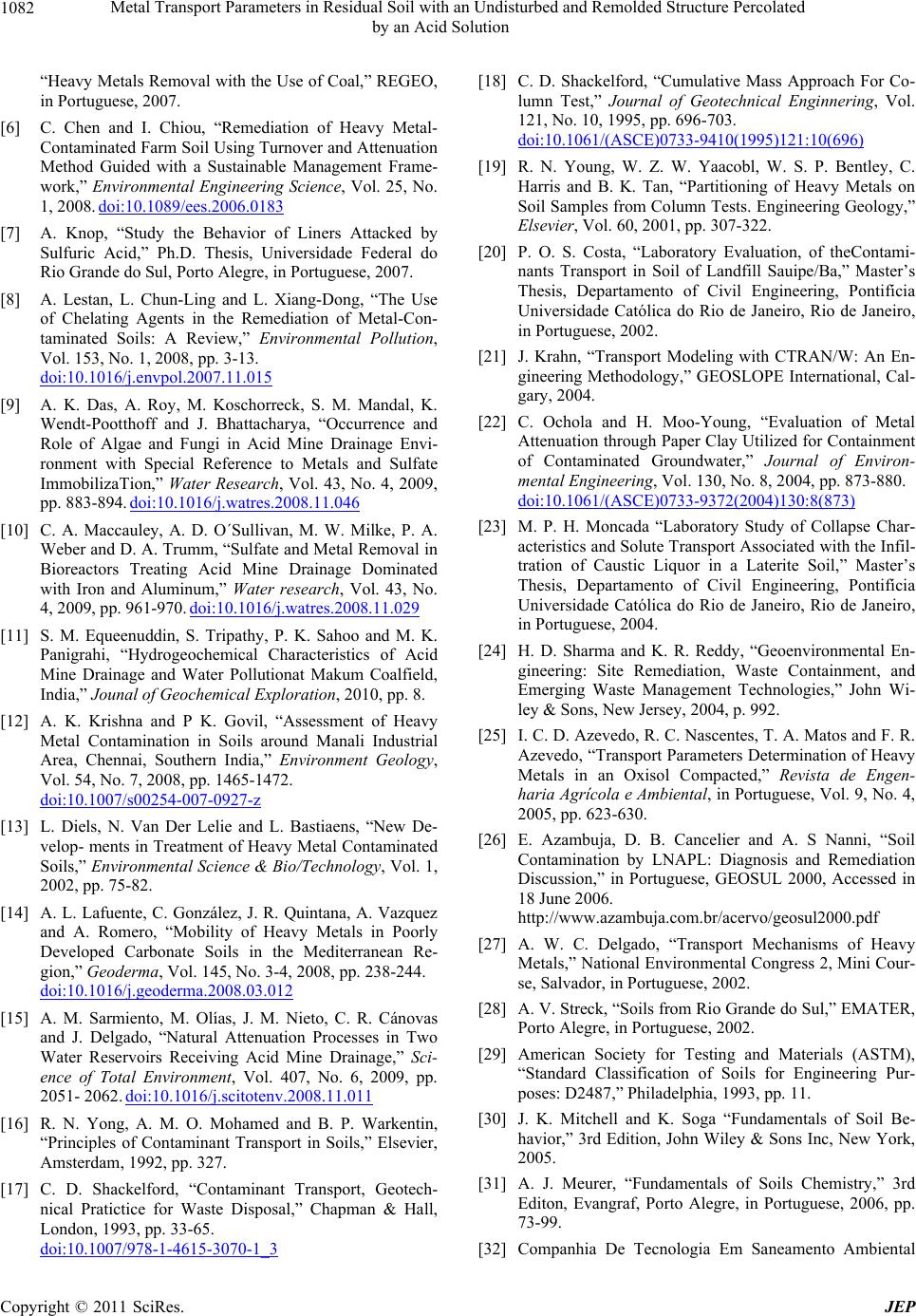
Metal Transport Parameters in Residual Soil with an Undisturbed and Remolded Structure Percolated
1082
by an Acid Solution
“Heavy Metals Removal with the Use of Coal,” REGEO,
in Portuguese, 2007.
[6] C. Chen and I. Chiou, “Remediation of Heavy Metal-
Contaminated Farm Soil Using Turnover and Attenuation
Method Guided with a Sustainable Management Frame-
work,” Environmental Engineering Science, Vol. 25, No.
1, 2008. doi:10.1089/ees.2006.0183
[7] A. Knop, “Study the Behavior of Liners Attacked by
Sulfuric Acid,” Ph.D. Thesis, Universidade Federal do
Rio Grande do Sul, Porto Alegre, in Portuguese, 2007.
[8] A. Lestan, L. Chun-Ling and L. Xiang-Dong, “The Use
of Chelating Agents in the Remediation of Metal-Con-
taminated Soils: A Review,” Environmental Pollution,
Vol. 153, No. 1, 2008, pp. 3-13.
doi:10.1016/j.envpol.2007.11.015
[9] A. K. Das, A. Roy, M. Koschorreck, S. M. Mandal, K.
Wendt-Pootthoff and J. Bhattacharya, “Occurrence and
Role of Algae and Fungi in Acid Mine Drainage Envi-
ronment with Special Reference to Metals and Sulfate
ImmobilizaTion,” Water Re search, Vol. 43, No. 4, 2009,
pp. 883-894. doi:10.1016/j.watres.2008.11.046
[10] C. A. Maccauley, A. D. O´Sullivan, M. W. Milke, P. A.
Weber and D. A. Trumm, “Sulfate and Metal Removal in
Bioreactors Treating Acid Mine Drainage Dominated
with Iron and Aluminum,” Water research, Vol. 43, No.
4, 2009, pp. 961-970. doi:10.1016/j.watres.2008.11.029
[11] S. M. Equeenuddin, S. Tripathy, P. K. Sahoo and M. K.
Panigrahi, “Hydrogeochemical Characteristics of Acid
Mine Drainage and Water Pollutionat Makum Coalfield,
India,” Jounal of Geochemical Exploration, 2010, pp. 8.
[12] A. K. Krishna and P K. Govil, “Assessment of Heavy
Metal Contamination in Soils around Manali Industrial
Area, Chennai, Southern India,” Environment Geology,
Vol. 54, No. 7, 2008, pp. 1465-1472.
doi:10.1007/s00254-007-0927-z
[13] L. Diels, N. Van Der Lelie and L. Bastiaens, “New De-
velop- ments in Treatment of Heavy Metal Contaminated
Soils,” Environmental Science & Bio/Technology, Vol. 1,
2002, pp. 75-82.
[14] A. L. Lafuente, C. González, J. R. Quintana, A. Vazquez
and A. Romero, “Mobility of Heavy Metals in Poorly
Developed Carbonate Soils in the Mediterranean Re-
gion,” Geoderma, Vol. 145, No. 3-4, 2008, pp. 238-244.
doi:10.1016/j.geoderma.2008.03.012
[15] A. M. Sarmiento, M. Olías, J. M. Nieto, C. R. Cánovas
and J. Delgado, “Natural Attenuation Processes in Two
Water Reservoirs Receiving Acid Mine Drainage,” Sci-
ence of Total Environment, Vol. 407, No. 6, 2009, pp.
2051- 2062. doi:10.1016/j.scitotenv.2008.11.011
[16] R. N. Yong, A. M. O. Mohamed and B. P. Warkentin,
“Principles of Contaminant Transport in Soils,” Elsevier,
Amsterdam, 1992, pp. 327.
[17] C. D. Shackelford, “Contaminant Transport, Geotech-
nical Pratictice for Waste Disposal,” Chapman & Hall,
London, 1993, pp. 33-65.
doi:10.1007/978-1-4615-3070-1_3
[18] C. D. Shackelford, “Cumulative Mass Approach For Co-
lumn Test,” Journal of Geotechnical Enginnering, Vol.
121, No. 10, 1995, pp. 696-703.
doi:10.1061/(ASCE)0733-9410(1995)121:10(696)
[19] R. N. Young, W. Z. W. Yaacobl, W. S. P. Bentley, C.
Harris and B. K. Tan, “Partitioning of Heavy Metals on
Soil Samples from Column Tests. Engineering Geology,”
Elsevier, Vol. 60, 2001, pp. 307-322.
[20] P. O. S. Costa, “Laboratory Evaluation, of theContami-
nants Transport in Soil of Landfill Sauipe/Ba,” Master’s
Thesis, Departamento of Civil Engineering, Pontifícia
Universidade Católica do Rio de Janeiro, Rio de Janeiro,
in Portuguese, 2002.
[21] J. Krahn, “Transport Modeling with CTRAN/W: An En-
gineering Methodology,” GEOSLOPE International, Cal-
gary, 2004.
[22] C. Ochola and H. Moo-Young, “Evaluation of Metal
Attenuation through Paper Clay Utilized for Containment
of Contaminated Groundwater,” Journal of Environ-
mental Engineer ing, Vol. 130, No. 8, 2004, pp. 873-880.
doi:10.1061/(ASCE)0733-9372(2004)130:8(873)
[23] M. P. H. Moncada “Laboratory Study of Collapse Char-
acteristics and Solute Transport Associated with the Infil-
tration of Caustic Liquor in a Laterite Soil,” Master’s
Thesis, Departamento of Civil Engineering, Pontifícia
Universidade Católica do Rio de Janeiro, Rio de Janeiro,
in Portuguese, 2004.
[24] H. D. Sharma and K. R. Reddy, “Geoenvironmental En-
gineering: Site Remediation, Waste Containment, and
Emerging Waste Management Technologies,” John Wi-
ley & Sons, New Jersey, 2004, p. 992.
[25] I. C. D. Azevedo, R. C. Nascentes, T. A. Matos and F. R.
Azevedo, “Transport Parameters Determination of Heavy
Metals in an Oxisol Compacted,” Revista de Engen-
haria Agrícola e Ambiental, in Portuguese, Vol. 9, No. 4,
2005, pp. 623-630.
[26] E. Azambuja, D. B. Cancelier and A. S Nanni, “Soil
Contamination by LNAPL: Diagnosis and Remediation
Discussion,” in Portuguese, GEOSUL 2000, Accessed in
18 June 2006.
http://www.azambuja.com.br/acervo/geosul2000.pdf
[27] A. W. C. Delgado, “Transport Mechanisms of Heavy
Metals,” National Environmental Congress 2, Mini Cour-
se, Salvador, in Portuguese, 2002.
[28] A. V. Streck, “Soils from Rio Grande do Sul,” EMATER,
Porto Alegre, in Portuguese, 2002.
[29] American Society for Testing and Materials (ASTM),
“Standard Classification of Soils for Engineering Pur-
poses: D2487,” Philadelphia, 1993, pp. 11.
[30] J. K. Mitchell and K. Soga “Fundamentals of Soil Be-
havior,” 3rd Edition, John Wiley & Sons Inc, New York,
2005.
[31] A. J. Meurer, “Fundamentals of Soils Chemistry,” 3rd
Editon, Evangraf, Porto Alegre, in Portuguese, 2006, pp.
73-99.
[32] Companhia De Tecnologia Em Saneamento Ambiental
C
opyright © 2011 SciRes. JEP