”Photochemistry and Photobiology, Vol. 12, No. 5,
1970, pp. 383-393.
doi:10.1111/j.1751-1097.1970.tb06069.x
[13] C. Lenoble and R. S. Becker, “Photophysics, Photo-
chemistry and Kinetics of Photochromic 2H-Pyrans and
Chromenes,” Journal of Photochemistry, Vol. 33, No. 2,
1986, pp. 187-197. doi:10.1016/0047-2670(86)87033-2
[14] D. Kühn, H. Balli and U. E. Steiner, “Kinetic Study of the
Photodecoloration Mechanism of an Inversely Photo-
chromic Class of Compounds Forming Spiropyran Ana-
logues,” Journal of Photochemistry and Photobiology A:
Chemistry, Vol. 61, No. 1, 1991, pp. 99-112.
[15] B. Van Gemert and D. Knowles, “Photochromism of Dia-
rylnaphthopyrans,” PPG Informations, Vol. 1, 1995, pp. 11-
17.
[16] J. C. Crano, T. Flood, D. Knowles, A. Kumar and B. van
Gemert, “Photochromic Compounds: Chemistry and Ap-
plication in Ophthalmic Lenses,” Pure and Applied Che-
mistry, Vol. 68, No. 7, 1996, pp. 1395-1398.
doi:/10.1351/pac199668071395
[17] G. Favaro, U. Mazzucato, G. Ottavi and R. S. Becker, “Ki-
netic Analysis of the Photochromic Behavior of a Na-
turally Occurring Chromene (Lapachenole) Under Steady
Irradiation,” Molecular Crystals and Liquid Crystals Sci-
ence and Technology. Section A. Molecular Crystals and
Liquid Crystals, Vol. 298, No. 1, 1997, pp. 137-144.
doi:10.1080/10587259708036153
[18] G. Ottavi, G. Favaro and V. Malatesta, “Spectrokinetic
Study of 2,2-Diphenyl-5,6-benzo(2H) Chromene: A Ther-
moreversible and Photoreversible Photochromic System,”
Journal of Photochemistry Photobiology A: Chemistry,
Vol. 115, 1998, pp. 123-128.
[19] J. K. Hurst, K. Giertz, R. F. Khairutdinov, E. N. Vo-
loshina and V. I. Minkin, “Photochromism of Spirooxaz-
ines in Homogeneous Solution and Phospholipid Lipo-
somes,” Journal of American Chemical Society, Vol. 120,
No. 49, 1998, pp. 12707-12713.
doi:10.1021/ja9825985
[20] S. Delbaere, B. Luccioni-Houze, Ch. Bochu, Y. Teral, M.
Campredon and G. Vermeersch, “Kinetic and Structural
Copyright © 2011 SciRes. ACES