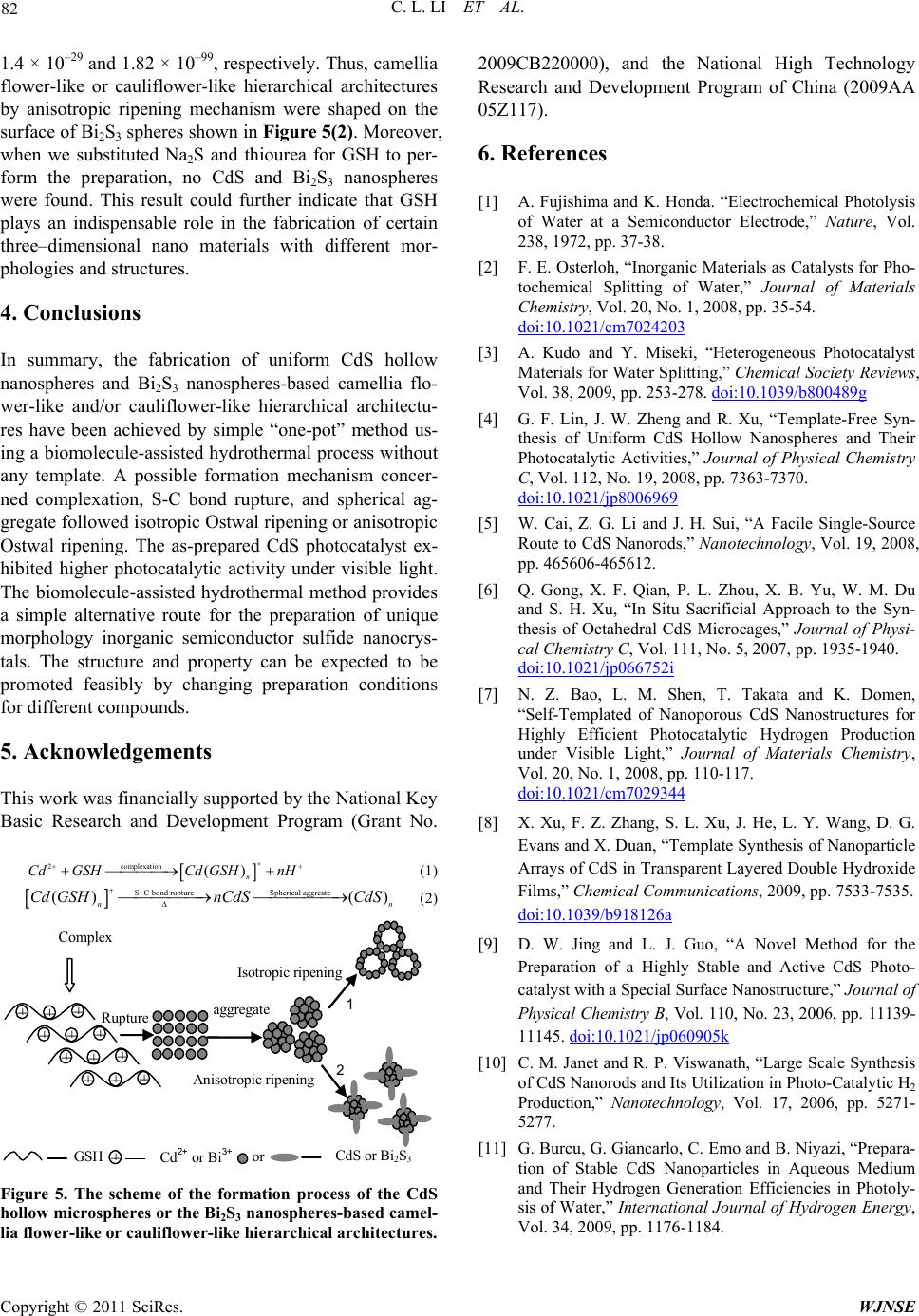
C. L. LI ET AL.
82
1.4 × 10–29 and 1.82 × 10–99, respectively. Thus, camellia
flower-like or cauliflower-like hierarchical architectures
by anisotropic ripening mechanism were shaped on the
surface of Bi2S3 spheres shown in Figure 5(2). Moreover,
when we substituted Na2S and thiourea for GSH to per-
form the preparation, no CdS and Bi2S3 nanospheres
were found. This result could further indicate that GSH
plays an indispensable role in the fabrication of certain
three–dimensional nano materials with different mor-
phologies and structures.
4. Conclusions
In summary, the fabrication of uniform CdS hollow
nanospheres and Bi2S3 nanospheres-based camellia flo-
wer-like and/or cauliflower-like hierarchical architectu-
res have been achieved by simple “one-pot” method us-
ing a biomolecule-assisted hydrothermal process without
any template. A possible formation mechanism concer-
ned complexation, S-C bond rupture, and spherical ag-
gregate followed isotropic Ostwal ripening or anisotropic
Ostwal ripening. The as-prepared CdS photocatalyst ex-
hibited higher photocatalytic activity under visible light.
The biomolecule-assisted hydrothermal method provides
a simple alternative route for the preparation of unique
morphology inorganic semiconductor sulfide nanocrys-
tals. The structure and property can be expected to be
promoted feasibly by changing preparation conditions
for different compounds.
5. Acknowledgements
This work was financially supported by the National Key
Basic Research and Development Program (Grant No.
2complexation ()
n
CdGSHCd GSHnH
SC bond ruptureSpherical
(1)
aggreate
() ()
n n
Cd GSHnCdSCdS
(2)
Rupture
Complex
aggregate
Isotropic ripening
Anisotropic ripening
1
2
Cd2+ or Bi3+ CdS or Bi2S3
or
GSH
Figure 5. The scheme of the formation process of the CdS
hollow microspheres or the Bi2S3 nanospheres-based camel-
lia flower-like or cauliflower-like hierarchical architectures.
2009CB220000), and the National High Technology
Research and Development Program of China (2009AA
05Z117).
6. References
[1] A. Fujishima and K. Honda. “Electrochemical Photolysis
of Water at a Semiconductor Electrode,” Nature, Vol.
238, 1972, pp. 37-38.
[2] F. E. Osterloh, “Inorganic Materials as Catalysts for Pho-
tochemical Splitting of Water,” Journal of Materials
Chemistry, Vol. 20, No. 1, 2008, pp. 35-54.
doi:10.1021/cm7024203
[3] A. Kudo and Y. Miseki, “Heterogeneous Photocatalyst
Materials for Water Splitting,” Chemical Society Reviews,
Vol. 38, 2009, pp. 253-278. doi:10.1039/b800489g
[4] G. F. Lin, J. W. Zheng and R. Xu, “Template-Free Syn-
thesis of Uniform CdS Hollow Nanospheres and Their
Photocatalytic Activities,” Journal of Physical Chemistry
C, Vol. 112, No. 19, 2008, pp. 7363-7370.
doi:10.1021/jp8006969
[5] W. Cai, Z. G. Li and J. H. Sui, “A Facile Single-Source
Route to CdS Nanorods,” Nanotechnology, Vol. 19, 2008,
pp. 465606-465612.
[6] Q. Gong, X. F. Qian, P. L. Zhou, X. B. Yu, W. M. Du
and S. H. Xu, “In Situ Sacrificial Approach to the Syn-
thesis of Octahedral CdS Microcages,” Journal of Physi-
cal Chemistry C, Vol. 111, No. 5, 2007, pp. 1935-1940.
doi:10.1021/jp066752i
[7] N. Z. Bao, L. M. Shen, T. Takata and K. Domen,
“Self-Templated of Nanoporous CdS Nanostructures for
Highly Efficient Photocatalytic Hydrogen Production
under Visible Light,” Journal of Materials Chemistry,
Vol. 20, No. 1, 2008, pp. 110-117.
doi:10.1021/cm7029344
[8] X. Xu, F. Z. Zhang, S. L. Xu, J. He, L. Y. Wang, D. G.
Evans and X. Duan, “Template Synthesis of Nanoparticle
Arrays of CdS in Transparent Layered Double Hydroxide
Films,” Chemical Communications, 2009, pp. 7533-7535.
doi:10.1039/b918126a
[9] D. W. Jing and L. J. Guo, “A Novel Method for the
Preparation of a Highly Stable and Active CdS Photo-
catalyst with a Special Surface Nanostructure,” Journal of
Physical Chemistry B, Vol. 110, No. 23, 2006, pp. 11139-
11145. doi:10.1021/jp060905k
[10] C. M. Janet and R. P. Viswanath, “Large Scale Synthesis
of CdS Nanorods and Its Utilization in Photo-Catalytic H2
Production,” Nanotechnology, Vol. 17, 2006, pp. 5271-
5277.
[11] G. Burcu, G. Giancarlo, C. Emo and B. Niyazi, “Prepara-
tion of Stable CdS Nanoparticles in Aqueous Medium
and Their Hydrogen Generation Efficiencies in Photoly-
sis of Water,” International Journal of Hydrogen Energy,
Vol. 34, 2009, pp. 1176-1184.
Copyright © 2011 SciRes. WJNSE