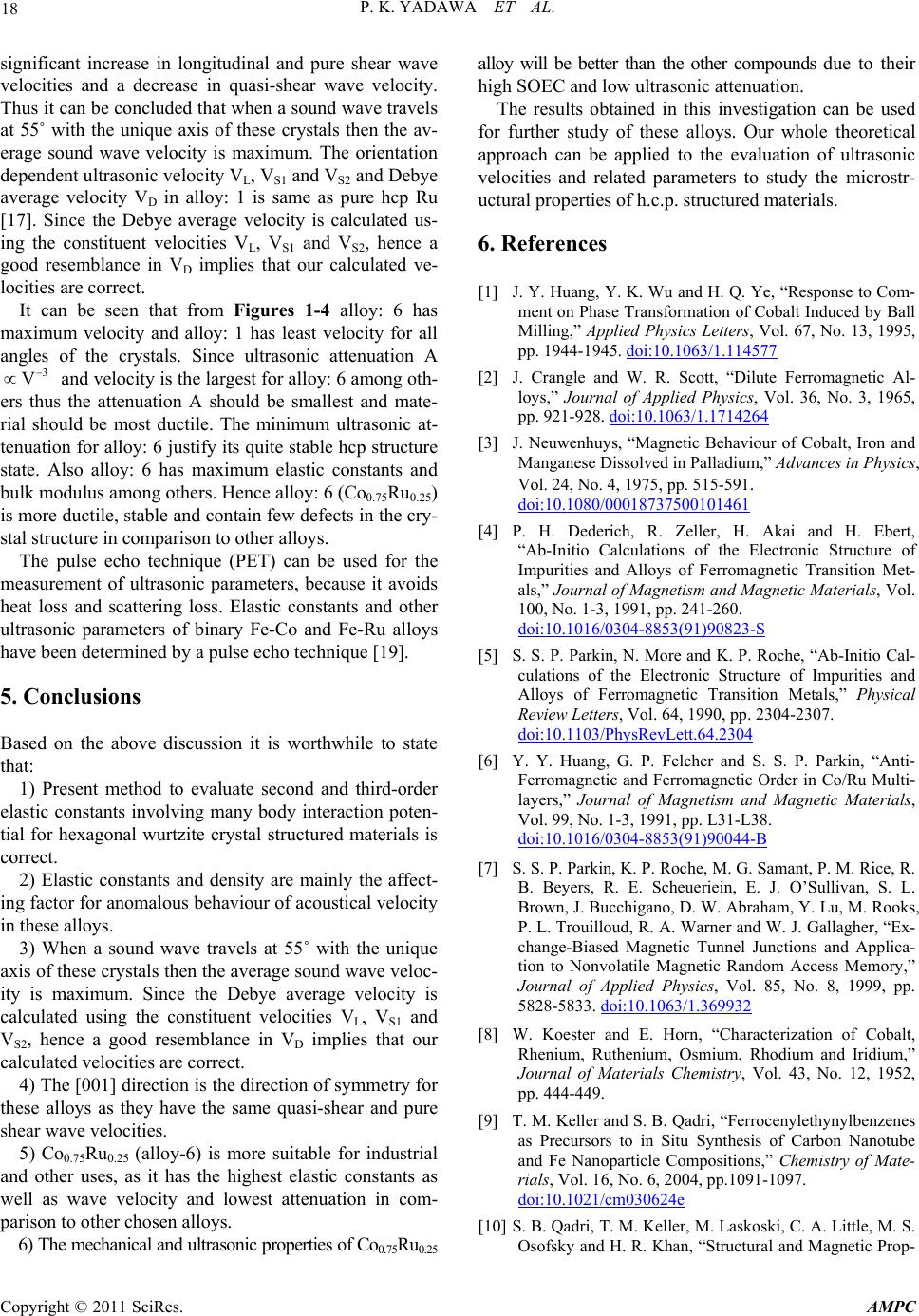
P. K. YADAWA ET AL.
18
significant increase in longitudinal and pure shear wave
velocities and a decrease in quasi-shear wave velocity.
Thus it can be concluded that when a sound wave travels
at 55˚ with the unique axis of these crystals then the av-
erage sound wave velocity is maximum. The orientation
dependent ultrasonic velocity VL, VS1 and VS2 and Debye
average velocity VD in alloy: 1 is same as pure hcp Ru
[17]. Since the Debye average velocity is calculated us-
ing the constituent velocities VL, VS1 and VS2, hence a
good resemblance in VD implies that our calculated ve-
locities are correct.
It can be seen that from Figures 1-4 alloy: 6 has
maximum velocity and alloy: 1 has least velocity for all
angles of the crystals. Since ultrasonic attenuation A
and velocity is the largest for alloy: 6 among oth-
ers thus the attenuation A should be smallest and mate-
rial should be most ductile. The minimum ultrasonic at-
tenuation for alloy: 6 justify its quite stable hcp structure
state. Also alloy: 6 has maximum elastic constants and
bulk modulus among others. Hence alloy: 6 (Co0.75Ru0.25)
is more ductile, stable and contain few defects in the cry-
stal structure in comparison to other alloys.
3
V
The pulse echo technique (PET) can be used for the
measurement of ultrasonic parameters, because it avoids
heat loss and scattering loss. Elastic constants and other
ultrasonic parameters of binary Fe-Co and Fe-Ru alloys
have been determined by a pulse echo technique [19].
5. Conclusions
Based on the above discussion it is worthwhile to state
that:
1) Present method to evaluate second and third-order
elastic constants involving many body interaction poten-
tial for hexagonal wurtzite crystal structured materials is
correct.
2) Elastic constants and density are mainly the affect-
ing factor for anomalous behaviour of acoustical velocity
in these alloys.
3) When a sound wave travels at 55˚ with the unique
axis of these crystals then the average sound wave veloc-
ity is maximum. Since the Debye average velocity is
calculated using the constituent velocities VL, VS1 and
VS2, hence a good resemblance in VD implies that our
calculated velocities are correct.
4) The [001] direction is the direction of symmetry for
these alloys as they have the same quasi-shear and pure
shear wave velocities.
5) Co0.75Ru0.25 (alloy-6) is more suitable for industrial
and other uses, as it has the highest elastic constants as
well as wave velocity and lowest attenuation in com-
parison to other chosen alloys.
6) The mechanical and ultrasonic properties of Co0.75Ru0.25
alloy will be better than the other compounds due to their
high SOEC and low ultrasonic attenuation.
The results obtained in this investigation can be used
for further study of these alloys. Our whole theoretical
approach can be applied to the evaluation of ultrasonic
velocities and related parameters to study the microstr-
uctural properties of h.c.p. structured materials.
6. References
[1] J. Y. Huang, Y. K. Wu and H. Q. Ye, “Response to Com-
ment on Phase Transformation of Cobalt Induced by Ball
Milling,” Applied Physics Letters, Vol. 67, No. 13, 1995,
pp. 1944-1945. doi:10.1063/1.114577
[2] J. Crangle and W. R. Scott, “Dilute Ferromagnetic Al-
loys,” Journal of Applied Physics, Vol. 36, No. 3, 1965,
pp. 921-928. doi:10.1063/1.1714264
[3] J. Neuwenhuys, “Magnetic Behaviour of Cobalt, Iron and
Manganese Dissolved in Palladium, ” Advances in Physics,
Vol. 24, No. 4, 1975, pp. 515-591.
doi:10.1080/00018737500101461
[4] P. H. Dederich, R. Zeller, H. Akai and H. Ebert,
“Ab-Initio Calculations of the Electronic Structure of
Impurities and Alloys of Ferromagnetic Transition Met-
als,” Journal of Magnetism and Magnetic Materials, Vol.
100, No. 1-3, 1991, pp. 241-260.
doi:10.1016/0304-8853(91)90823-S
[5] S. S. P. Parkin, N. More and K. P. Roche, “Ab-Initio Cal-
culations of the Electronic Structure of Impurities and
Alloys of Ferromagnetic Transition Metals,” Physical
Review Letters, Vol. 64, 1990, pp. 2304-2307.
doi:10.1103/PhysRevLett.64.2304
[6] Y. Y. Huang, G. P. Felcher and S. S. P. Parkin, “Anti-
Ferromagnetic and Ferromagnetic Order in Co/Ru Multi-
layers,” Journal of Magnetism and Magnetic Materials,
Vol. 99, No. 1-3, 1991, pp. L31-L38.
doi:10.1016/0304-8853(91)90044-B
[7] S. S. P. Parkin, K. P. Roche, M. G. Sa mant, P. M. Ric e, R.
B. Beyers, R. E. Scheueriein, E. J. O’Sullivan, S. L.
Brown, J. Bucchigano, D. W. Abraham, Y. Lu, M. Rooks,
P. L. Trouilloud, R. A. Warner and W. J. Gallagher, “Ex-
change-Biased Magnetic Tunnel Junctions and Applica-
tion to Nonvolatile Magnetic Random Access Memory,”
Journal of Applied Physics, Vol. 85, No. 8, 1999, pp.
5828-5833. doi:10.1063/1.369932
[8] W. Koester and E. Horn, “Characterization of Cobalt,
Rhenium, Ruthenium, Osmium, Rhodium and Iridium,”
Journal of Materials Chemistry, Vol. 43, No. 12, 1952,
pp. 444-449.
[9] T. M. Keller and S. B. Qadri, “Ferrocenylethynylbenzenes
as Precursors to in Situ Synthesis of Carbon Nanotube
and Fe Nanoparticle Compositions,” Chemistry of Mate-
rials, Vol. 16, No. 6, 2004, pp.1091-1097.
doi:10.1021/cm030624e
[10] S. B. Qadri, T. M. Keller, M. Laskoski, C. A. Little, M. S.
Osofsky and H. R. Khan, “Structural and Magnetic Prop-
Copyright © 2011 SciRes. AMPC