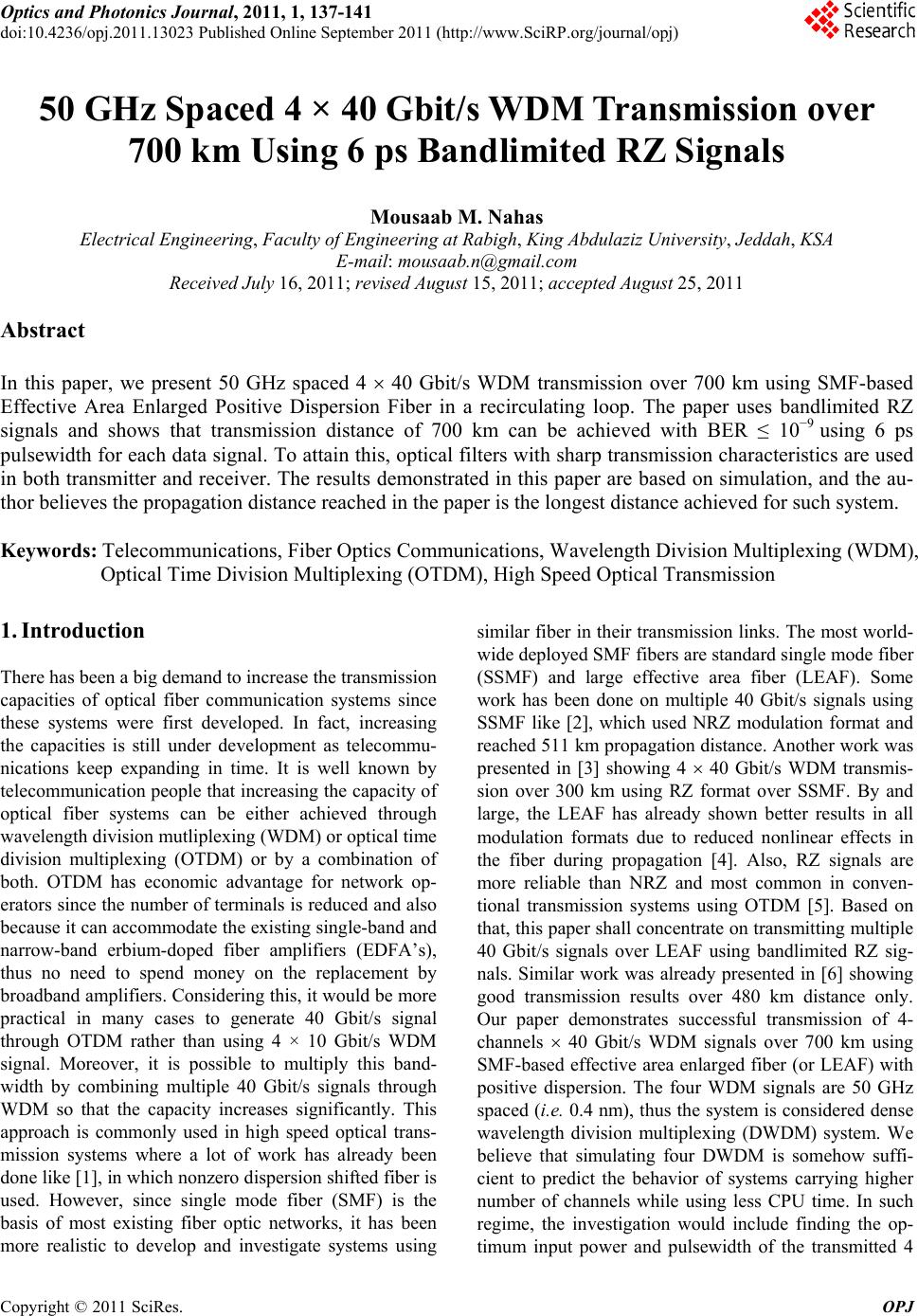
Optics and Photonics Journal, 2011, 1, 137-141
doi:10.4236/opj.2011.13023 Published Online September 2011 (http://www.SciRP.org/journal/opj)
Copyright © 2011 SciRes. OPJ
50 GHz Spaced 4 × 40 Gbit/s WDM Transmission over
700 km Using 6 ps Bandlimited RZ Signals
Mousaab M. Nahas
Electrical Engineering, Faculty of Engineering at Rabigh, King Abdulaziz University, Jeddah, KSA
E-mail: mousaab.n@gmail.com
Received July 16, 2011; revised August 15, 2011; accepted August 25, 2011
Abstract
In this paper, we present 50 GHz spaced 4 40 Gbit/s WDM transmission over 700 km using SMF-based
Effective Area Enlarged Positive Dispersion Fiber in a recirculating loop. The paper uses bandlimited RZ
signals and shows that transmission distance of 700 km can be achieved with BER ≤ 10−9 using 6 ps
pulsewidth for each data signal. To attain this, optical filters with sharp transmission characteristics are used
in both transmitter and receiver. The results demonstrated in this paper are based on simulation, and the au-
thor believes the propagation distance reached in the paper is the longest distance achieved for such system.
Keywords: Telecommunications, Fiber Optics Communications, Wavelength Division Multiplexing (WDM),
Optical Time Division Multiplexing (OTDM), High Speed Optical Transmission
1. Introduction
There has been a big demand to increase the transmission
capacities of optical fiber communication systems since
these systems were first developed. In fact, increasing
the capacities is still under development as telecommu-
nications keep expanding in time. It is well known by
telecommunication people that increasing the capacity of
optical fiber systems can be either achieved through
wavelength division mutliplexing (WDM) or optical time
division multiplexing (OTDM) or by a combination of
both. OTDM has economic advantage for network op-
erators since the number of terminals is reduced and also
because it can accommodate the existing single-band and
narrow-band erbium-doped fiber amplifiers (EDFA’s),
thus no need to spend money on the replacement by
broadband amplifiers. Considering this, it would be more
practical in many cases to generate 40 Gbit/s signal
through OTDM rather than using 4 × 10 Gbit/s WDM
signal. Moreover, it is possible to multiply this band-
width by combining multiple 40 Gbit/s signals through
WDM so that the capacity increases significantly. This
approach is commonly used in high speed optical trans-
mission systems where a lot of work has already been
done like [1], in which nonzero dispersion shifted fiber is
used. However, since single mode fiber (SMF) is the
basis of most existing fiber optic networks, it has been
more realistic to develop and investigate systems using
similar fiber in their transmission links. The most world-
wide deployed SMF fibers are standard single mode fiber
(SSMF) and large effective area fiber (LEAF). Some
work has been done on multiple 40 Gbit/s signals using
SSMF like [2], which used NRZ modulation format and
reached 511 km propagation distance. Another work was
presented in [3] showing 4 40 Gbit/s WDM transmis-
sion over 300 km using RZ format over SSMF. By and
large, the LEAF has already shown better results in all
modulation formats due to reduced nonlinear effects in
the fiber during propagation [4]. Also, RZ signals are
more reliable than NRZ and most common in conven-
tional transmission systems using OTDM [5]. Based on
that, this paper shall concentrate on transmitting multiple
40 Gbit/s signals over LEAF using bandlimited RZ sig-
nals. Similar work was already presented in [6] showing
good transmission results over 480 km distance only.
Our paper demonstrates successful transmission of 4-
channels 40 Gbit/s WDM signals over 700 km using
SMF-based effective area enlarged fiber (or LEAF) with
positive dispersion. The four WDM signals are 50 GHz
spaced (i.e. 0.4 nm), thus the system is considered dense
wavelength division multiplexing (DWDM) system. We
believe that simulating four DWDM is somehow suffi-
cient to predict the behavior of systems carrying higher
number of channels while using less CPU time. In such
regime, the investigation would include finding the op-
timum input power and pulsewidth of the transmitted 4