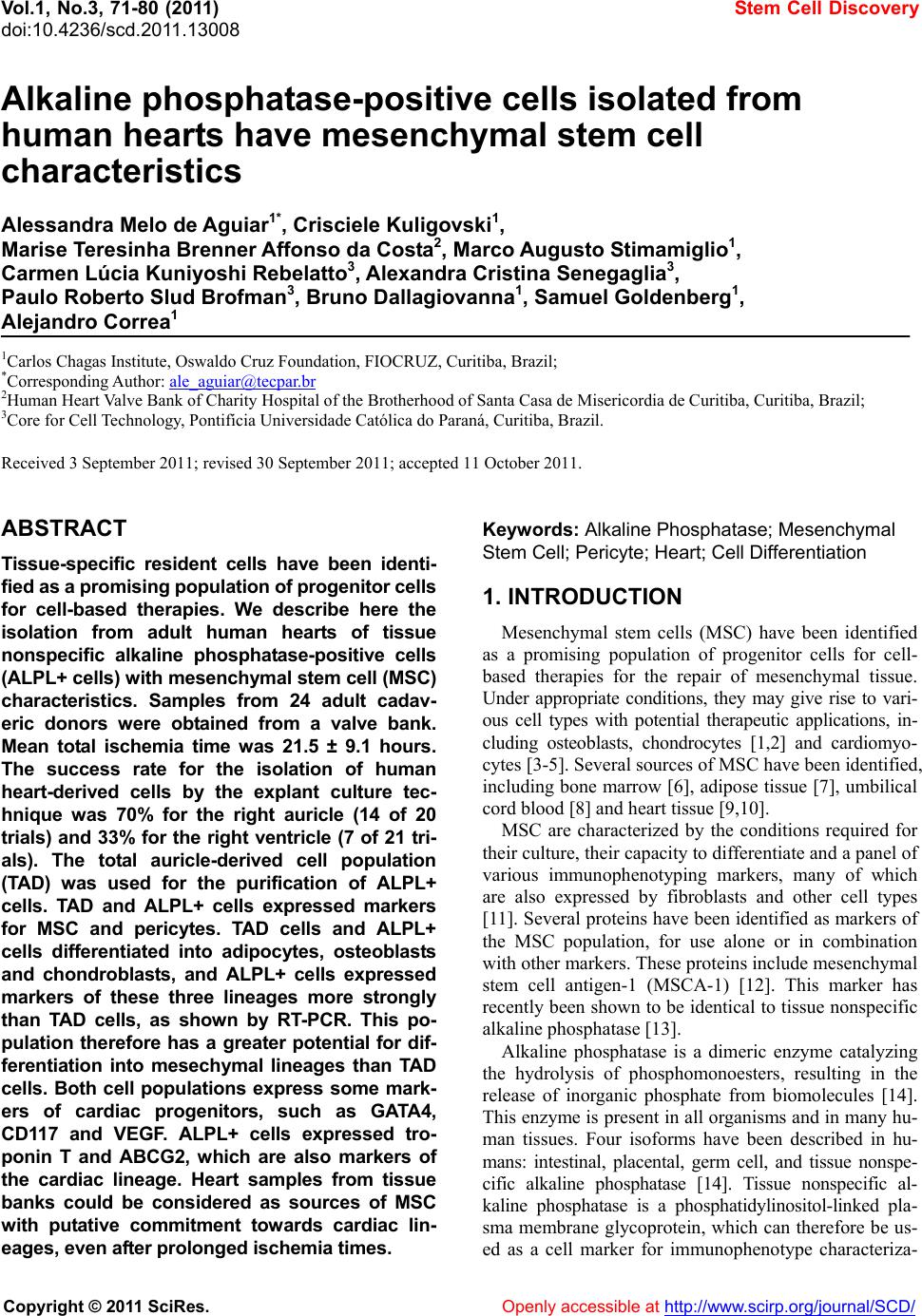 Vol.1, No.3, 71-80 (2011) doi:10.4236/scd.2011.13008 C opyright © 2011 SciRes. Openly accessible at http://www.scirp.org/journal/SCD/ Stem Cell Discovery Alkaline phosphatase-pos itive cells isolate d from human hearts have mesenchymal stem cell characteristics Alessandra Melo de Aguiar1*, Crisciele Kuligovski1, Marise Teresinha Brenner Affonso da Costa2, Marco Augusto Stimamiglio1, Carmen Lúcia Kuniyoshi Rebelatto3, Alexandra Cristina Senegaglia3, Paulo Roberto Slud Brofman3, Bruno Dallagiovanna1, Samuel Goldenberg1, Alejandro Correa1 1Carlos Chagas Institute, Oswaldo Cruz Foundation, FIOCRUZ, Curitiba, Brazil; *Corresponding Aut hor: ale_aguiar@tecpar.br 2Human Heart Valve Bank of Charity Hospital of the Brotherhood of Santa Casa de Misericordia de Curitiba, Curitiba, Brazil; 3Core for Cell Technology, Pontifícia Universidade Católica do Paraná, Curitiba, Brazil. Received 3 September 2011; revised 30 September 2011; accepted 11 October 2011. ABSTRACT Tissue-specific resident cells have been identi- fied as a promising population of progenitor cells for cell-based therapies. We describe here the isolation from adult human hearts of tissue nonspecific alkaline phosphatase-positive cells (ALPL+ cells) with mesenchymal stem cell (MSC) characteristics. Samples from 24 adult cadav- eric donors were obtained from a valve bank. Mean total ischemia time was 21.5 ± 9.1 hours. The success rate for the isolation of human heart-derived cells by the explant culture tec- hnique was 70% for the right auricle (14 of 20 trials) and 33% for the right ventricle (7 of 21 tri- als). The total auricle-derived cell population (TAD) was used for the purification of ALPL+ cells. TAD and ALPL+ cells expressed markers for MSC and pericytes. TAD cells and ALPL+ cells differentiated into adipocytes, osteoblasts and chondroblasts, and ALPL+ cells expressed markers of these three lineages more strongly than TAD cells, as shown by RT-PCR. This po- pulation therefore has a greater potential for dif- ferentiation into mesechymal lineages than TAD cells. Both cell populations express some mark- ers of cardiac progenitors, such as GATA4, CD117 and VEGF. ALPL+ cells expressed tro- ponin T and ABCG2, which are also markers of the cardiac lineage. Heart samples from tissue banks could be considered as sources of MSC with putative commitment towards cardiac lin- eages, even af ter prolonged ischemia times. Keywords: Alkaline Phosphatase; Mesenchymal Stem Cell; Pericyte; Heart; Cell Differentiation 1. INTRODUCTION Mesenchymal stem cells (MSC) have been identified as a promising population of progenitor cells for cell- based therapies for the repair of mesenchymal tissue. Under appropriate conditions, they may give rise to vari- ous cell types with potential therapeutic applications, in- cluding osteoblasts, chondrocytes [1,2] and cardiomyo- cytes [3-5]. Several sources of MSC have been identified, including bone marrow [6], adipose tissue [7], umbilical cord blood [8] and heart tissue [9,10]. MSC are characterized by the conditions required for their culture, their capacity to differentiate and a panel of various immunophenotyping markers, many of which are also expressed by fibroblasts and other cell types [11]. Several proteins have been identified as markers of the MSC population, for use alone or in combination with other markers. These proteins include mesenchymal stem cell antigen-1 (MSCA-1) [12]. This marker has recently been shown to be identical to tissue nonspecific alkaline phosphatase [13]. Alkaline phosphatase is a dimeric enzyme catalyzing the hydrolysis of phosphomonoesters, resulting in the release of inorganic phosphate from biomolecules [14]. This enzyme is present in all organisms and in many hu- man tissues. Four isoforms have been described in hu- mans: intestinal, placental, germ cell, and tissue nonspe- cific alkaline phosphatase [14]. Tissue nonspecific al- kaline phosphatase is a phosphatidylinositol-linked pla- sma membrane glycoprotein, which can therefore be us- ed as a cell marker for immunophenotype characteriza-
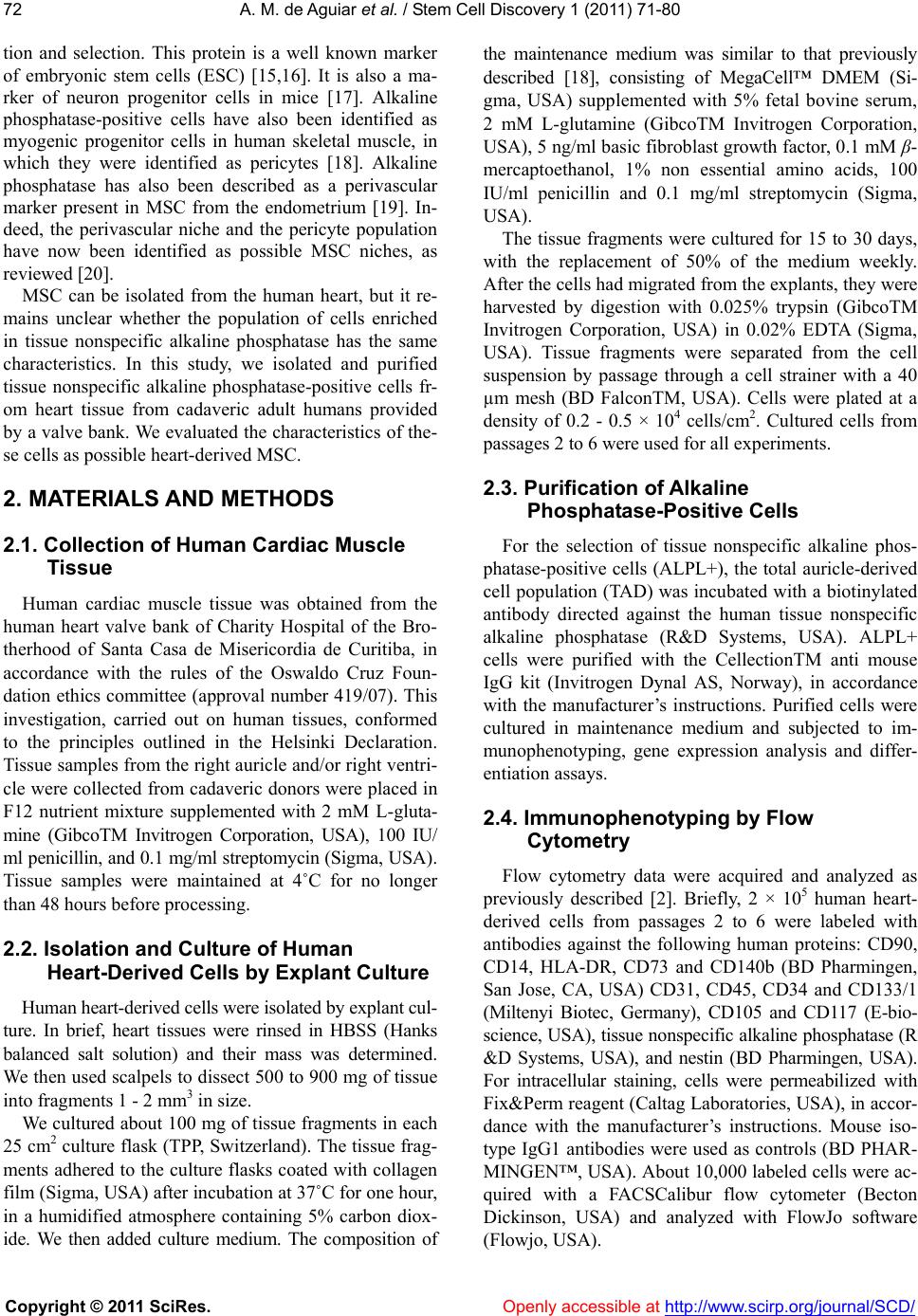 A. M. de Aguiar et al. / Stem Cell Discovery 1 (2011) 71-80 Copyright © 2011 SciRes. Openl y accessible at http://www.scirp.org/journal/SCD/ 72 tion and selection. This protein is a well known marker of embryonic stem cells (ESC) [15,16]. It is also a ma- rker of neuron progenitor cells in mice [17]. Alkaline phosphatase-positive cells have also been identified as myogenic progenitor cells in human skeletal muscle, in which they were identified as pericytes [18]. Alkaline phosphatase has also been described as a perivascular marker present in MSC from the endometrium [19]. In- deed, the perivascular niche and the pericyte population have now been identified as possible MSC niches, as reviewed [20]. MSC can be isolated from the human heart, but it re- mains unclear whether the population of cells enriched in tissue nonspecific alkaline phosphatase has the same characteristics. In this study, we isolated and purified tissue nonspecific alkaline phosphatase-positive cells fr- om heart tissue from cadaveric adult humans provided by a valve bank. We evaluated the characteristics of the- se cells as possible heart-derived MSC. 2. MATERIALS AND METHODS 2.1. Collection of Human Cardiac Muscle Tissue Human cardiac muscle tissue was obtained from the human heart valve bank of Charity Hospital of the Bro- therhood of Santa Casa de Misericordia de Curitiba, in accordance with the rules of the Oswaldo Cruz Foun- dation ethics committee (approval number 419/07). This investigation, carried out on human tissues, conformed to the principles outlined in the Helsinki Declaration. Tissue samples from the right auricle and/or right ventri- cle were collected from cadaveric donors were placed in F12 nutrient mixture supplemented with 2 mM L-gluta- mine (GibcoTM Invitrogen Corporation, USA), 100 IU/ ml penicillin, and 0.1 mg/ml streptomycin (Sigma, USA ). Tissue samples were maintained at 4˚C for no longer than 48 hours before processing. 2.2. Isolation and Culture of Human Heart-Derived Cells by Explant Culture Human heart-derived cells were isolated by explant cul- ture. In brief, heart tissues were rinsed in HBSS (Hanks balanced salt solution) and their mass was determined. We then used scalpels to dissect 500 to 900 mg of tissue into fragments 1 - 2 mm3 in size. We cultured about 100 mg of tissue fragments in each 25 cm2 culture flask (TPP, Switzerland). The tissue frag- ments adhered to the culture flasks coated with collagen film (Sigma, USA) after incubation at 37˚C for one hour, in a humidified atmosphere containing 5% carbon diox- ide. We then added culture medium. The composition of the maintenance medium was similar to that previously described [18], consisting of MegaCell™ DMEM (Si- gma, USA) supplemented with 5% fetal bovine serum, 2 mM L-glutamine (GibcoTM Invitrogen Corporation, USA), 5 ng/ml basic fibroblast growth factor, 0.1 mM β- mercaptoethanol, 1% non essential amino acids, 100 IU/ml penicillin and 0.1 mg/ml streptomycin (Sigma, USA). The tissue fragments were cultured for 15 to 30 days, with the replacement of 50% of the medium weekly. After the cells had migrated from the explants, they were harvested by digestion with 0.025% trypsin (GibcoTM Invitrogen Corporation, USA) in 0.02% EDTA (Sigma, USA). Tissue fragments were separated from the cell suspension by passage through a cell strainer with a 40 µm mesh (BD FalconTM, USA). Cells were plated at a density of 0.2 - 0.5 × 104 cells/cm2. Cultured cells from passages 2 to 6 were use d for al l expe ri ments. 2.3. Purification of Alkaline Phosphatase-Positive Cells For the selection of tissue nonspecific alkaline phos- phatase-positive cells (ALPL+), the total auricle-derived cell population (TAD) was incubated with a biotin ylated antibody directed against the human tissue nonspecific alkaline phosphatase (R&D Systems, USA). ALPL+ cells were purified with the CellectionTM anti mouse IgG kit (Invitrogen Dynal AS, Norway), in accordance with the manufacturer ’s instructions. Purified cells were cultured in maintenance medium and subjected to im- munophenotyping, gene expression analysis and differ- entiation assays. 2.4. Immunophenotyping by Flow Cytometry Flow cytometry data were acquired and analyzed as previously described [2]. Briefly, 2 × 105 human heart- derived cells from passages 2 to 6 were labeled with antibodies against the following human proteins: CD90, CD14, HLA-DR, CD73 and CD140b (BD Pharmingen, San Jose, CA, USA) CD31, CD45, CD34 and CD133/1 (Miltenyi Biotec, Germany), CD105 and CD117 (E-bio- science, USA), tissue nonspecific alkaline phosphatase (R &D Systems, USA), and nestin (BD Pharmingen, USA). For intracellular staining, cells were permeabilized with Fix&Perm reagent (Caltag Laboratories, USA), in accor- dance with the manufacturer’s instructions. Mouse iso- type IgG1 antibodies were used as controls (BD PHAR- MINGEN™, USA). About 10,000 labeled cells were ac- quired with a FACSCalibur flow cytometer (Becton Dickinson, USA) and analyzed with FlowJo software (Flowjo, USA).
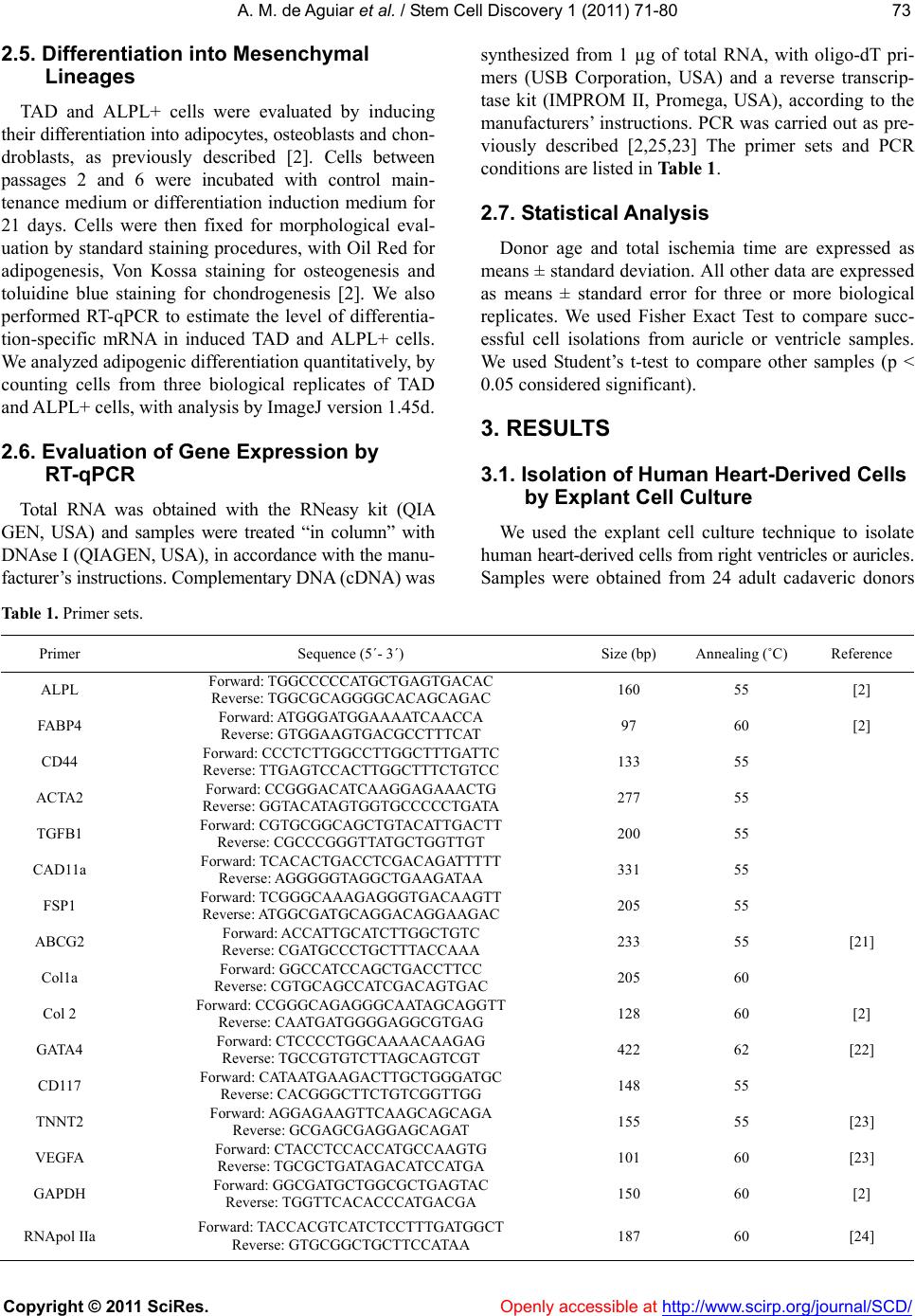 A. M. de Aguiar et al. / Stem Cell Discovery 1 (2011) 71-80 Copyright © 2011 SciRes. http://www.scirp.org/journal/SCD/Openly accessible at 7373 2.5. Differentiation into Mesenchymal Lineages TAD and ALPL+ cells were evaluated by inducing their differentiation into adipocytes, osteoblasts and cho n- droblasts, as previously described [2]. Cells between passages 2 and 6 were incubated with control main- tenance medium or differentiation induction medium for 21 days. Cells were then fixed for morphological eval- uation by standard staining procedures, with Oil Red for adipogenesis, Von Kossa staining for osteogenesis and toluidine blue staining for chondrogenesis [2]. We also performed RT-qPCR to estimate the level of differentia- tion-specific mRNA in induced TAD and ALPL+ cells. We analyzed adipogenic differentiation quantitatively, by counting cells from three biological replicates of TAD and ALPL+ cells, with analysis by ImageJ versio n 1.45d. 2.6. Evaluation of Gene Expression by RT-qPCR Total RNA was obtained with the RNeasy kit (QIA GEN, USA) and samples were treated “in column” with DNAse I (QIAGEN, USA), in accordance with the manu- facturer’s instructions. Complementary DNA (cDNA) was synthesized from 1 µg of total RNA, with oligo-dT pri- mers (USB Corporation, USA) and a reverse transcrip- tase kit (IMPROM II, Promega, USA), according to the manufacturers’ instructions. PCR was carried out as pre- viously described [2,25,23] The primer sets and PCR conditions are listed in Table 1. 2.7. Statistical Analysis Donor age and total ischemia time are expressed as means ± standard deviation. All other data are expressed as means ± standard error for three or more biological replicates. We used Fisher Exact Test to compare succ- essful cell isolations from auricle or ventricle samples. We used Student’s t-test to compare other samples (p < 0.05 considered significant). 3. RESULTS 3.1. Isolation of Human Heart-Derived Cells by Explant Cell Culture We used the explant cell culture technique to isolate hu ma n heart-derived cells from right ventricles or auricles. Samples were obtained from 24 adult cadaveric donors Table 1. Primer sets. Primer Sequence (5´- 3´) Size (bp) Annealing (˚C) Reference ALPL Forward: TGGCCCCCATGCTGAGTGACAC Reverse: TGGCGCAGGGGCACAGCAGAC 160 55 [2] FABP4 Forward: AT GGGATGGAAAATCAACCA Reverse: GTGGAAGTGACGCCTTTCAT 97 60 [2] CD44 Forward: CCCTCTTGGCCTTGGCTTTGATTC Reverse: TTGAGTCCACTTGGCTTTCTGTCC 133 55 ACTA2 Forward: CCGGGACATCAAGGAGAAACTG Reverse: GGTACATAGTGGTGCCCCCTGATA 277 55 TGFB1 Forward: CGTGCGGCAGCTGTACATTGACTT Reverse: CGCCCGGGTTATGCTGGTTGT 200 55 CAD11a Forward: TCACACTGACCTCGACAGATTTTT Reverse: AGGGGGT AGGCTGAAGATAA 331 55 FSP1 Forward: TCG GGCAAAGAGGGTGACAAGTT Reverse: AT GGCGATGCAGGACAGGAAGAC 205 55 ABCG2 Forward: ACCATTGCATCTTGGCTGTC Reverse: CGATGCCCTGCTTTACCAAA 233 55 [21] Col1a Forward: GGCCATCCAGCTGACCTTCC Reverse: CGTGCAGCCATCGACAGTGAC 205 60 Col 2 Forward: CCGGGCAGAGGGCAATAGCAGGTT Reverse: CAATGATGGGGAGGCGTGAG 128 60 [2] GATA4 Forward: CTCCCCTGGCAAAACAAGAG Reverse: TGCCGTGTCTTAGCAGTCGT 422 62 [22] CD117 Forward: CATAATGAAGACTTGCTGGGATGC Reverse: CACGGGCTTCTGTCGG TTGG 148 55 TNNT2 For ward: AGGAGAAGTTCAAGCAGCAGA Reverse: GCGAGCGAGGAGCAGAT 155 55 [23] VEGFA Forward: CTACCTCCACCATGCCAAGTG Reverse: TGCGCTGATAG ACAT CCAT G A 101 60 [23] GAPDH Forward: GGCGATGCTGGCGCTGAGTAC Reverse: TGGTTCACACCCATGACGA 150 60 [2] RNApol IIa Forward: TACCACGTCATCTCCTTTGATGGCT Reverse: GTGCGGCTGCTTCCATAA 187 60 [24]
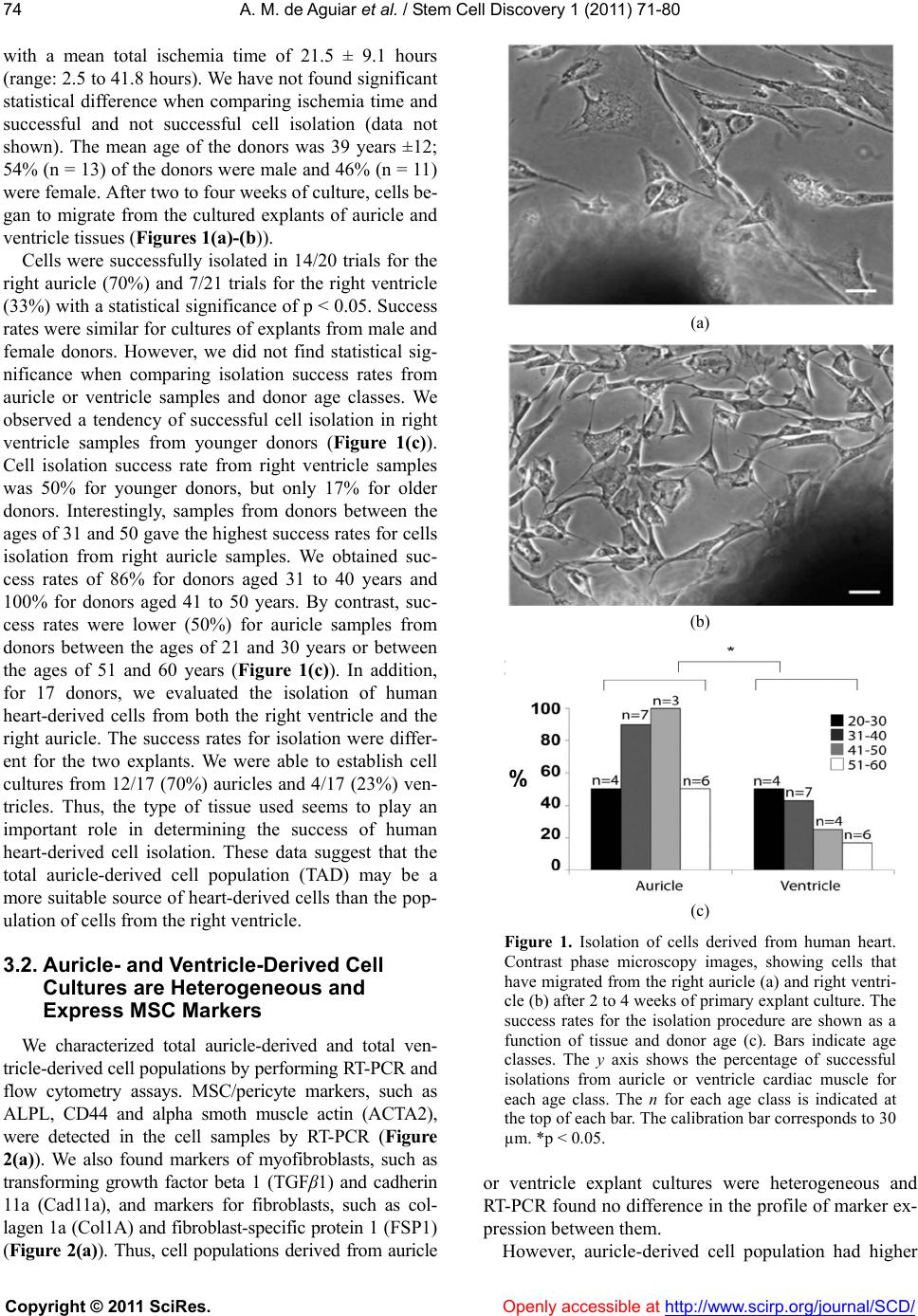 A. M. de Aguiar et al. / Stem Cell Discovery 1 (2011) 71-80 Copyright © 2011 SciRes. Openl y accessible at http://www.scirp.org/journal/SCD/ 74 with a mean total ischemia time of 21.5 ± 9.1 hours (range: 2.5 to 41.8 hours). We have not found significant statistical difference when comparing ischemia time and successful and not successful cell isolation (data not shown). The mean age of the donors was 39 years ±12; 54% (n = 13) of the donor s were male and 46% (n = 11) were female. After two to four weeks of culture, cells be- gan to migrate from the cultured explants of auricle and ventricle tissues (Fi gures 1(a)-(b)). Cells were successfully isolated in 14/20 trials for the right auricle (70%) and 7/21 trials for the right ventricle (33%) with a statistical significance of p < 0.05. Success rates were similar for cultures of explants from male and female donors. However, we did not find statistical sig- nificance when comparing isolation success rates from auricle or ventricle samples and donor age classes. We observed a tendency of successful cell isolation in right ventricle samples from younger donors (Figure 1(c)). Cell isolation success rate from right ventricle samples was 50% for younger donors, but only 17% for older donors. Interestingly, samples from donors between the ages of 31 and 50 gave the highest success rates for cells isolation from right auricle samples. We obtained suc- cess rates of 86% for donors aged 31 to 40 years and 100% for donors aged 41 to 50 years. By contrast, suc- cess rates were lower (50%) for auricle samples from donors between the ages of 21 and 30 years or between the ages of 51 and 60 years (Figure 1(c)). In addition, for 17 donors, we evaluated the isolation of human heart-derived cells from both the right ventricle and the right auricle. The success rates for isolation were differ- ent for the two explants. We were able to establish cell cultures from 12/17 (70%) auricles and 4/17 (23%) ven- tricles. Thus, the type of tissue used seems to play an important role in determining the success of human heart-derived cell isolation. These data suggest that the total auricle-derived cell population (TAD) may be a more suitable source of heart-derived cells than the pop- ulation of cells from the right ventricle. 3.2. Auricle- and Ventricle-Derived Cell Cultures are Heterogeneous and Express MSC Markers We characterized total auricle-derived and total ven- tricle-derived cell populations by performing RT-PCR and flow cytometry assays. MSC/pericyte markers, such as ALPL, CD44 and alpha smoth muscle actin (ACTA2), were detected in the cell samples by RT-PCR (Figure 2(a)). We also found markers of myofibroblasts, such as transforming growth factor beta 1 (TGFβ1) and cadherin 11a (Cad11a), and markers for fibroblasts, such as col- lagen 1a (Col1A) and fibroblast-specific protein 1 (FSP1) (Figure 2(a)). Thus, cell populations derived from auricle (a) (b) (c) Figure 1. Isolation of cells derived from human heart. Contrast phase microscopy images, showing cells that have migrated from the right auricle (a) and right ventri- cle (b) after 2 to 4 weeks of primary explant culture. The success rates for the isolation procedure are shown as a function of tissue and donor age (c). Bars indicate age classes. The y axis shows the percentage of successful isolations from auricle or ventricle cardiac muscle for each age class. The n for each age class is indicated at the top of each bar. The calibration bar corresponds to 30 µm. *p < 0.05. or ventricle explant cultures were heterogeneous and RT-PCR found no difference in the pro file of marker ex- pression between them. However, auricle-derived cell population had higher
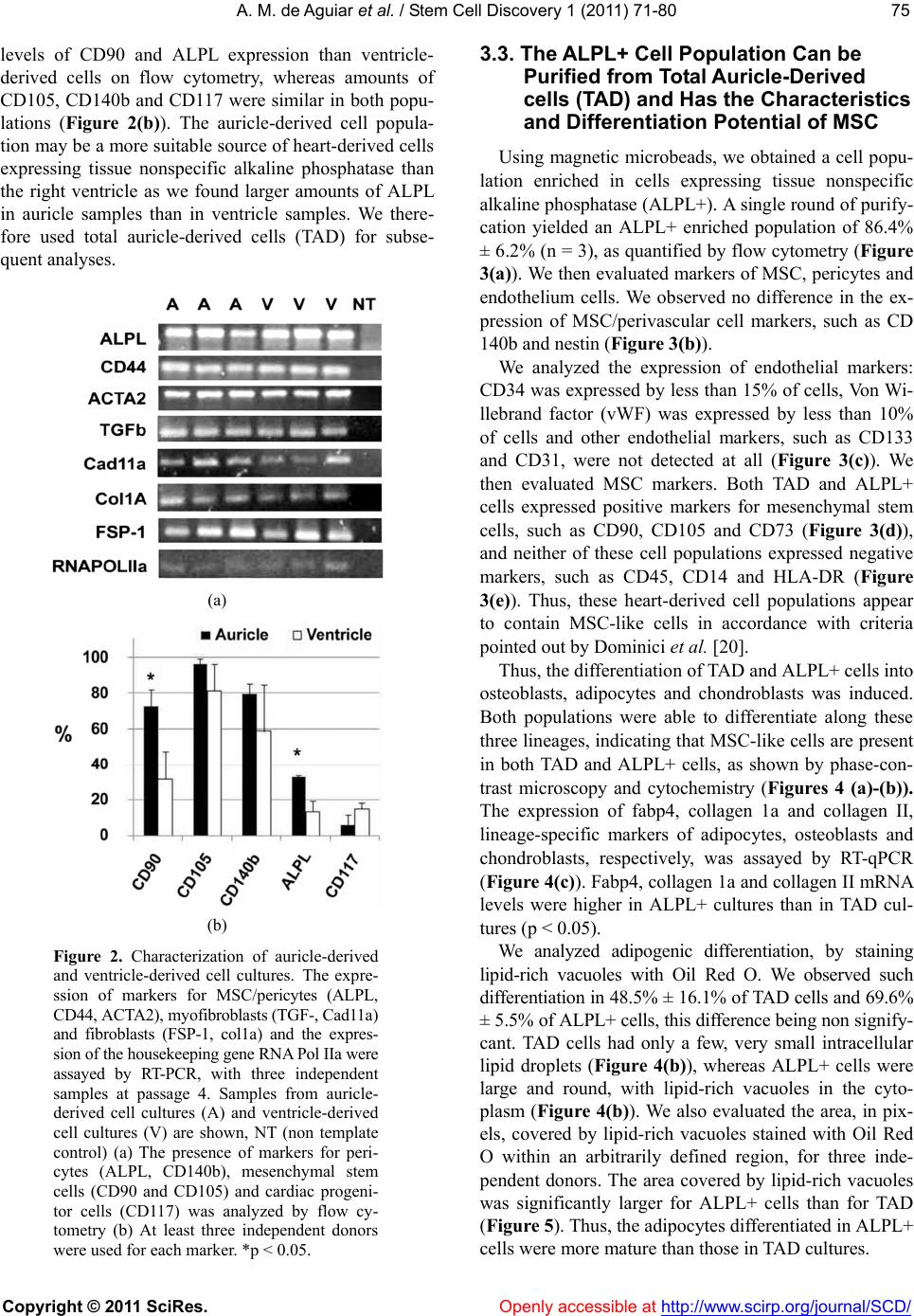 A. M. de Aguiar et al. / Stem Cell Discovery 1 (2011) 71-80 Copyright © 2011 SciRes. Openl y accessible at http://www.scirp.org/journal/SCD/ 7575 levels of CD90 and ALPL expression than ventricle- derived cells on flow cytometry, whereas amounts of CD105, CD140b and CD117 were similar in both popu- lations (Figure 2(b)). The auricle-derived cell popula- tion may be a more suitable source of heart-derived cells expressing tissue nonspecific alkaline phosphatase than the right ventricle as we found larger amounts of ALPL in auricle samples than in ventricle samples. We there- fore used total auricle-derived cells (TAD) for subse- quent analyses. (a) (b) Figure 2. Characterization of auricle-derived and ventricle-derived cell cultures. The expre- ssion of markers for MSC/pericytes (ALPL, CD44, ACTA2), myofibroblasts (TGF-, Cad11a) and fibroblasts (FSP-1, col1a) and the expres- sion of the housekeeping gene RNA Pol IIa were assayed by RT-PCR, with three independent samples at passage 4. Samples from auricle- derived cell cultures (A) and ventricle-derived cell cultures (V) are shown, NT (non template control) (a) The presence of markers for peri- cytes (ALPL, CD140b), mesenchymal stem cells (CD90 and CD105) and cardiac progeni- tor cells (CD117) was analyzed by flow cy- tometry (b) At least three independent donors were used for each marker. *p < 0.05. 3.3. The ALPL+ Cell Population Can be Purified from Total Auricle-Derived cells (TAD) and Has the Characteristics and Differentiation Potential of MSC Using magnetic microbeads, we obtained a cell popu- lation enriched in cells expressing tissue nonspecific alkaline phosphatase (ALPL+). A single round of purify- cation yielded an ALPL+ enriched population of 86.4% ± 6.2% (n = 3), as quantified by flow cytometry (Figure 3(a)). We then evaluated markers of MSC, pericytes and endothelium cells. We observed no difference in the ex- pression of MSC/perivascular cell markers, such as CD 140b and nestin (Figure 3(b)). We analyzed the expression of endothelial markers: CD34 was expressed by less than 15% of cells, Von Wi- llebrand factor (vWF) was expressed by less than 10% of cells and other endothelial markers, such as CD133 and CD31, were not detected at all (Figure 3(c)). We then evaluated MSC markers. Both TAD and ALPL+ cells expressed positive markers for mesenchymal stem cells, such as CD90, CD105 and CD73 (Figure 3(d)), and neither of these cell populations expressed negative markers, such as CD45, CD14 and HLA-DR (Figure 3(e)). Thus, these heart-derived cell populations appear to contain MSC-like cells in accordance with criteria pointed out by Dominici et al. [20]. Thus, the differentiation of TAD and ALPL+ cells into osteoblasts, adipocytes and chondroblasts was induced. Both populations were able to differentiate along these three lineages, indicating that MSC-like cells are present in both TAD and ALPL+ cells, as shown by phase-con- trast microscopy and cytochemistry (Figures 4 (a)-(b)). The expression of fabp4, collagen 1a and collagen II, lineage-specific markers of adipocytes, osteoblasts and chondroblasts, respectively, was assayed by RT-qPCR (Figure 4(c)). Fabp4, collagen 1a and collagen II mRNA levels were higher in ALPL+ cultures than in TAD cul- tures (p < 0.05). We analyzed adipogenic differentiation, by staining lipid-rich vacuoles with Oil Red O. We observed such differentiation in 48.5% ± 16.1% of TAD cells and 69.6% ± 5.5% of ALPL+ cells, this difference being non sig nif y - cant. TAD cells had only a few, very small intracellular lipid droplets (Figure 4(b)), whereas ALPL+ cells were large and round, with lipid-rich vacuoles in the cyto- plasm (Figure 4(b)). We also evaluated the area, in pix- els, covered by lipid-rich vacuoles stained with Oil Red O within an arbitrarily defined region, for three inde- pendent donors. The area covered by lipid-rich vacuoles was significantly larger for ALPL+ cells than for TAD (Figure 5). Thus, the adipocytes differentiated in ALPL+ cells were more mature than those in TAD cultures.
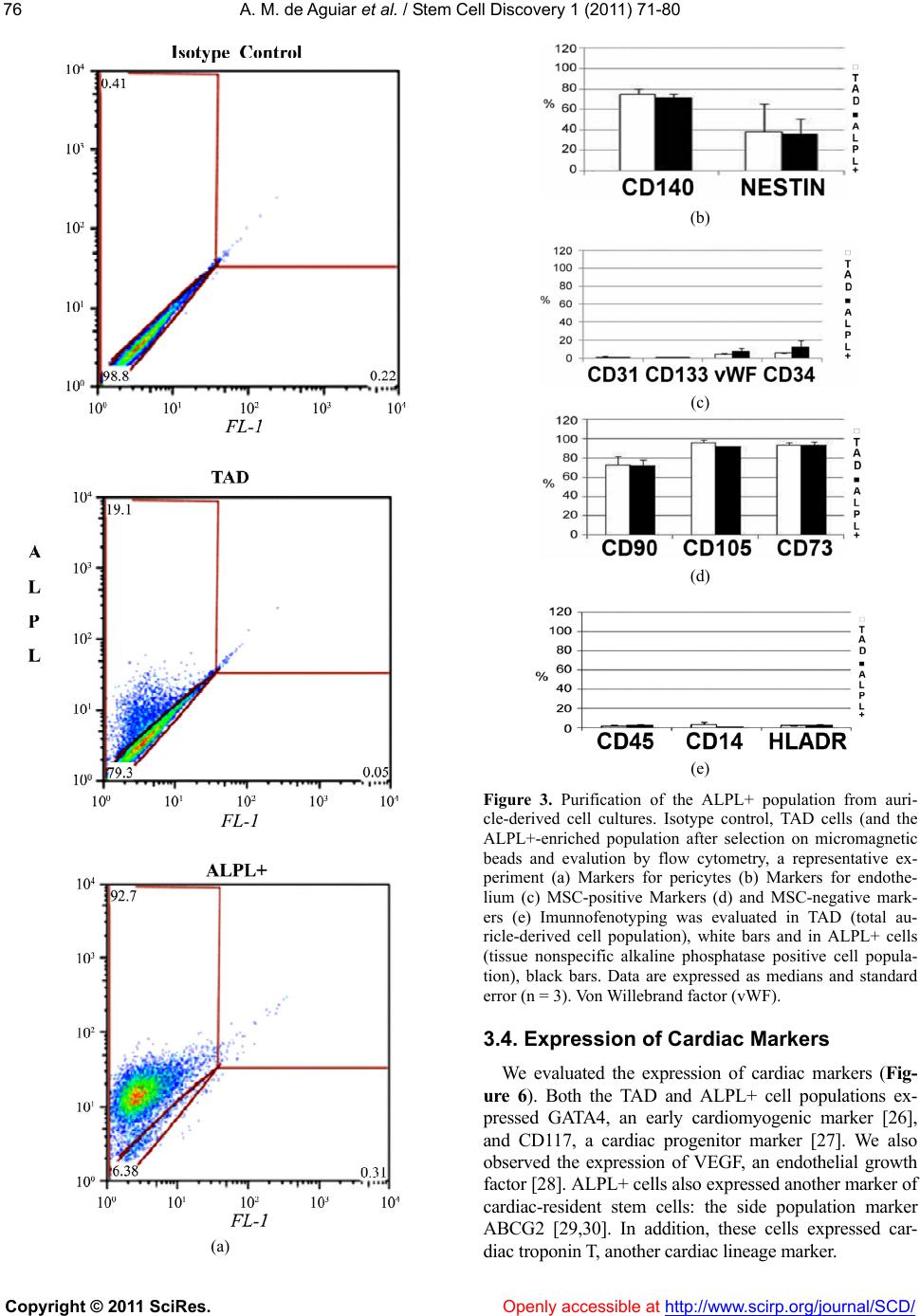 A. M. de Aguiar et al. / Stem Cell Discovery 1 (2011) 71-80 Copyright © 2011 SciRes. Openl y accessible at http://www.scirp.org/journal/SCD/ 76 (a) (b) (c) (d) (e) Figure 3. Purification of the ALPL+ population from auri- cle-derived cell cultures. Isotype control, TAD cells (and the ALPL+-enriched population after selection on micromagnetic beads and evalution by flow cytometry, a representative ex- periment (a) Markers for pericytes (b) Markers for endothe- lium (c) MSC-positive Markers (d) and MSC-negative mark- ers (e) Imunnofenotyping was evaluated in TAD (total au- ricle-derived cell population), white bars and in ALPL+ cells (tissue nonspecific alkaline phosphatase positive cell popula- tion), black bars. Data are expressed as medians and standard error (n = 3). Von Willebrand factor (vWF). 3.4. Expression of Cardiac Markers We evaluated the expression of cardiac markers (Fig- ure 6). Both the TAD and ALPL+ cell populations ex- pressed GATA4, an early cardiomyogenic marker [26], and CD117, a cardiac progenitor marker [27]. We also observed the expression of VEGF, an endothelial growth factor [28]. ALPL+ cells also expressed another marker of cardiac-resident stem cells: the side population marker ABCG2 [29,30]. In addition, these cells expressed car- diac troponin T, anot her cardi ac lineage marker.
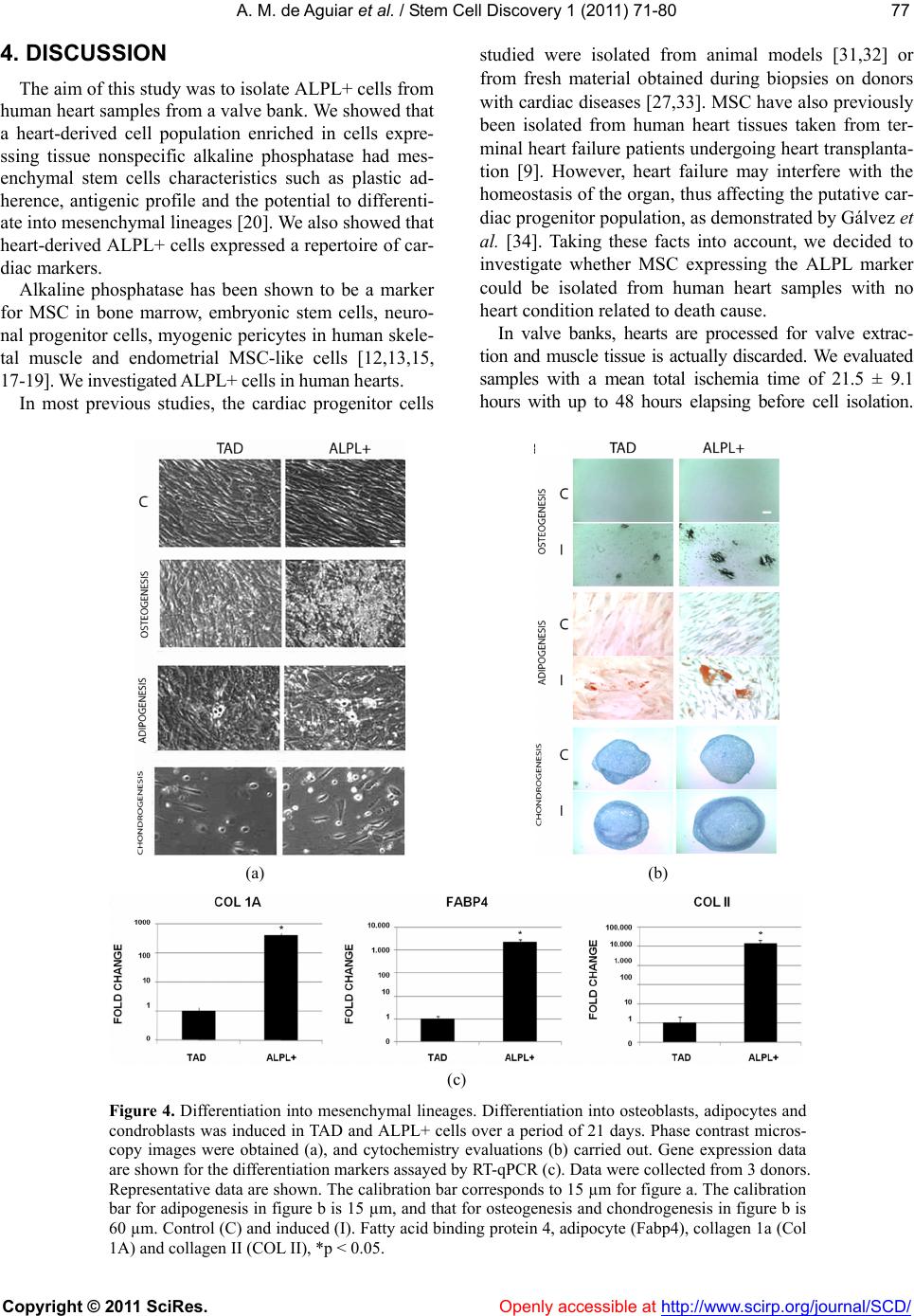 A. M. de Aguiar et al. / Stem Cell Discovery 1 (2011) 71-80 Copyright © 2011 SciRes. http://www.scirp.org/journal/SCD/ 7777 4. DISCUSSION studied were isolated from animal models [31,32] or from fresh material obtained during biopsies on donors with cardiac diseases [27,33]. MSC have also previously been isolated from human heart tissues taken from ter- minal heart failure patients undergoing heart transplanta- tion [9]. However, heart failure may interfere with the homeostasis of the organ, thus affecting the putative car- diac progenitor population, as demonstrated by Gálvez et al. [34]. Taking these facts into account, we decided to investigate whether MSC expressing the ALPL marker could be isolated from human heart samples with no heart condition related to death cause. The aim of this study was to isolate ALPL+ cells from human heart samples from a valve bank. We showed th at a heart-derived cell population enriched in cells expre- ssing tissue nonspecific alkaline phosphatase had mes- enchymal stem cells characteristics such as plastic ad- herence, antigenic profile and the potential to differenti- ate into mesenchymal lineages [20]. We also showed that heart-derived ALPL+ cells expressed a repertoire of car- diac markers. Alkaline phosphatase has been shown to be a marker for MSC in bone marrow, embryonic stem cells, neuro- nal progenitor cells, myogenic pericytes in human skele- tal muscle and endometrial MSC-like cells [12,13,15, 17-19]. We investigated ALPL+ cells in human hearts. In valve banks, hearts are processed for valve extrac- tion and muscle tissue is actually discarded. We evaluated samples with a mean total ischemia time of 21.5 ± 9.1 hours with up to 48 hours elapsing before cell isolation. In most previous studies, the cardiac progenitor cells (a) (b) (c) Figure 4. Differentiation into mesenchymal lineages. Differentiation into osteoblasts, adipocytes and condroblasts was induced in TAD and ALPL+ cells over a period of 21 days. Phase contrast micros- copy images were obtained (a), and cytochemistry evaluations (b) carried out. Gene expression data are shown for the differentiation markers assayed by RT-qPCR (c). Data were collected from 3 donors. Representative data are shown. The calibration bar corresponds to 15 µm for figure a. The calibration bar for adipogenesis in figure b is 15 µm, and that for osteogenesis and chondrogenesis in figure b is 60 µm. Control (C) and induced (I). Fatty acid binding protein 4, adipocyte (Fabp4), collagen 1a (Col 1A) and collagen II (COL II), *p < 0.05. Openly accessible at
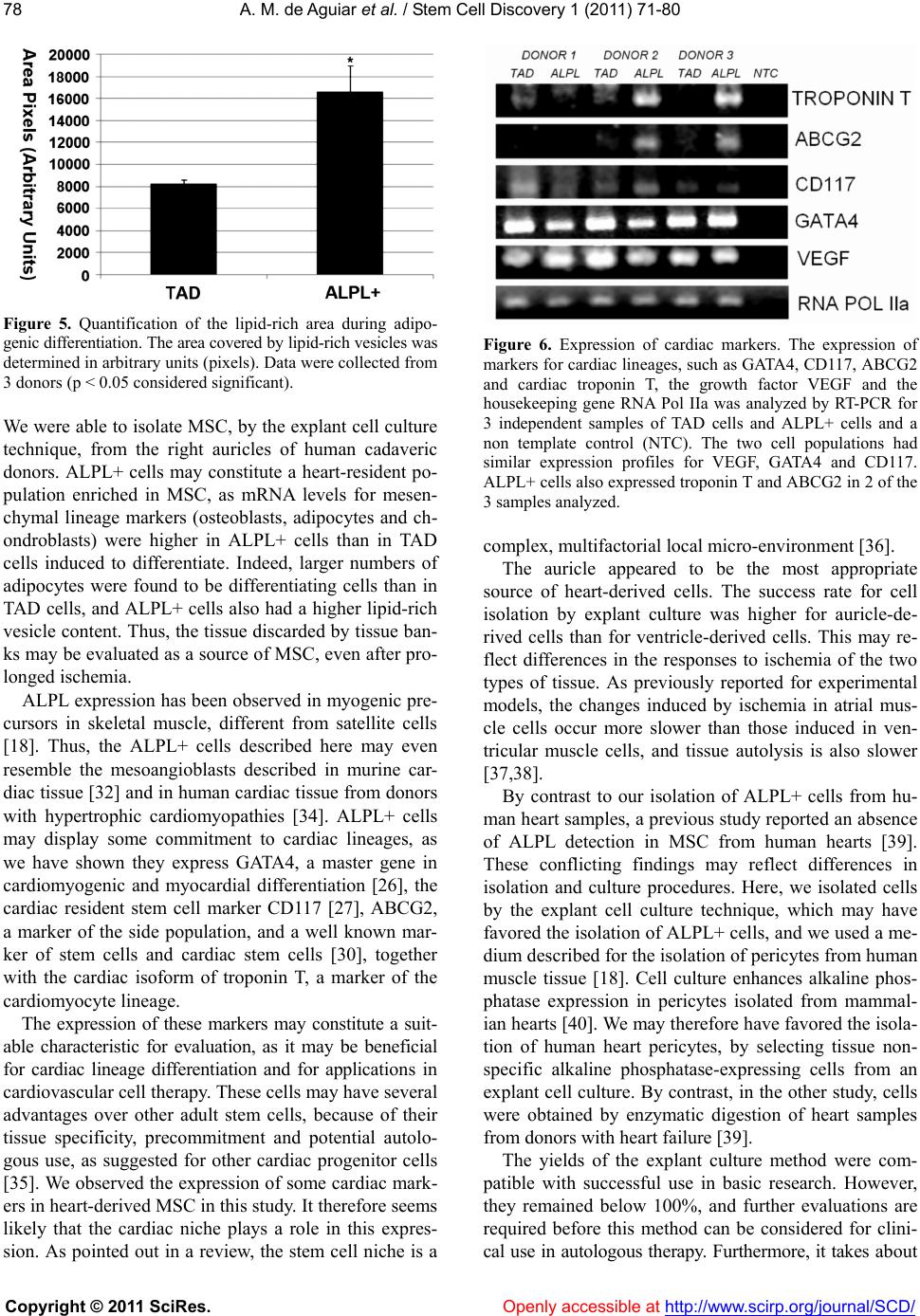 A. M. de Aguiar et al. / Stem Cell Discovery 1 (2011) 71-80 Copyright © 2011 SciRes. Openl y accessible at http://www.scirp.org/journal/SCD/ 78 Figure 5. Quantification of the lipid-rich area during adipo- genic differentiation. The area covered by lipid-rich vesicles was determined in arbitrary units (pixels). Data were collected from 3 donors (p < 0.05 considered significant). We were able to isolate MSC, by the exp lant cell culture technique, from the right auricles of human cadaveric donors. ALPL+ cells may constitute a heart-resident po- pulation enriched in MSC, as mRNA levels for mesen- chymal lineage markers (osteoblasts, adipocytes and ch- ondroblasts) were higher in ALPL+ cells than in TAD cells induced to differentiate. Indeed, larger numbers of adipocytes were found to be differentiating cells than in TAD cells, and ALPL+ cells also had a higher lipid-rich vesicle content. Thus, the tissue discarded by tissue ban- ks may be evaluated as a source of MSC, even after pro- longed ischemia. ALPL expression has been observed in myogenic pre- cursors in skeletal muscle, different from satellite cells [18]. Thus, the ALPL+ cells described here may even resemble the mesoangioblasts described in murine car- diac tissue [32] and in human cardiac tissue from donors with hypertrophic cardiomyopathies [34]. ALPL+ cells may display some commitment to cardiac lineages, as we have shown they express GATA4, a master gene in cardiomyogenic and myocardial differentiation [26], the cardiac resident stem cell marker CD117 [27], ABCG2, a marker of the side population, and a well known mar- ker of stem cells and cardiac stem cells [30], together with the cardiac isoform of troponin T, a marker of the cardiomyocyte lineage. The expression of these markers may constitute a suit- able characteristic for evaluation, as it may be beneficial for cardiac lineage differentiation and for applications in cardiovascular cell therapy. These cells may have several advantages over other adult stem cells, because of their tissue specificity, precommitment and potential autolo- gous use, as suggested for other cardiac progenitor cells [35]. We observed the expression of some cardiac mark- ers in heart-derived MSC in this study. It therefore seems likely that the cardiac niche plays a role in this expres- sion. As pointed out in a review, the stem cell niche is a Figure 6. Expression of cardiac markers. The expression of markers for cardiac lineages, such as GATA4, CD117, ABCG2 and cardiac troponin T, the growth factor VEGF and the housekeeping gene RNA Pol IIa was analyzed by RT-PCR for 3 independent samples of TAD cells and ALPL+ cells and a non template control (NTC). The two cell populations had similar expression profiles for VEGF, GATA4 and CD117. ALPL+ cells also expressed troponin T and ABCG2 in 2 of the 3 samples analyzed. complex, multifactorial local micro-environment [36]. The auricle appeared to be the most appropriate source of heart-derived cells. The success rate for cell isolation by explant culture was higher for auricle-de- rived cells than for ventricle-derived cells. This may re- flect differences in the responses to ischemia of the two types of tissue. As previously reported for experimental models, the changes induced by ischemia in atrial mus- cle cells occur more slower than those induced in ven- tricular muscle cells, and tissue autolysis is also slower [37,38]. By contrast to our isolation of ALPL+ cells from hu- man heart samples, a previous study reported an absence of ALPL detection in MSC from human hearts [39]. These conflicting findings may reflect differences in isolation and culture procedures. Here, we isolated cells by the explant cell culture technique, which may have favored the isolation of ALPL+ cells, and we used a me- dium described for the isolation of pericytes from human muscle tissue [18]. Cell culture enhances alkaline phos- phatase expression in pericytes isolated from mammal- ian hearts [40]. We may therefore have favored the isola- tion of human heart pericytes, by selecting tissue non- specific alkaline phosphatase-expressing cells from an explant cell culture. By contrast, in the other study, cells were obtained by enzymatic digestion of heart samples from donors with heart failure [39]. The yields of the explant culture method were com- patible with successful use in basic research. However, they remained below 100%, and further evaluations are required before this method can be considered for clini- cal use in autologous therapy. Furthermore, it takes about
 A. M. de Aguiar et al. / Stem Cell Discovery 1 (2011) 71-80 Copyright © 2011 SciRes. Openl y accessible at http://www.scirp.org/journal/SCD/ 7979 two to four weeks to isolate the first cells and, as re- ported here, yield varies with donor age. However, the explant cell culture method is simple, inexpensive and could be easily adapted for cell isolation from material provided by tissue banks, for obtaining heart-derived cells. In summary, tissue and cell banks now provide hope for improvements in the quality of life of people in need of cell or tissue transplantation. In the future, allogeneic cell banks for cell therapy should be evaluated, as pro- posed by Pasquinelli et al. [41]. If a heart-derived cell bank could be established, this might make it easier to obtain material for cell-based therapies on the basis of a histocompatibility match. This work provides new in- sight into possible sources and strategies for obtaining cells and making them available for cell therapy. 5. ACKNOWLEDGEMENTS We would like to thank Alyne Rocha Trigo, Andressa Vaz Schittini, Jaiesa Zych, Nilson Fidencio and Patrícia Shigunov for technical sup- port. We also thank all the staff of Instituto Carlos Chagas-Fiocruz Paraná for laboratory and administrative support and the staff of Banco de Homoexertos Cardíacos Humanos for tissue collection. We thank Itamar Crispin for graphic design. We thank Hugo Naya from Pasteur Institute, Montevideo for statistical analysis. This study was supported by Fundação Oswaldo Cruz, PAPES V (Grant number 0232 to AC), Decit/SCTIE/MS, by CNPq and Fundação Araucária (Grant number 202/2010 to SG), SETI/Fundação Araucária (Grant number 480/2010 to AMA). AC holds a Finep/CNPq fellowship, SG and BD are research fellows at the Conselho Nacional de Desenvolvimento Ci- entifico e Tecnológico-CNPq. The authors report no potential conflicts of interest or financial interests. REFERENCES [1] Gimble, J. and Guilak, F. (2003) Differentiation potential of adipose derived adult stem (ADAS) cells. Current Topics in Developmental Biology, 58, 137-160. doi:10.1016/S0070-2153(03)58005-X [2] Rebelatto, C., et al. (2008) Dissimilar differentiation of mesenchymal stem cells from bone marrow, umbilical cord blood, and adipose tissue . Experimental Biology and Medicine, 233, 901-913. doi:10.3181/0712-RM-356 [3] Makino, S., et al. (1999) Cardiomyocytes can be gen- erated from marrow stromal cells in vitro. Journal of Clinical Investigation, 103, 697-705. doi:10.1172/JCI5298 [4] Tomita, Y., et al. (2007) Application of mesenchymal stem cell-derived cardiomyocytes as bio-pacemakers: Current status and problems to be solved. Medical and Biological Engineering & Computing, 45, 209-220. doi:10.1007/s11517-007-0163-4 [5] Toma , C., Pi tte nger, M., Cahill, K., Byrne, B. and Kessle r, P. (2002) Human mesenchymal stem cells differentiate to a cardiomyocyte phenotype in the adult murine heart. Circulation, 105, 93-98. doi:10.1161/hc0102.101442 [6] Pittenger, M., et al. (1999) Multilineage potential of adult human mesenchymal stem cells. Science, 284, 143-147. doi:10.1126/science.284.5411.143 [7] Zuk, P., et al. (2002) Human adipose tissue is a source of multipotent stem cells. Molecular Biology of the Cell, 13, 4279-4295. doi:10.1091/mbc.E02-02-0105 [8] Rogers, I. and Casper, R. (2004) Umbilical cord blood stem cells. Best Practice and Research Clinical Obstet- rics and Gynaecolog, 18, 893-908. doi:10.1016/j.bpobgyn.2004.06.004 [9] Beltrami, A., et al. (2007) Multipotent cells can be gen- erated in vitro from several adult human organs (heart, liver, and bone ma rro w) . Blood, 110, 3438-3446. doi:10.1182/blood-2006-11-055566 [10] Crisan, M., et al. (2008) A perivascular origin for me- senchymal stem cells in multiple human organs. Cell Stem Cell, 3, 301-313. doi:10.1016/j.stem.2008.07.003 [11] Dominici, M., et al. (2006) Minimal criteria for defining multipotent mesenchymal stromal cells. The International Society for Cellular Therapy position statement. Cyto- therapy, 8, 315-317. doi:10.1080/14653240600855905 [12] Battula, V., et al. (2009) Isolation of functionally distinct mesenchymal stem cell subsets using antibodies against CD56, CD271, and mesenchymal stem cell antigen-1. Haematologica, 94, 173-184. doi:10.3324/haematol.13740 [13] Sobiesiak, M., et al. (2009) The mesenchymal stem cell antigen MSCA-1 is identical to tissue non-specific alka- line phosphatase. Stem Cells and Development, 19, 669- 677. doi:10.1089/scd.2009.0290 [14] Mornet, E., et al. (2001) Structural evidence for a func- tional role of human tissue nonspecific alkaline phos- phatase in bone mineralization. Journal of Biological Che- mistry, 276, 31171-31178. doi:10.1074/jbc.M102788200 [15] O'Connor, M., et al. (2008) Alkaline phosphatase-posi- tive colony formation is a sensitive, specific, and quanti- tative indicator of undifferentiated human embryonic stem cells. Stem Cells, 26, 1109-1116. doi:10.1634/stemcells.2007-0801 [16] Adewumi, O. et al. (2007) Characterization of human embryonic stem cell lines by the International Stem Cell Initiative. Nature Biotechnology, 25, 803-816. doi:10.1038/nbt1318 [17] Langer, D., Ikehara, Y., Takebayashi, H., Hawkes, R. and Zimmermann, H. (2007) The ectonucleotidases alkaline phosphatase and nucleoside triphosphate diphosphohy- drolase 2 are associated with subsets of progenitor cell populations in the mouse embryonic, postnatal and adult neurogenic zones. Neuroscience, 150, 863-879. doi:10.1016/j.neuroscience.2007.07.064 [18] Dellavalle, A., et al. (2007) Pericytes of human skeletal muscle are myogenic precursors distinct from satellite cells. Nature Cell Biology, 9, 255-267. doi:10.1038/ncb1542 [19] Schwab, K. and Gargett, C. (2008) Co-expression of two perivascular cell markers isolates mesenchymal stem-like cells from human e ndometri um. Human Reproduction, 22, 2903-291 1. doi:10.1093/humrep/dem265 [20] Crisan, M., Corselli, M., Chen, C.W. and Péault, B. (2011) Multilineage stem cells in the adult: A perivascular leg- acy? Organogenesis, 7, 101-104.
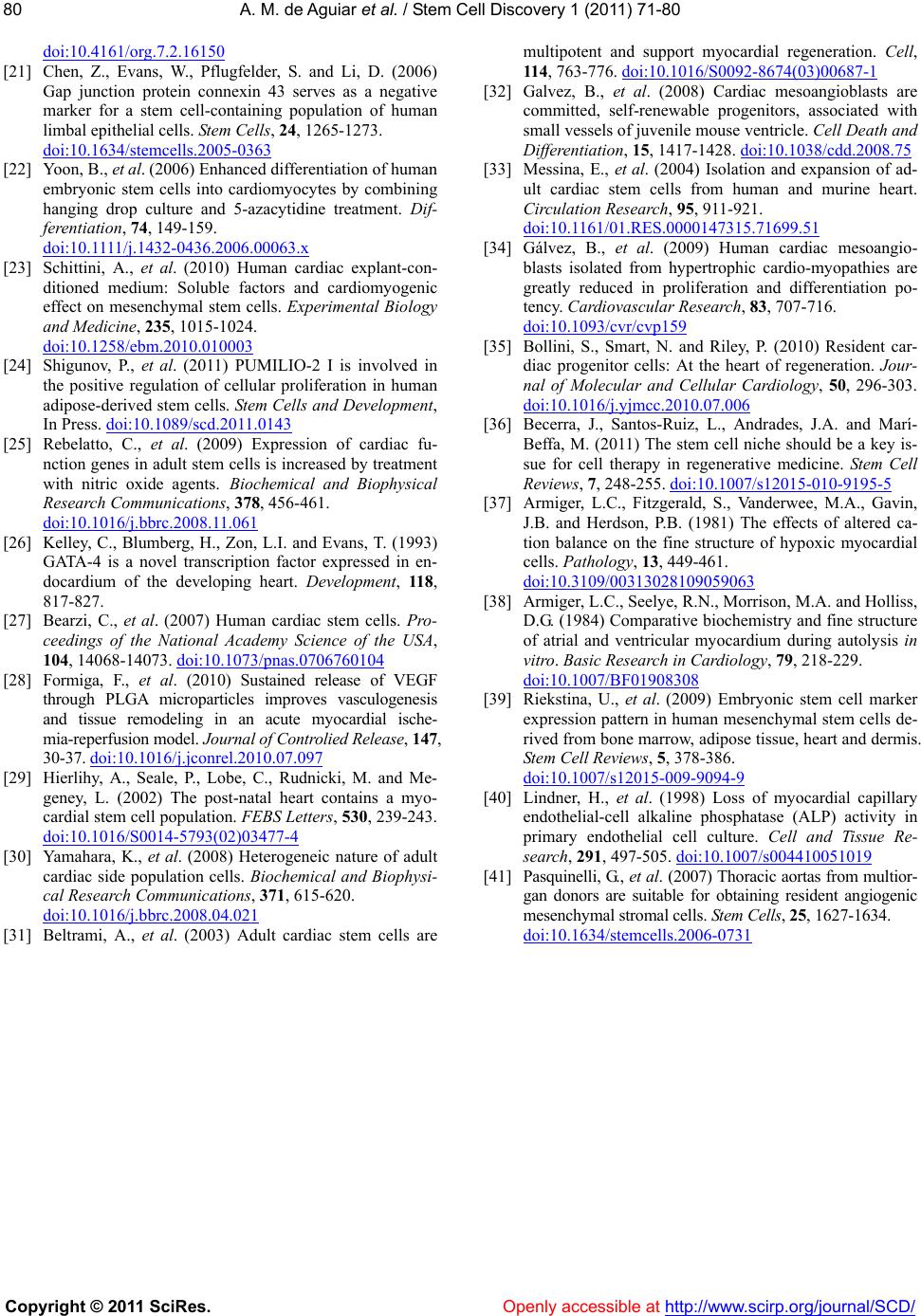 A. M. de Aguiar et al. / Stem Cell Discovery 1 (2011) 71-80 Copyright © 2011 SciRes. http://www.scirp.org/journal/SCD/Openly accessible at 80 doi:10.4161/org.7.2.16150 [21] Chen, Z., Evans, W., Pflugfelder, S. and Li, D. (2006) Gap junction protein connexin 43 serves as a negative marker for a stem cell-containing population of human limbal epithelial cells. Stem Cells, 24, 1265-1273. doi:10.1634/stemcells.2005-0363 [22] Yoon, B., et al. (2006) Enhanced differentiation of human embryonic stem cells into cardiomyocytes by combining hanging drop culture and 5-azacytidine treatment. Dif- ferentiation, 74, 149-159. doi:10.1111/j.1432-0436.2006.00063.x [23] Schittini, A., et al. (2010) Human cardiac explant-con- ditioned medium: Soluble factors and cardiomyogenic effect on mesenchymal stem cells. Experimental Biology and Medicine, 235, 1015-1024. doi:10.1258/ebm.2010.010003 [24] Shigunov, P., et al. (2011) PUMILIO-2 I is involved in the positive regulation of cellular proliferation in human adipose-derived stem cells. Stem Cells and Development, In Press. doi:10.1089/scd.2011.0143 [25] Rebelatto, C., et al. (2009) Expression of cardiac fu- nction genes in adult stem cells is increased by treatment with nitric oxide agents. Biochemical and Biophysical Research Communications, 378, 45 6- 461 . doi:10.1016/j.bbrc.2008.11.061 [26] Kelley, C., Blumberg, H., Zon, L.I. and Evans, T. (1993) GATA-4 is a novel transcription factor expressed in en- docardium of the developing heart. Development, 11 8, 817-827. [27] Bearzi, C., et al. (2007) Human cardiac stem cells. Pro- ceedings of the National Academy Science of the USA, 104, 14068-14073. doi:10.1073/pnas.0706760104 [28] Formiga, F., et al. (2010) Sustained release of VEGF through PLGA microparticles improves vasculogenesis and tissue remodeling in an acute myocardial ische- mia-reperfusion mo del. Journal of Controlied Release, 147, 30-37. doi:10.1016/j.jconrel.2010.07.097 [29] Hierlihy, A., Seale, P., Lobe, C., Rudnicki, M. and Me- geney, L. (2002) The post-natal heart contains a myo- cardial stem cell population. FEBS Letters, 530, 239-243. doi:10.1016/S0014-5793(02)03477-4 [30] Yamahara, K., et al. (2008) Heterogeneic nature of adult cardiac side population cells. Biochemical and Biophysi- cal Research Communications, 371, 615-620. doi:10.1016/j.bbrc.2008.04.021 [31] Beltrami, A., et al. (2003) Adult cardiac stem cells are multipotent and support myocardial regeneration. Cell, 114, 763-776. doi:10.1016/S0092-8674(03)00687-1 [32] Galvez, B., et al. (2008) Cardiac mesoangioblasts are committed, self-renewable progenitors, associated with small vessels of juvenile mouse ventricle. Cell Death and Differentiation, 15, 1417-1428. doi:10.1038/cdd.2008.75 [33] Messina, E., et al. (2004) Isolation and expansion of ad- ult cardiac stem cells from human and murine heart. Circulation Research, 95, 911-921. doi:10.1161/01.RES.0000147315.71699.51 [34] Gálvez, B., et al. (2009) Human cardiac mesoangio- blasts isolated from hypertrophic cardio-myopathies are greatly reduced in proliferation and differentiation po- tency. Cardiovascular Research, 83, 707-716. doi:10.1093/cvr/cvp159 [35] Bollini, S., Smart, N. and Riley, P. (2010) Resident car- diac progenitor cells: At the heart of regeneration. Jour- nal of Molecular and Cellular Cardiology, 50, 296-303. doi:10.1016/j.yjmcc.2010.07.006 [36] Becerra, J., Santos-Ruiz, L., Andrades, J.A. and Marí- Beffa, M. (2011) The stem cell niche should be a key is- sue for cell therapy in regenerative medicine. Stem Cell Reviews, 7, 248-255. doi:10.1007/s12015-010-9195-5 [37] Armiger, L.C., Fitzgerald, S., Vanderwee, M.A., Gavin, J.B. and Herdson, P.B. (1981) The effects of altered ca- tion balance on the fine structure of hypoxic myocardial cells. Pathology, 13, 449-461. doi:10.3109/00313028109059063 [38] Armiger, L.C., Seelye, R.N. , Morrison, M.A. and Holliss, D.G. (1984) Comparative biochemistry and fine structure of atrial and ventricular myocardium during autolysis in vitro. Basic Research in Cardiology, 79, 218-229. doi:10.1007/BF01908308 [39] Riekstina, U., et al. (2009) Embryonic stem cell marker expression pattern in human mesenchymal stem cells de- rived from bone marrow, adipose tissue, heart and dermis. Stem Cell Reviews, 5, 378-386. doi:10.1007/s12015-009-9094-9 [40] Lindner, H., et al. (1998) Loss of myocardial capillary endothelial-cell alkaline phosphatase (ALP) activity in primary endothelial cell culture. Cell and Tissue Re- search, 291, 497-505. doi:10.1007/s004410051019 [41] Pasquinelli, G., et al. (2007) Thoracic aortas from multior- gan donors are suitable for obtaining resident angiogenic mesenchymal stromal cells. Stem Cells, 25 , 1627-1634. doi:10.1634/stemcells.2006-0731
|