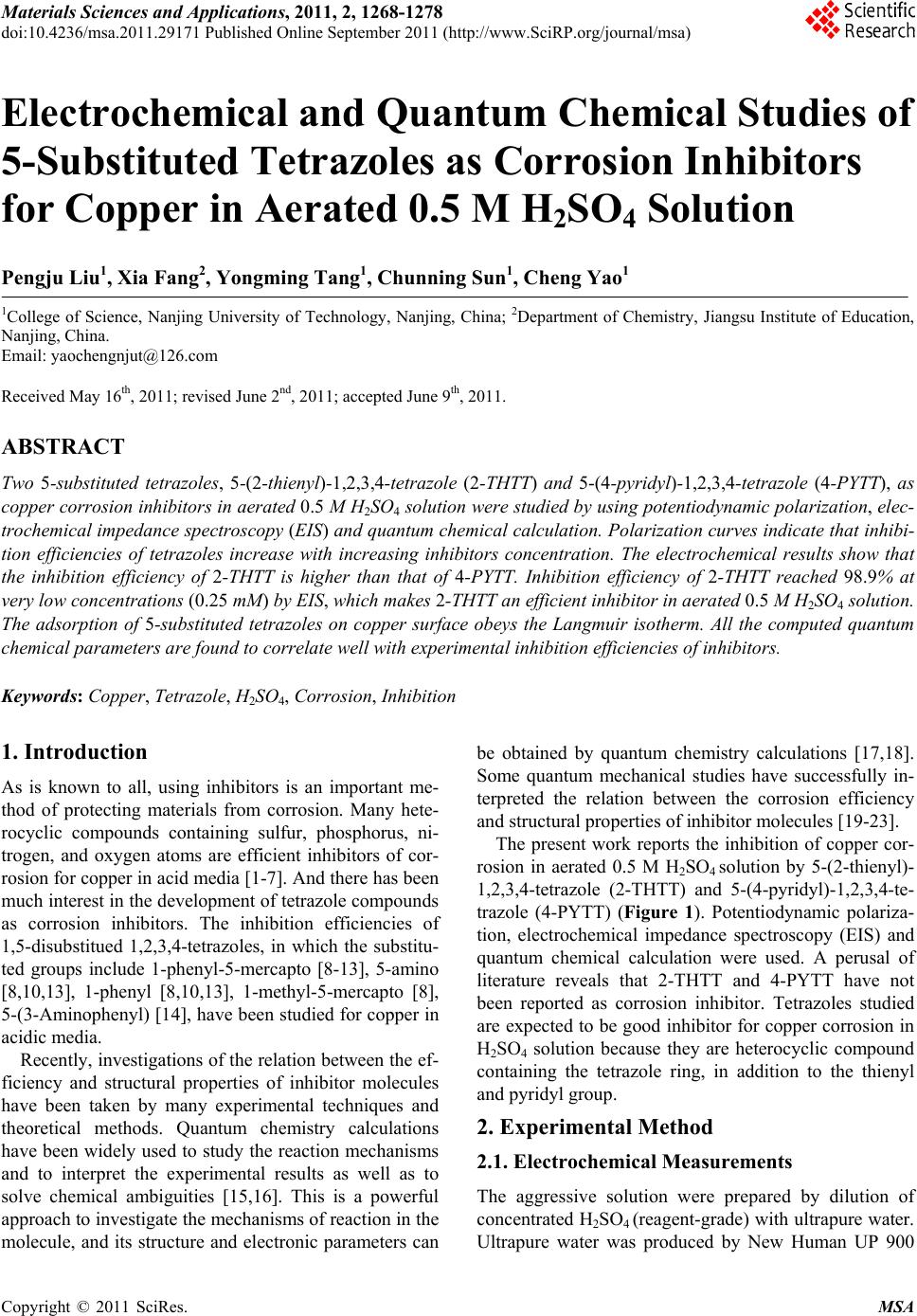 Materials Sciences and Applicatio ns, 2011, 2, 1268-1278 doi:10.4236/msa.2011.29171 Published Online September 2011 (http://www.SciRP.org/journal/msa) Copyright © 2011 SciRes. MSA Electrochemical and Quantum Chemical Studies of 5-Substituted Tetrazoles as Corrosion Inhibitors for Copper in Aerated 0.5 M H2SO4 Solution Pengju Liu1, Xia Fang2, Yongming Tang1, Chunning Sun1, Cheng Yao1 1College of Science, Nanjing University of Technology, Nanjing, China; 2Department of Chemistry, Jiangsu Institute of Education, Nanjing, China. Email: yaochengnjut@126.com Received May 16th, 2011; revised June 2nd, 2011; accepted June 9th, 2011. ABSTRACT Two 5-substituted tetrazoles, 5-(2-thienyl)-1,2,3,4-tetrazole (2-THTT) and 5-(4-pyridyl)-1,2,3,4-tetrazole (4-PYTT), as copper corrosion inhibitors in aerated 0.5 M H2SO4 solution were studied by using potentiodynamic polarization, elec- trochemical impedance spectroscopy (EIS) and quantum chemical calculation. Polarization curves indicate that inhibi- tion efficiencies of tetrazoles increase with increasing inhibitors concentration. The electrochemical results show that the inhibition efficiency of 2-THTT is higher than that of 4-PYTT. Inhibition efficiency of 2-THTT reached 98.9% at very low concentrations (0.25 mM ) by EIS, which makes 2-THTT an efficient inhibitor in aerated 0.5 M H2SO4 solution. The adsorption of 5-substituted tetrazoles on copper surface obeys the Langmuir isotherm. All the computed quantum chemical parameters are found to correlate well with experimental inhibition efficiencies of inhibitors. Keywords: Copper, Tetrazole, H2SO4, Corrosion, Inhibition 1. Introduction As is known to all, using inhibitors is an important me- thod of protecting materials from corrosion. Many hete- rocyclic compounds containing sulfur, phosphorus, ni- trogen, and oxygen atoms are efficient inhibitors of cor- rosion for copper in acid media [1-7]. And there has been much interest in the development of tetrazole compounds as corrosion inhibitors. The inhibition efficiencies of 1,5-disubstitued 1,2,3,4-tetrazoles, in which the substitu- ted groups include 1-phenyl-5-mercapto [8-13], 5-amino [8,10,13], 1-phenyl [8,10,13], 1-methyl-5-mercapto [8], 5-(3-Aminophenyl) [14], have been studied for copper in acidic media. Recently, investigations of the relation between the ef- ficiency and structural properties of inhibitor molecules have been taken by many experimental techniques and theoretical methods. Quantum chemistry calculations have been widely used to study the reaction mechanisms and to interpret the experimental results as well as to solve chemical ambiguities [15,16]. This is a powerful approach to investigate the mechanisms of reaction in the molecule, and its structure and electronic parameters can be obtained by quantum chemistry calculations [17,18]. Some quantum mechanical studies have successfully in- terpreted the relation between the corrosion efficiency and structural properties of inhibitor molecules [19-23]. The present work reports the inhibition of copper cor- rosion in aerated 0.5 M H2SO4 solution by 5-(2-thienyl)- 1,2,3,4-tetrazole (2-THTT) and 5-(4-pyridyl)-1,2,3,4-te- trazole (4-PYTT) (Figure 1). Potentiodynamic polariza- tion, electrochemical impedance spectroscopy (EIS) and quantum chemical calculation were used. A perusal of literature reveals that 2-THTT and 4-PYTT have not been reported as corrosion inhibitor. Tetrazoles studied are expected to be good inhibitor for copper corrosion in H2SO4 solution because they are heterocyclic compound containing the tetrazole ring, in addition to the thienyl and pyridyl group. 2. Experimental Method 2.1. Electrochemical Measurements The aggressive solution were prepared by dilution of concentrated H2SO4 (reagent-grade) with ultrapure water. Ultrapure water was produced by New Human UP 900
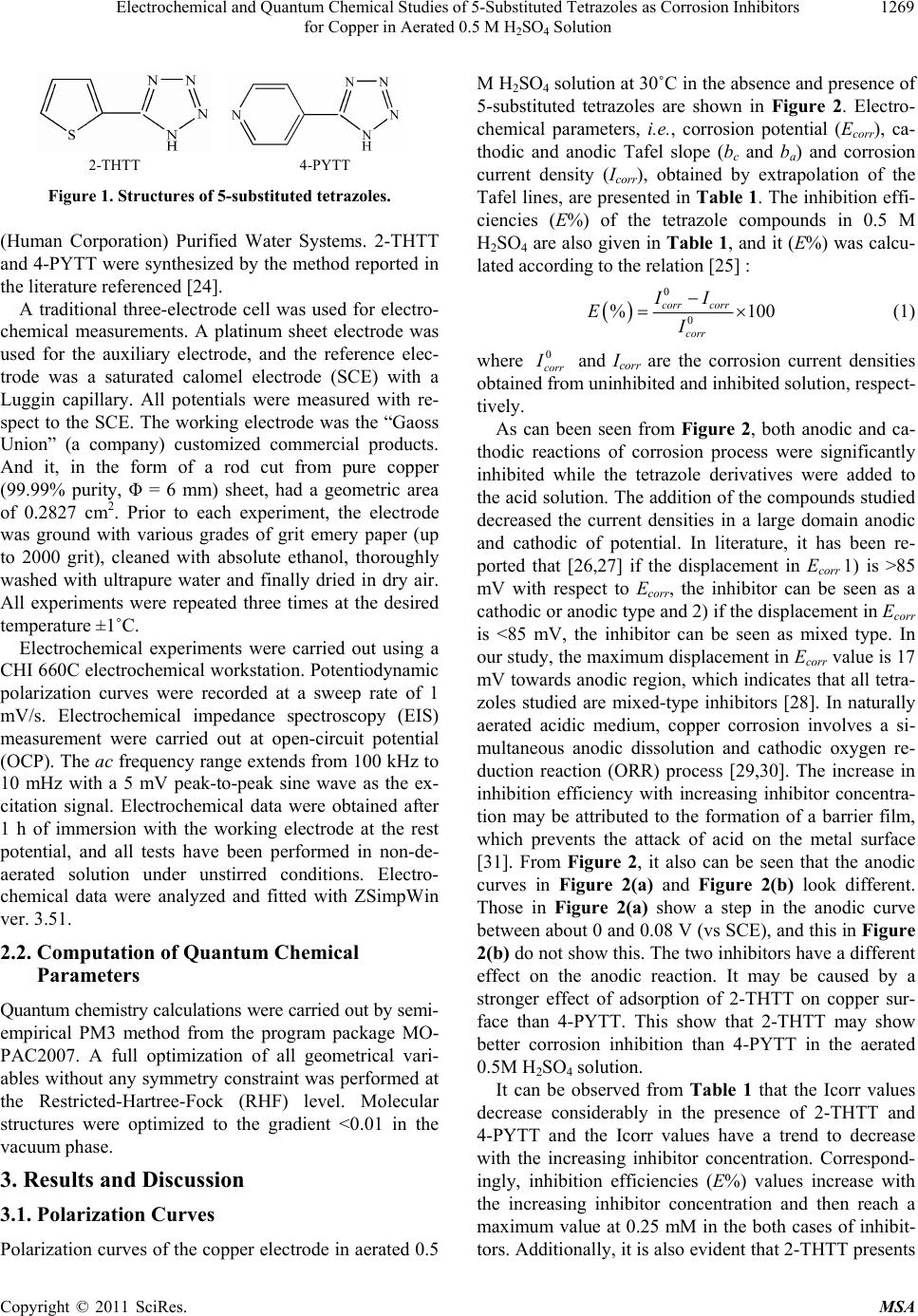 Electrochemical and Quantum Chemical Studies of 5-Substituted Tetrazoles as Corrosion Inhibitors 1269 for Copper in Aerated 0.5 M H2SO4 Solution 2-THTT 4-PYTT Figure 1. Structures of 5-substituted tetrazoles. (Human Corporation) Purified Water Systems. 2-THTT and 4-PYTT were synthesized by the method reported in the literature referenced [24]. A traditional three-electrode cell was used for electro- chemical measurements. A platinum sheet electrode was used for the auxiliary electrode, and the reference elec- trode was a saturated calomel electrode (SCE) with a Luggin capillary. All potentials were measured with re- spect to the SCE. The working electrode was the “Gaoss Union” (a company) customized commercial products. And it, in the form of a rod cut from pure copper (99.99% purity, Φ = 6 mm) sheet, had a geometric area of 0.2827 cm2. Prior to each experiment, the electrode was ground with various grades of grit emery paper (up to 2000 grit), cleaned with absolute ethanol, thoroughly washed with ultrapure water and finally dried in dry air. All experiments were repeated three times at the desired temperature ±1˚C. Electrochemical experiments were carried out using a CHI 660C electrochemical workstation. Potentiodynamic polarization curves were recorded at a sweep rate of 1 mV/s. Electrochemical impedance spectroscopy (EIS) measurement were carried out at open-circuit potential (OCP). The ac frequency range extends from 100 kHz to 10 mHz with a 5 mV peak-to-peak sine wave as the ex- citation signal. Electrochemical data were obtained after 1 h of immersion with the working electrode at the rest potential, and all tests have been performed in non-de- aerated solution under unstirred conditions. Electro- chemical data were analyzed and fitted with ZSimpWin ver. 3.51. 2.2. Computation of Quantum Chemical Parameters Quantum chemistry calculations were carried out by semi- empirical PM3 method from the program package MO- PAC2007. A full optimization of all geometrical vari- ables without any symmetry constraint was performed at the Restricted-Hartree-Fock (RHF) level. Molecular structures were optimized to the gradient <0.01 in the vacuum phase. 3. Results and Discussion 3.1. Polarization Curves Polarization curves of the copper electrode in aerated 0.5 M H2SO4 solution at 30˚C in the absence and presence of 5-substituted tetrazoles are shown in Figure 2. Electro- chemical parameters, i.e., corrosion potential (Ecorr), ca- thodic and anodic Tafel slope (bc and ba) and corrosion current density (Icorr), obtained by extrapolation of the Tafel lines, are presented in Table 1. The inhibition effi- ciencies (E%) of the tetrazole compounds in 0.5 M H2SO4 are also given in Table 1, and it (E%) was calcu- lated according to the relation [25] : 0 0 % 100 corr corr corr II EI (1) where 0 corr and Icorr are the corrosion current densities obtained from uninhibited and inhibited solution, respect- tively. As can been seen from Figure 2, both anodic and ca- thodic reactions of corrosion process were significantly inhibited while the tetrazole derivatives were added to the acid solution. The addition of the compounds studied decreased the current densities in a large domain anodic and cathodic of potential. In literature, it has been re- ported that [26,27] if the displacement in Ecorr 1) is >85 mV with respect to Ecorr, the inhibitor can be seen as a cathodic or anodic type and 2) if the displacement in Ecorr is <85 mV, the inhibitor can be seen as mixed type. In our study, the maximum displacement in Ecorr value is 17 mV towards anodic region, which indicates that all tetra- zoles studied are mixed-type inhibitors [28]. In naturally aerated acidic medium, copper corrosion involves a si- multaneous anodic dissolution and cathodic oxygen re- duction reaction (ORR) process [29,30]. The increase in inhibition efficiency with increasing inhibitor concentra- tion may be attributed to the formation of a barrier film, which prevents the attack of acid on the metal surface [31]. From Figure 2, it also can be seen that the anodic curves in Figure 2(a) and Figure 2(b) look different. Those in Figure 2(a) show a step in the anodic curve between about 0 and 0.08 V (vs SCE), and this in Figure 2(b) do not show this. The two inhibitors have a different effect on the anodic reaction. It may be caused by a stronger effect of adsorption of 2-THTT on copper sur- face than 4-PYTT. This show that 2-THTT may show better corrosion inhibition than 4-PYTT in the aerated 0.5M H2SO4 solution. It can be observed from Table 1 that the Icorr values decrease considerably in the presence of 2-THTT and 4-PYTT and the Icorr values have a trend to decrease with the increasing inhibitor concentration. Correspond- ingly, inhibition efficiencies (E%) values increase with the increasing inhibitor concentration and then reach a maximum value at 0.25 mM in the both cases of inhibit- tors. Additionally, it is also evident that 2-THTT presents Copyright © 2011 SciRes. MSA
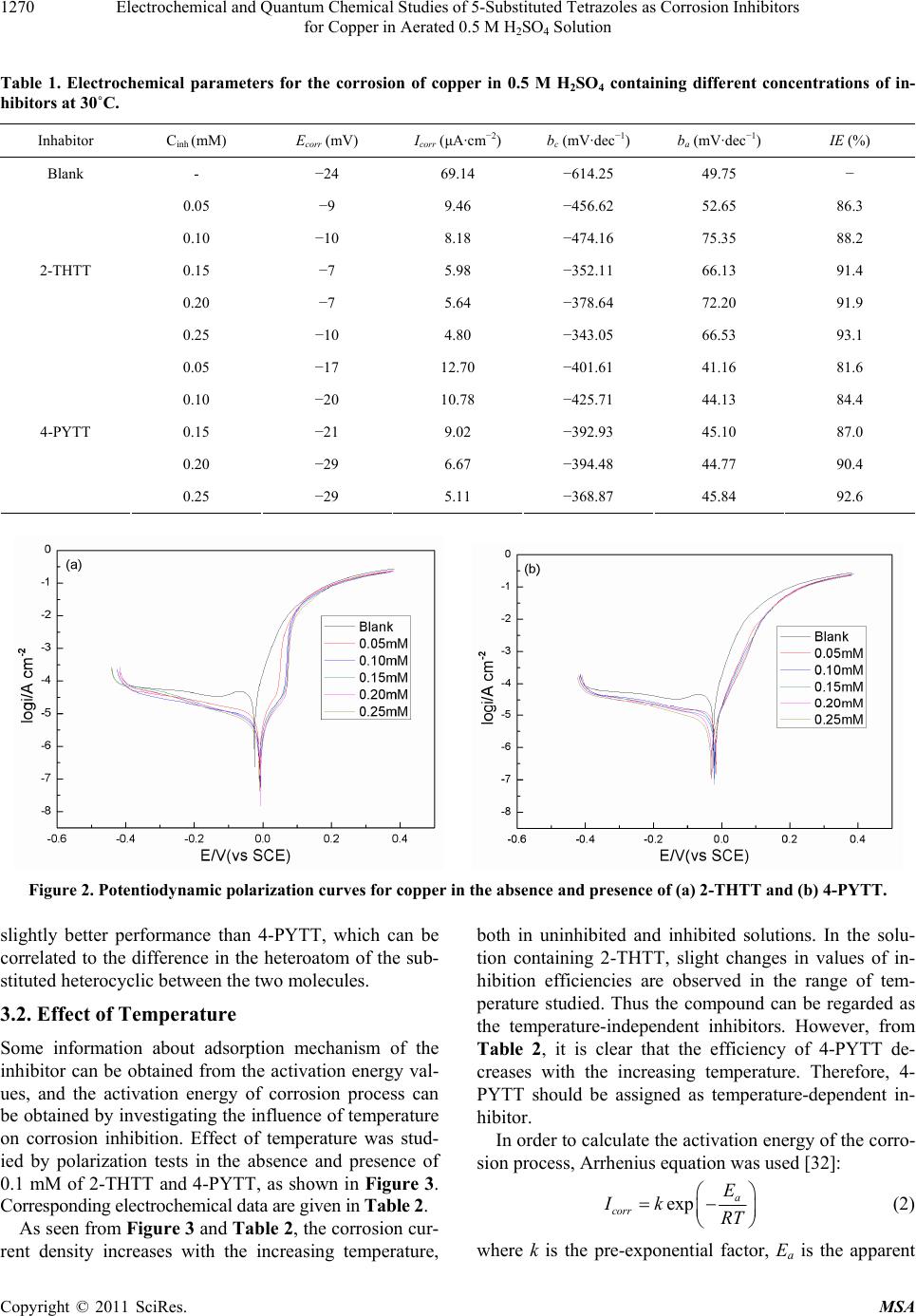 Electrochemical and Quantum Chemical Studies of 5-Substituted Tetrazoles as Corrosion Inhibitors for Copper in Aerated 0.5 M H2SO4 Solution Copyright © 2011 SciRes. MSA 1270 Table 1. Electrochemical parameters for the corrosion of copper in 0.5 M H2SO4 containing different concentrations of in- hibitors at 30˚C. Inhabitor Cinh (mM) Ecorr (mV) Icorr (μA·cm−2) bc (mV·dec−1) ba (mV·dec−1) IE (%) Blank - −24 69.14 −614.25 49.75 − 0.05 −9 9.46 −456.62 52.65 86.3 0.10 −10 8.18 −474.16 75.35 88.2 0.15 −7 5.98 −352.11 66.13 91.4 0.20 −7 5.64 −378.64 72.20 91.9 2-THTT 0.25 −10 4.80 −343.05 66.53 93.1 0.05 −17 12.70 −401.61 41.16 81.6 0.10 −20 10.78 −425.71 44.13 84.4 0.15 −21 9.02 −392.93 45.10 87.0 0.20 −29 6.67 −394.48 44.77 90.4 4-PYTT 0.25 −29 5.11 −368.87 45.84 92.6 Figure 2. Potentiodynamic polarization curves for copper in the absence and presence of (a) 2-THTT and (b) 4-PYTT. slightly better performance than 4-PYTT, which can be correlated to the difference in the heteroatom of the sub- stituted heterocyclic between the two molecules. 3.2. Effect of Temperature Some information about adsorption mechanism of the inhibitor can be obtained from the activation energy val- ues, and the activation energy of corrosion process can be obtained by investigating the influence of temperature on corrosion inhibition. Effect of temperature was stud- ied by polarization tests in the absence and presence of 0.1 mM of 2-THTT and 4-PYTT, as shown in Figure 3. Corresponding electrochemical data are given in Table 2. As seen from Figure 3 and Table 2, the corrosion cur- rent density increases with the increasing temperature, both in uninhibited and inhibited solutions. In the solu- tion containing 2-THTT, slight changes in values of in- hibition efficiencies are observed in the range of tem- perature studied. Thus the compound can be regarded as the temperature-independent inhibitors. However, from Table 2, it is clear that the efficiency of 4-PYTT de- creases with the increasing temperature. Therefore, 4- PYTT should be assigned as temperature-dependent in- hibitor. In order to calculate the activation energy of the corro- sion process, Arrhenius equation was used [32]: exp a corr E Ik RT (2) where k is the pre-exponential factor, Ea is the apparent
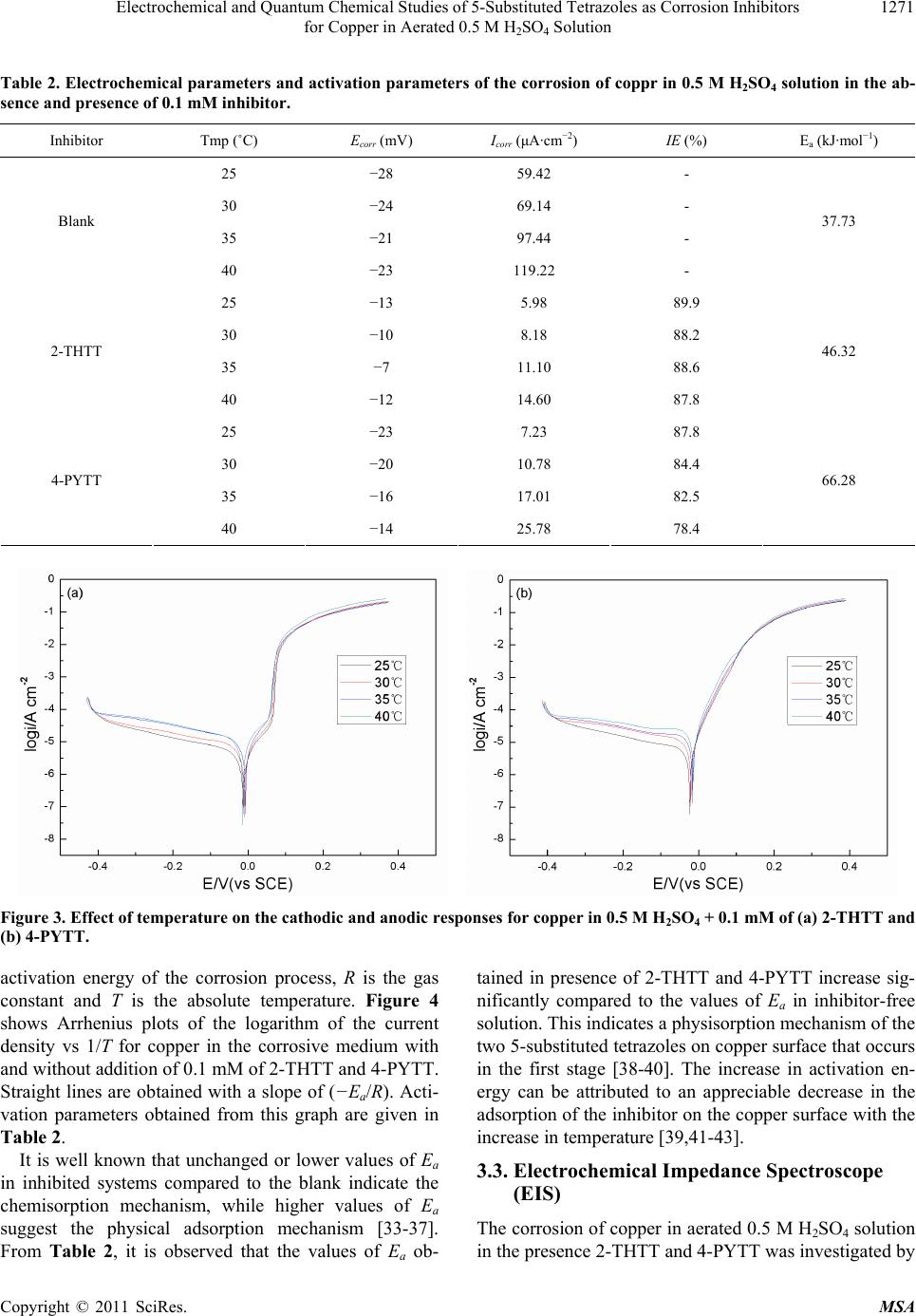 Electrochemical and Quantum Chemical Studies of 5-Substituted Tetrazoles as Corrosion Inhibitors 1271 for Copper in Aerated 0.5 M H2SO4 Solution Table 2. Electrochemical parameters and activation parameters of the corrosion of coppr in 0.5 M H2SO4 solution in the ab- sence and presence of 0.1 mM inhibitor. Inhibitor Tmp (˚C) Ecorr (mV) Icorr (μA·cm−2) IE (%) Ea (kJ·mol−1) 25 −28 59.42 - 30 −24 69.14 - 35 −21 97.44 - Blank 40 −23 119.22 - 37.73 25 −13 5.98 89.9 30 −10 8.18 88.2 35 −7 11.10 88.6 2-THTT 40 −12 14.60 87.8 46.32 25 −23 7.23 87.8 30 −20 10.78 84.4 35 −16 17.01 82.5 4-PYTT 40 −14 25.78 78.4 66.28 Figure 3. Effect of temperature on the cathodic and anodic responses for copper in 0.5 M H2SO4 + 0.1 mM of (a) 2-THTT and (b) 4-PYTT. activation energy of the corrosion process, R is the gas constant and T is the absolute temperature. Figure 4 shows Arrhenius plots of the logarithm of the current density vs 1/T for copper in the corrosive medium with and without addition of 0.1 mM of 2-THTT and 4-PYTT. Straight lines are obtained with a slope of (−Ea/R). Acti- vation parameters obtained from this graph are given in Table 2. It is well known that unchanged or lower values of Ea in inhibited systems compared to the blank indicate the chemisorption mechanism, while higher values of Ea suggest the physical adsorption mechanism [33-37]. From Table 2, it is observed that the values of Ea ob- tained in presence of 2-THTT and 4-PYTT increase sig- nificantly compared to the values of Ea in inhibitor-free solution. This indicates a physisorption mechanism of the two 5-substituted tetrazoles on copper surface that occurs in the first stage [38-40]. The increase in activation en- ergy can be attributed to an appreciable decrease in the adsorption of the inhibitor on the copper surface with the increase in temperature [39,41-43]. 3.3. Electrochemical Impedance Spectroscope (EIS) The corrosion of copper in aerated 0.5 M H2SO4 solution in the presence 2-THTT and 4-PYTT was investigated by Copyright © 2011 SciRes. MSA
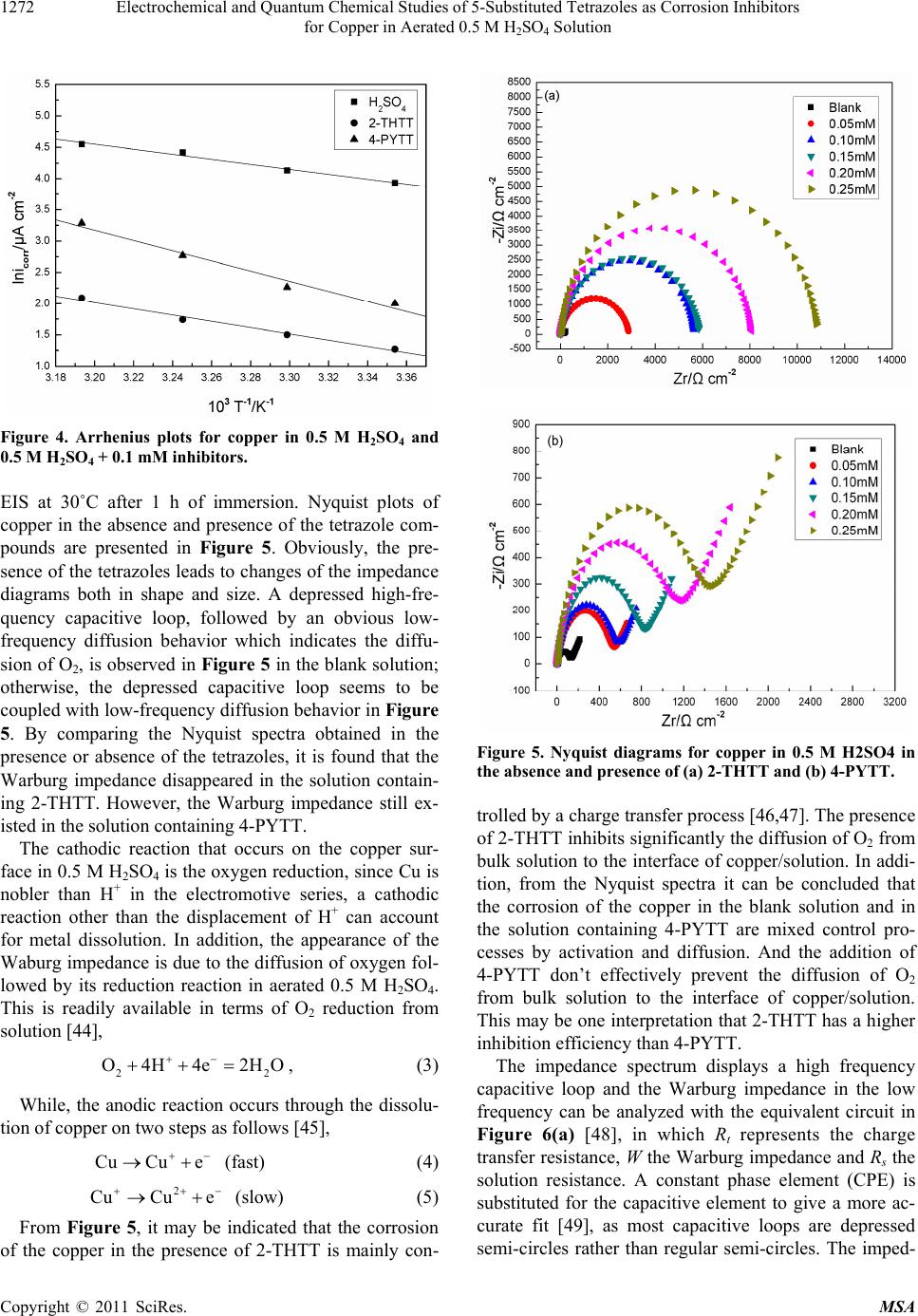 Electrochemical and Quantum Chemical Studies of 5-Substituted Tetrazoles as Corrosion Inhibitors 1272 for Copper in Aerated 0.5 M H2SO4 Solution Figure 4. Arrhenius plots for copper in 0.5 M H2SO4 and 0.5 M H2SO4 + 0.1 mM inhibitors. EIS at 30˚C after 1 h of immersion. Nyquist plots of copper in the absence and presence of the tetrazole com- pounds are presented in Figure 5. Obviously, the pre- sence of the tetrazoles leads to changes of the impedance diagrams both in shape and size. A depressed high-fre- quency capacitive loop, followed by an obvious low- frequency diffusion behavior which indicates the diffu- sion of O2, is observed in Figure 5 in the blank solution; otherwise, the depressed capacitive loop seems to be coupled with low-frequency diffusion behavior in Figure 5. By comparing the Nyquist spectra obtained in the presence or absence of the tetrazoles, it is found that the Warburg impedance disappeared in the solution contain- ing 2-THTT. However, the Warburg impedance still ex- isted in the solution containing 4-PYTT. The cathodic reaction that occurs on the copper sur- face in 0.5 M H2SO4 is the oxygen reduction, since Cu is nobler than H+ in the electromotive series, a cathodic reaction other than the displacement of H+ can account for metal dissolution. In addition, the appearance of the Waburg impedance is due to the diffusion of oxygen fol- lowed by its reduction reaction in aerated 0.5 M H2SO4. This is readily available in terms of O2 reduction from solution [44], 2 O4H4e2HO 2 , (3) While, the anodic reaction occurs through the dissolu- tion of copper on two steps as follows [45], CuCu e (fast) (4) 2 CuCu e (slow) (5) From Figure 5, it may be indicated that the corrosion of the copper in the presence of 2-THTT is mainly con- Figure 5. Nyquist diagrams for copper in 0.5 M H2SO4 in the absence and presence of (a) 2-THTT and (b) 4-PYTT. trolled by a charge transfer process [46,47]. The presence of 2-THTT inhibits significantly the diffusion of O2 from bulk solution to the interface of copper/solution. In addi- tion, from the Nyquist spectra it can be concluded that the corrosion of the copper in the blank solution and in the solution containing 4-PYTT are mixed control pro- cesses by activation and diffusion. And the addition of 4-PYTT don’t effectively prevent the diffusion of O2 from bulk solution to the interface of copper/solution. This may be one interpretation that 2-THTT has a higher inhibition efficiency than 4-PYTT. The impedance spectrum displays a high frequency capacitive loop and the Warburg impedance in the low frequency can be analyzed with the equivalent circuit in Figure 6(a) [48], in which Rt represents the charge transfer resistance, W the Warburg impedance and Rs the solution resistance. A constant phase element (CPE) is substituted for the capacitive element to give a more ac- curate fit [49], as most capacitive loops are depressed semi-circles rather than regular semi-circles. The imped- Copyright © 2011 SciRes. MSA
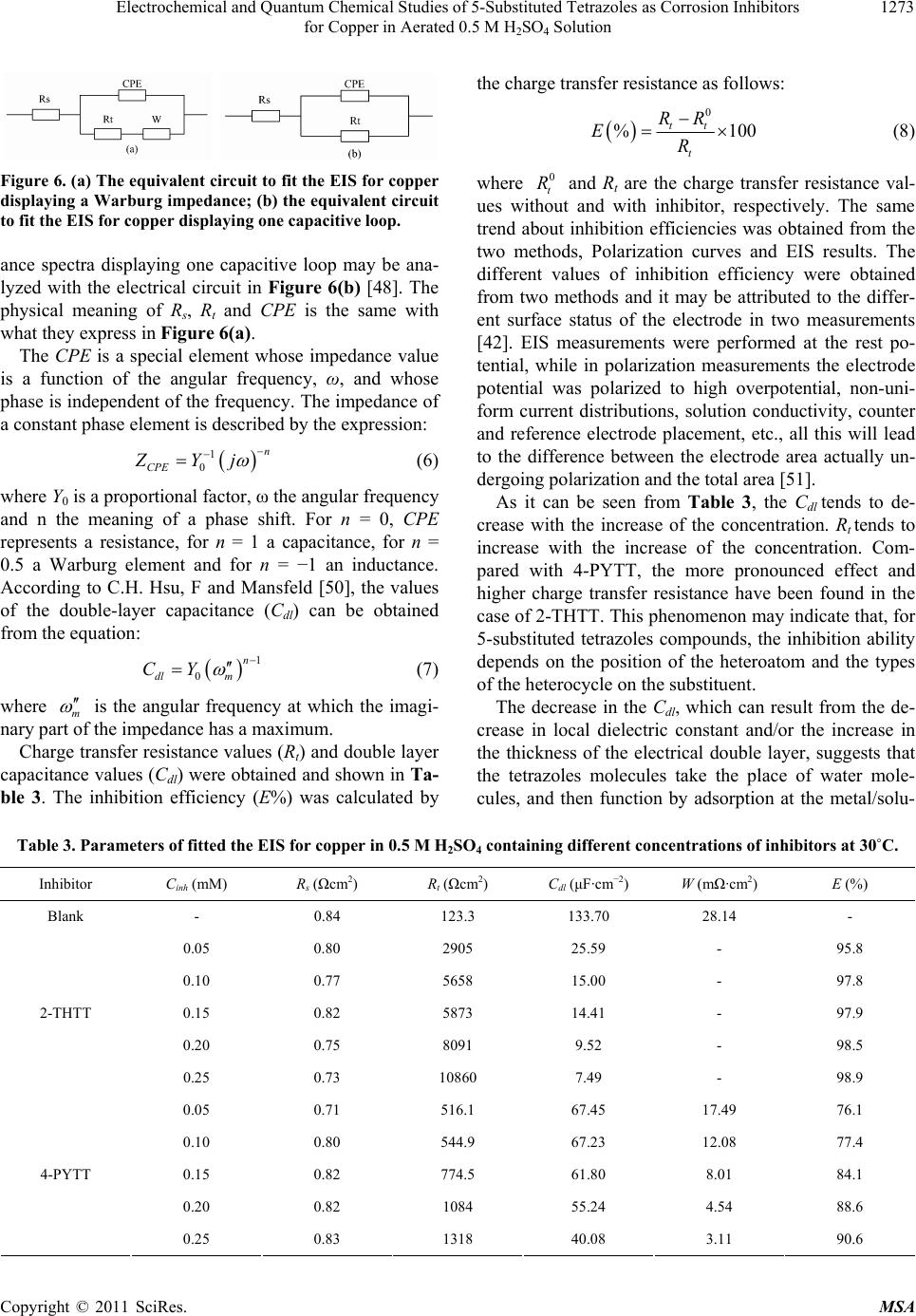 Electrochemical and Quantum Chemical Studies of 5-Substituted Tetrazoles as Corrosion Inhibitors for Copper in Aerated 0.5 M H2SO4 Solution Copyright © 2011 SciRes. MSA 1273 the charge transfer resistance as follows: 0 % 100 tt t RR ER (8) Figure 6. (a) The equivalent circuit to fit the EIS for copper displaying a Warburg impedance ; (b) the equivalent circuit to fit the EIS for copper displaying one capacitive loop. where and Rt are the charge transfer resistance val- ues without and with inhibitor, respectively. The same trend about inhibition efficiencies was obtained from the two methods, Polarization curves and EIS results. The different values of inhibition efficiency were obtained from two methods and it may be attributed to the differ- ent surface status of the electrode in two measurements [42]. EIS measurements were performed at the rest po- tential, while in polarization measurements the electrode potential was polarized to high overpotential, non-uni- form current distributions, solution conductivity, counter and reference electrode placement, etc., all this will lead to the difference between the electrode area actually un- dergoing polarization and the total area [51]. 0 t R ance spectra displaying one capacitive loop may be ana- lyzed with the electrical circuit in Figure 6(b) [48]. The physical meaning of Rs, Rt and CPE is the same with what they express in Figure 6(a). The CPE is a special element whose impedance value is a function of the angular frequency, ω, and whose phase is independent of the frequency. The impedance of a constant phase element is described by the expression: 1 0 n CPE ZYj (6) where Y0 is a proportional factor, ω the angular frequency and n the meaning of a phase shift. For n = 0, CPE represents a resistance, for n = 1 a capacitance, for n = 0.5 a Warburg element and for n = −1 an inductance. According to C.H. Hsu, F and Mansfeld [50], the values of the double-layer capacitance (Cdl) can be obtained from the equation: As it can be seen from Table 3, the Cdl tends to de- crease with the increase of the concentration. Rt tends to increase with the increase of the concentration. Com- pared with 4-PYTT, the more pronounced effect and higher charge transfer resistance have been found in the case of 2-THTT. This phenomenon may indicate that, for 5-substituted tetrazoles compounds, the inhibition ability depends on the position of the heteroatom and the types of the heterocycle on the substituent. 1 0 n dl m CY (7) where m is the angular frequency at which the imagi- nary part of the impedance has a maximum. The decrease in the Cdl, which can result from the de- crease in local dielectric constant and/or the increase in the thickness of the electrical double layer, suggests that the tetrazoles molecules take the place of water mole- cules, and then function by adsorption at the metal/solu- Charge transfer resistance values (Rt) and double layer capacitance values (Cdl) were obtained and shown in Ta- ble 3. The inhibition efficiency (E%) was calculated by Table 3. Parameters of fitted the EIS for copper in 0.5 M H2SO4 containing different concentrations of inhibitors at 30˚C. Inhibitor Cinh (mM) Rs (Ωcm2) Rt (Ωcm2) Cdl (μF·cm−2) W (mΩ·cm2) E (%) Blank - 0.84 123.3 133.70 28.14 - 0.05 0.80 2905 25.59 - 95.8 0.10 0.77 5658 15.00 - 97.8 0.15 0.82 5873 14.41 - 97.9 0.20 0.75 8091 9.52 - 98.5 2-THTT 0.25 0.73 10860 7.49 - 98.9 0.05 0.71 516.1 67.45 17.49 76.1 0.10 0.80 544.9 67.23 12.08 77.4 0.15 0.82 774.5 61.80 8.01 84.1 0.20 0.82 1084 55.24 4.54 88.6 4-PYTT 0.25 0.83 1318 40.08 3.11 90.6
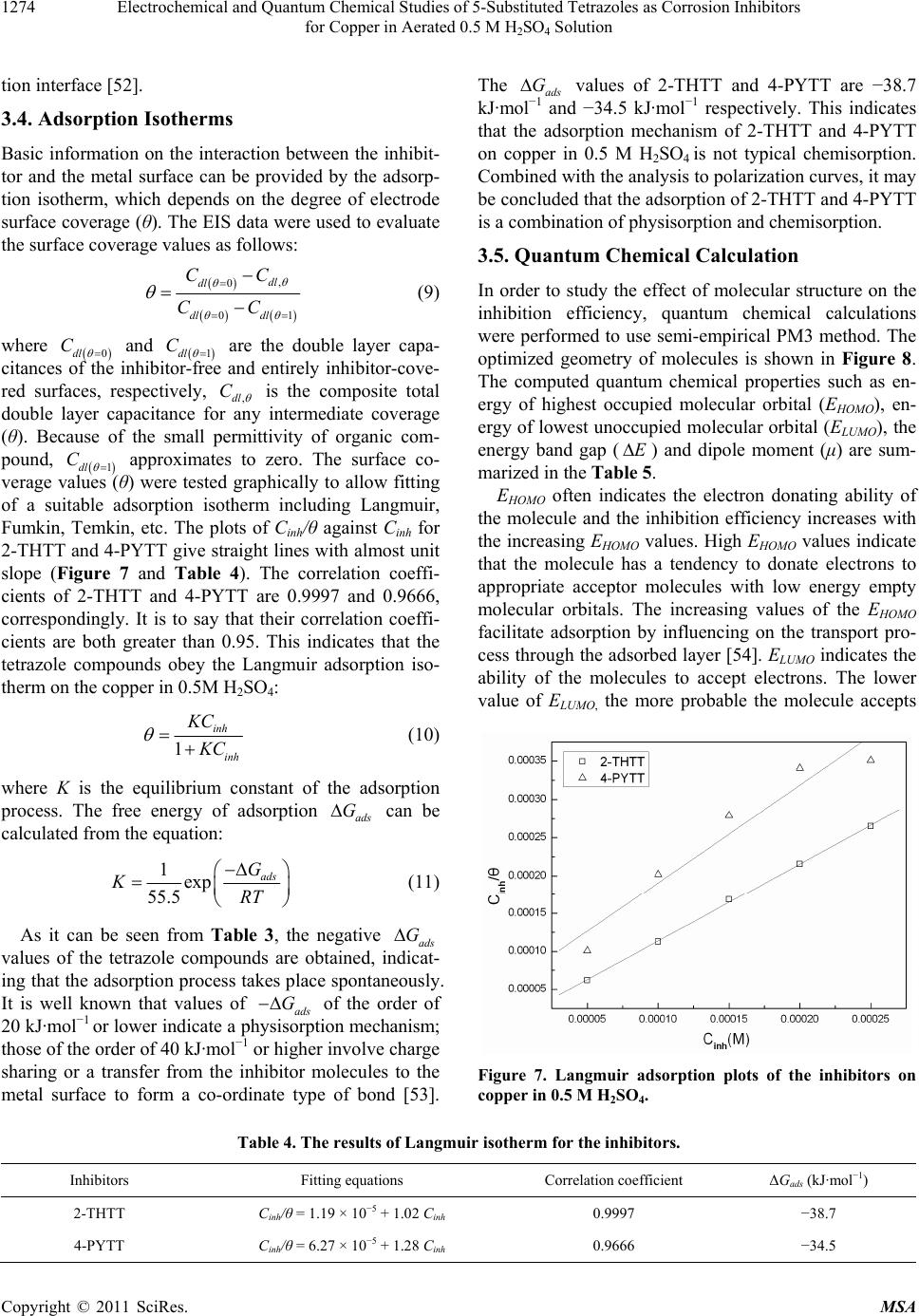 Electrochemical and Quantum Chemical Studies of 5-Substituted Tetrazoles as Corrosion Inhibitors 1274 for Copper in Aerated 0.5 M H2SO4 Solution tion interface [52]. 3.4. Adsorption Isotherms Basic information on the interaction between the inhibit- tor and the metal surface can be provided by the adsorp- tion isotherm, which depends on the degree of electrode surface coverage (θ). The EIS data were used to evaluate the surface coverage values as follows: , 0 01 dl dl dl dl CC CC (9) where 0dl and 1dl are the double layer capa- citances of the inhibitor-free and entirely inhibitor-cove- red surfaces, respectively, ,dl C C C is the composite total double layer capacitance for any intermediate coverage (θ). Because of the small permittivity of organic com- pound, 1dl approximates to zero. The surface co- verage values (θ) were tested graphically to allow fitting of a suitable adsorption isotherm including Langmuir, Fumkin, Temkin, etc. The plots of Cinh/θ against Cinh for 2-THTT and 4-PYTT give straight lines with almost unit slope (Figure 7 and Table 4). The correlation coeffi- cients of 2-THTT and 4-PYTT are 0.9997 and 0.9666, correspondingly. It is to say that their correlation coeffi- cients are both C greater than 0.95. This indicates that the tetrazole compounds obey the Langmuir adsorption iso- therm on the copper in 0.5M H2SO4: 1 inh inh KC C (10) where K is the equilibrium constant of the adsorption process. The free energy of adsorption can be calculated from the equation: ads G 1exp 55.5 ads G KRT (11) As it can be seen from Table 3, the negative ads G values of the tetrazole compounds are obtained, indicat- ing that the adsorption process takes place spontaneously. It is well known that values of ads of the order of 20 kJ·mol−1 or lower indicate a physisorption mechanism; those of the order of 40 kJ·mol−1 or higher involve charge sharing or a transfer from the inhibitor molecules to the metal surface to form a co-ordinate type of bond [53]. G The ads G values of 2-THTT and 4-PYTT are −38.7 kJ·mol−1 and −34.5 kJ·mol−1 respectively. This indicates that the adsorption mechanism of 2-THTT and 4-PYTT on copper in 0.5 M H2SO4 is not typical chemisorption. Combined with the analysis to polarization curves, it may be concluded that the adsorption of 2-THTT and 4-PYTT is a combination of physisorption and chemisorption. 3.5. Quantum Chemical Calculation In order to study the effect of molecular structure on the inhibition efficiency, quantum chemical calculations were performed to use semi-empirical PM3 method. The optimized geometry of molecules is shown in Figure 8. The computed quantum chemical properties such as en- ergy of highest occupied molecular orbital (EHOMO), en- ergy of lowest unoccupied molecular orbital (ELUMO), the energy band gap (E ) and dipole moment (μ) are sum- marized in the Table 5. EHOMO often indicates the electron donating ability of the molecule and the inhibition efficiency increases with the increasing EHOMO values. High EHOMO values indicate that the molecule has a tendency to donate electrons to appropriate acceptor molecules with low energy empty molecular orbitals. The increasing values of the EHOMO facilitate adsorption by influencing on the transport pro- cess through the adsorbed layer [54]. ELUMO indicates the ability of the molecules to accept electrons. The lower value of ELUMO, the more probable the molecule accepts Figure 7. Langmuir adsorption plots of the inhibitors on copper in 0.5 M H2SO4. Table 4. The results of Langmuir isotherm for the inhibitors. Inhibitors Fitting equations Correlation coefficient ΔGads (kJ·mol−1) 2-THTT Cinh/θ = 1.19 × 10−5 + 1.02 Cinh 0.9997 −38.7 4-PYTT Cinh/θ = 6.27 × 10−5 + 1.28 Cinh 0.9666 −34.5 Copyright © 2011 SciRes. MSA
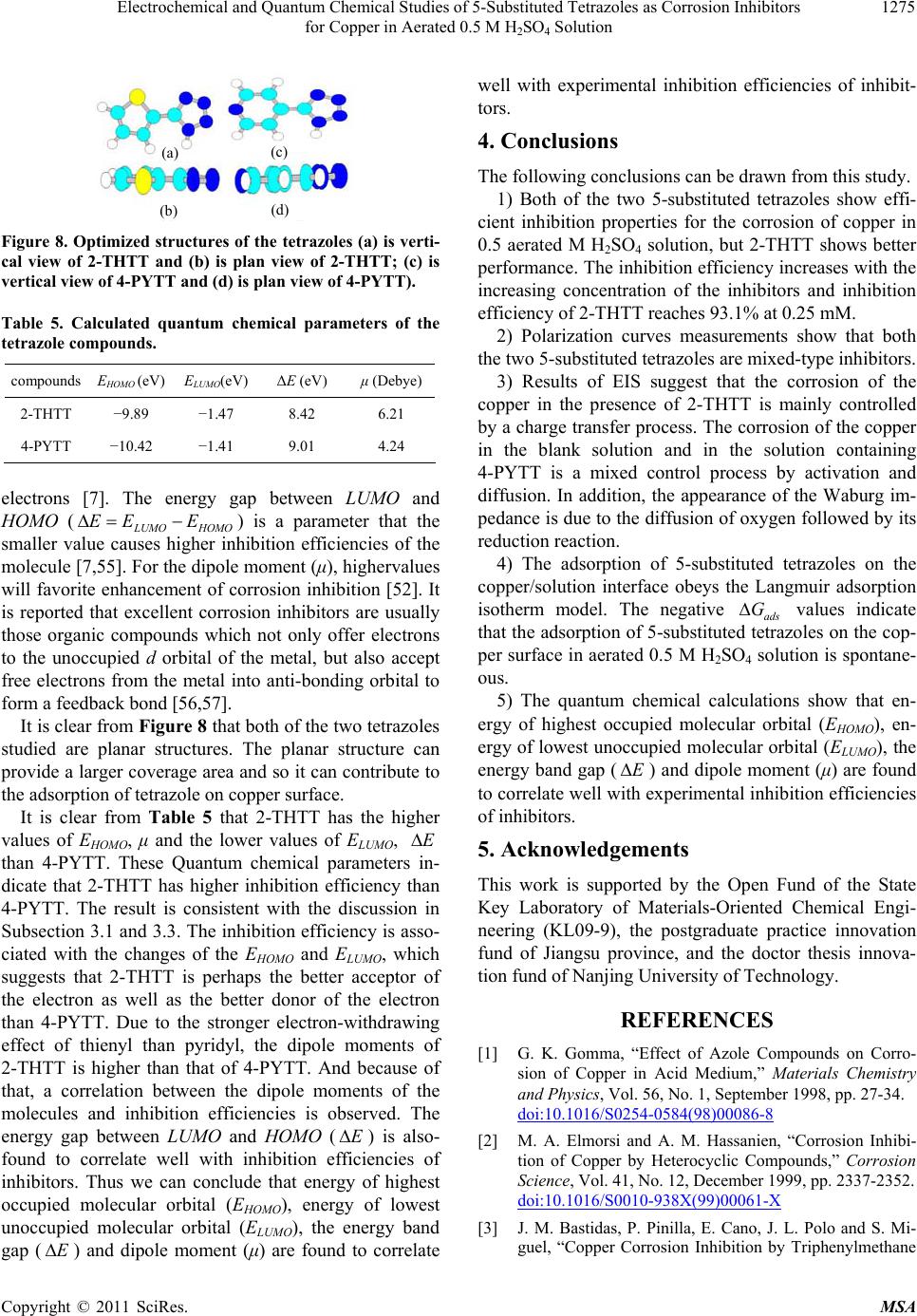 Electrochemical and Quantum Chemical Studies of 5-Substituted Tetrazoles as Corrosion Inhibitors 1275 for Copper in Aerated 0.5 M H2SO4 Solution (a) (c) (b) (d) Figure 8. Optimized structures of the tetrazoles (a) is verti- cal view of 2-THTT and (b) is plan view of 2-THTT; (c) is vertical view of 4-PYTT and (d) is plan view of 4-PYTT). Table 5. Calculated quantum chemical parameters of the tetrazole compounds. compounds EHOMO (eV) ELUMO(eV) ΔE (eV) μ (Debye) 2-THTT −9.89 −1.47 8.42 6.21 4-PYTT −10.42 −1.41 9.01 4.24 electrons [7]. The energy gap between LUMO and HOMO ( UMOHOMO ) is a parameter that the smaller value causes higher inhibition efficiencies of the molecule [7,55]. For the dipole moment (μ), highervalues will favorite enhancement of corrosion inhibition [52]. It is reported that excellent corrosion inhibitors are usually those organic compounds which not only offer electrons to the unoccupied d orbital of the metal, but also accept free electrons from the metal into anti-bonding orbital to form a feedback bond [56,57]. EE E It is clear from Figure 8 that both of the two tetrazoles studied are planar structures. The planar structure can provide a larger coverage area and so it can contribute to the adsorption of tetrazole on copper surface. It is clear from Table 5 that 2-THTT has the higher values of EHOMO, μ and the lower values of ELUMO, E than 4-PYTT. These Quantum chemical parameters in- dicate that 2-THTT has higher inhibition efficiency than 4-PYTT. The result is consistent with the discussion in Subsection 3.1 and 3.3. The inhibition efficiency is asso- ciated with the changes of the EHOMO and ELUMO, which suggests that 2-THTT is perhaps the better acceptor of the electron as well as the better donor of the electron than 4-PYTT. Due to the stronger electron-withdrawing effect of thienyl than pyridyl, the dipole moments of 2-THTT is higher than that of 4-PYTT. And because of that, a correlation between the dipole moments of the molecules and inhibition efficiencies is observed. The energy gap between LUMO and HOMO () is also- found to correlate well with inhibition efficiencies of inhibitors. Thus we can conclude that energy of highest occupied molecular orbital (EHOMO), energy of lowest unoccupied molecular orbital (ELUMO), the energy band gap () and dipole moment (μ) are found to correlate well with experimental inhibition efficiencies of inhibit- tors. E E 4. Conclusions The following conclusions can be drawn from this study. 1) Both of the two 5-substituted tetrazoles show effi- cient inhibition properties for the corrosion of copper in 0.5 aerated M H2SO4 solution, but 2-THTT shows better performance. The inhibition efficiency increases with the increasing concentration of the inhibitors and inhibition efficiency of 2-THTT reaches 93.1% at 0.25 mM. 2) Polarization curves measurements show that both the two 5-substituted tetrazoles are mixed-type inhibitors. 3) Results of EIS suggest that the corrosion of the copper in the presence of 2-THTT is mainly controlled by a charge transfer process. The corrosion of the copper in the blank solution and in the solution containing 4-PYTT is a mixed control process by activation and diffusion. In addition, the appearance of the Waburg im- pedance is due to the diffusion of oxygen followed by its reduction reaction. 4) The adsorption of 5-substituted tetrazoles on the copper/solution interface obeys the Langmuir adsorption isotherm model. The negative ads values indicate that the adsorption of 5-substituted tetrazoles on the cop- per surface in aerated 0.5 M H2SO4 solution is spontane- ous. G 5) The quantum chemical calculations show that en- ergy of highest occupied molecular orbital (EHOMO), en- ergy of lowest unoccupied molecular orbital (ELUMO), the energy band gap (E ) and dipole moment (μ) are found to correlate well with experimental inhibition efficiencies of inhibitors. 5. Acknowledgements This work is supported by the Open Fund of the State Key Laboratory of Materials-Oriented Chemical Engi- neering (KL09-9), the postgraduate practice innovation fund of Jiangsu province, and the doctor thesis innova- tion fund of Nanjing University of Technology. REFERENCES [1] G. K. Gomma, “Effect of Azole Compounds on Corro- sion of Copper in Acid Medium,” Materials Chemistry and Physics, Vol. 56, No. 1, September 1998, pp. 27-34. doi:10.1016/S0254-0584(98)00086-8 [2] M. A. Elmorsi and A. M. Hassanien, “Corrosion Inhibi- tion of Copper by Heterocyclic Compounds,” Corrosion Science, Vol. 41, No. 12, December 1999, pp. 2337-2352. doi:10.1016/S0010-938X(99)00061-X [3] J. M. Bastidas, P. Pinilla, E. Cano, J. L. Polo and S. Mi- guel, “Copper Corrosion Inhibition by Triphenylmethane Copyright © 2011 SciRes. MSA
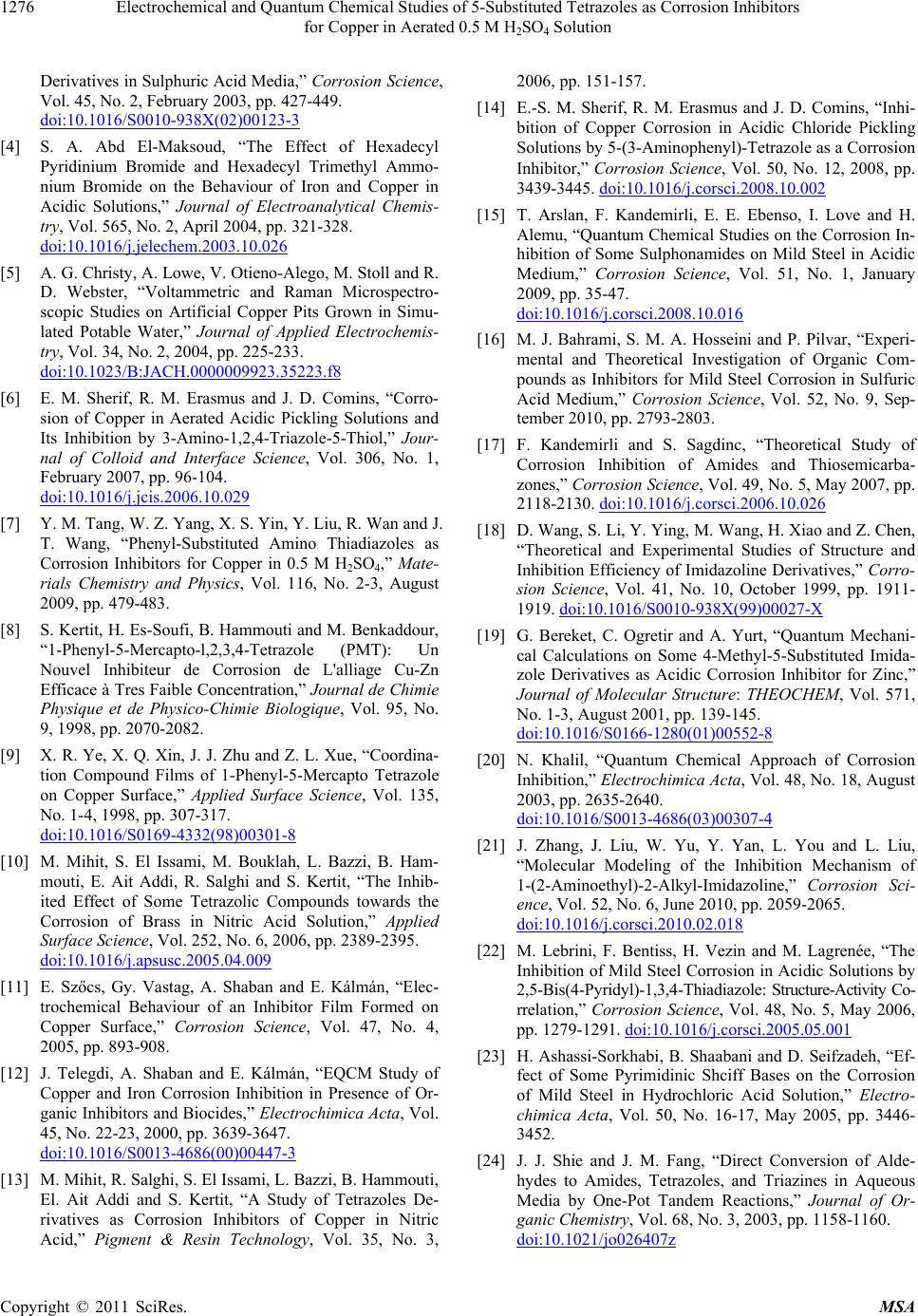 Electrochemical and Quantum Chemical Studies of 5-Substituted Tetrazoles as Corrosion Inhibitors 1276 for Copper in Aerated 0.5 M H2SO4 Solution Derivatives in Sulphuric Acid Media,” Corrosion Science, Vol. 45, No. 2, February 2003, pp. 427-449. doi:10.1016/S0010-938X(02)00123-3 [4] S. A. Abd El-Maksoud, “The Effect of Hexadecyl Pyridinium Bromide and Hexadecyl Trimethyl Ammo- nium Bromide on the Behaviour of Iron and Copper in Acidic Solutions,” Journal of Electroanalytical Chemis- try, Vol. 565, No. 2, April 2004, pp. 321-328. doi:10.1016/j.jelechem.2003.10.026 [5] A. G. Christy, A. Lowe, V. Otieno-Alego, M. Stoll and R. D. Webster, “Voltammetric and Raman Microspectro- scopic Studies on Artificial Copper Pits Grown in Simu- lated Potable Water,” Journal of Applied Electrochemis- try, Vol. 34, No. 2, 2004, pp. 225-233. doi:10.1023/B:JACH.0000009923.35223.f8 [6] E. M. Sherif, R. M. Erasmus and J. D. Comins, “Corro- sion of Copper in Aerated Acidic Pickling Solutions and Its Inhibition by 3-Amino-1,2,4-Triazole-5-Thiol,” Jour- nal of Colloid and Interface Science, Vol. 306, No. 1, February 2007, pp. 96-104. doi:10.1016/j.jcis.2006.10.029 [7] Y. M. Tang, W. Z. Yang, X. S. Yin, Y. Liu, R. Wan and J. T. Wang, “Phenyl-Substituted Amino Thiadiazoles as Corrosion Inhibitors for Copper in 0.5 M H2SO4,” Mate- rials Chemistry and Physics, Vol. 116, No. 2-3, August 2009, pp. 479-483. [8] S. Kertit, H. Es-Soufi, B. Hammouti and M. Benkaddour, “1-Phenyl-5-Mercapto-l,2,3,4-Tetrazole (PMT): Un Nouvel Inhibiteur de Corrosion de L'alliage Cu-Zn Efficace à Tres Faible Concentration,” Journal de Chimie Physique et de Physico-Chimie Biologique, Vol. 95, No. 9, 1998, pp. 2070-2082. [9] X. R. Ye, X. Q. Xin, J. J. Zhu and Z. L. Xue, “Coordina- tion Compound Films of 1-Phenyl-5-Mercapto Tetrazole on Copper Surface,” Applied Surface Science, Vol. 135, No. 1-4, 1998, pp. 307-317. doi:10.1016/S0169-4332(98)00301-8 [10] M. Mihit, S. El Issami, M. Bouklah, L. Bazzi, B. Ham- mouti, E. Ait Addi, R. Salghi and S. Kertit, “The Inhib- ited Effect of Some Tetrazolic Compounds towards the Corrosion of Brass in Nitric Acid Solution,” Applied Surface Science, Vol. 252, No. 6, 2006, pp. 2389-2395. doi:10.1016/j.apsusc.2005.04.009 [11] E. Szőcs, Gy. Vastag, A. Shaban and E. Kálmán, “Elec- trochemical Behaviour of an Inhibitor Film Formed on Copper Surface,” Corrosion Science, Vol. 47, No. 4, 2005, pp. 893-908. [12] J. Telegdi, A. Shaban and E. Kálmán, “EQCM Study of Copper and Iron Corrosion Inhibition in Presence of Or- ganic Inhibitors and Biocides,” Electrochimica Acta, Vol. 45, No. 22-23, 2000, pp. 3639-3647. doi:10.1016/S0013-4686(00)00447-3 [13] M. Mihit, R. Salghi, S. El Issami, L. Bazzi, B. Hammouti, El. Ait Addi and S. Kertit, “A Study of Tetrazoles De- rivatives as Corrosion Inhibitors of Copper in Nitric Acid,” Pigment & Resin Technology, Vol. 35, No. 3, 2006, pp. 151-157. [14] E.-S. M. Sherif, R. M. Erasmus and J. D. Comins, “Inhi- bition of Copper Corrosion in Acidic Chloride Pickling Solutions by 5-(3-Aminophenyl)-Tetrazole as a Corrosion Inhibitor,” Corrosion Science, Vol. 50, No. 12, 2008, pp. 3439-3445. doi:10.1016/j.corsci.2008.10.002 [15] T. Arslan, F. Kandemirli, E. E. Ebenso, I. Love and H. Alemu, “Quantum Chemical Studies on the Corrosion In- hibition of Some Sulphonamides on Mild Steel in Acidic Medium,” Corrosion Science, Vol. 51, No. 1, January 2009, pp. 35-47. doi:10.1016/j.corsci.2008.10.016 [16] M. J. Bahrami, S. M. A. Hosseini and P. Pilvar, “Experi- mental and Theoretical Investigation of Organic Com- pounds as Inhibitors for Mild Steel Corrosion in Sulfuric Acid Medium,” Corrosion Science, Vol. 52, No. 9, Sep- tember 2010, pp. 2793-2803. [17] F. Kandemirli and S. Sagdinc, “Theoretical Study of Corrosion Inhibition of Amides and Thiosemicarba- zones,” Corrosion Science, Vol. 49, No. 5, May 2007, pp. 2118-2130. doi:10.1016/j.corsci.2006.10.026 [18] D. Wang, S. Li, Y. Ying, M. Wang, H. Xiao and Z. Chen, “Theoretical and Experimental Studies of Structure and Inhibition Efficiency of Imidazoline Derivatives,” Corro- sion Science, Vol. 41, No. 10, October 1999, pp. 1911- 1919. doi:10.1016/S0010-938X(99)00027-X [19] G. Bereket, C. Ogretir and A. Yurt, “Quantum Mechani- cal Calculations on Some 4-Methyl-5-Substituted Imida- zole Derivatives as Acidic Corrosion Inhibitor for Zinc,” Journal of Molecular Structure: THEOCHEM, Vol. 571, No. 1-3, August 2001, pp. 139-145. doi:10.1016/S0166-1280(01)00552-8 [20] N. Khalil, “Quantum Chemical Approach of Corrosion Inhibition,” Electrochimica Acta, Vol. 48, No. 18, August 2003, pp. 2635-2640. doi:10.1016/S0013-4686(03)00307-4 [21] J. Zhang, J. Liu, W. Yu, Y. Yan, L. You and L. Liu, “Molecular Modeling of the Inhibition Mechanism of 1-(2-Aminoethyl)-2-Alkyl-Imidazoline,” Corrosion Sci- ence, Vol. 52, No. 6, June 2010, pp. 2059-2065. doi:10.1016/j.corsci.2010.02.018 [22] M. Lebrini, F. Bentiss, H. Vezin and M. Lagrenée, “The Inhibition of Mild Steel Corrosion in Acidic Solutions by 2,5-Bis(4-Pyridyl)-1,3,4-Thiadiazole: Structure-Activity Co- rrelation,” Corrosion Science, Vol. 48, No. 5, May 2006, pp. 1279-1291. doi:10.1016/j.corsci.2005.05.001 [23] H. Ashassi-Sorkhabi, B. Shaabani and D. Seifzadeh, “Ef- fect of Some Pyrimidinic Shciff Bases on the Corrosion of Mild Steel in Hydrochloric Acid Solution,” Electro- chimica Acta, Vol. 50, No. 16-17, May 2005, pp. 3446- 3452. [24] J. J. Shie and J. M. Fang, “Direct Conversion of Alde- hydes to Amides, Tetrazoles, and Triazines in Aqueous Media by One-Pot Tandem Reactions,” Journal of Or- ganic Chemistry, Vol. 68, No. 3, 2003, pp. 1158-1160. doi:10.1021/jo026407z Copyright © 2011 SciRes. MSA
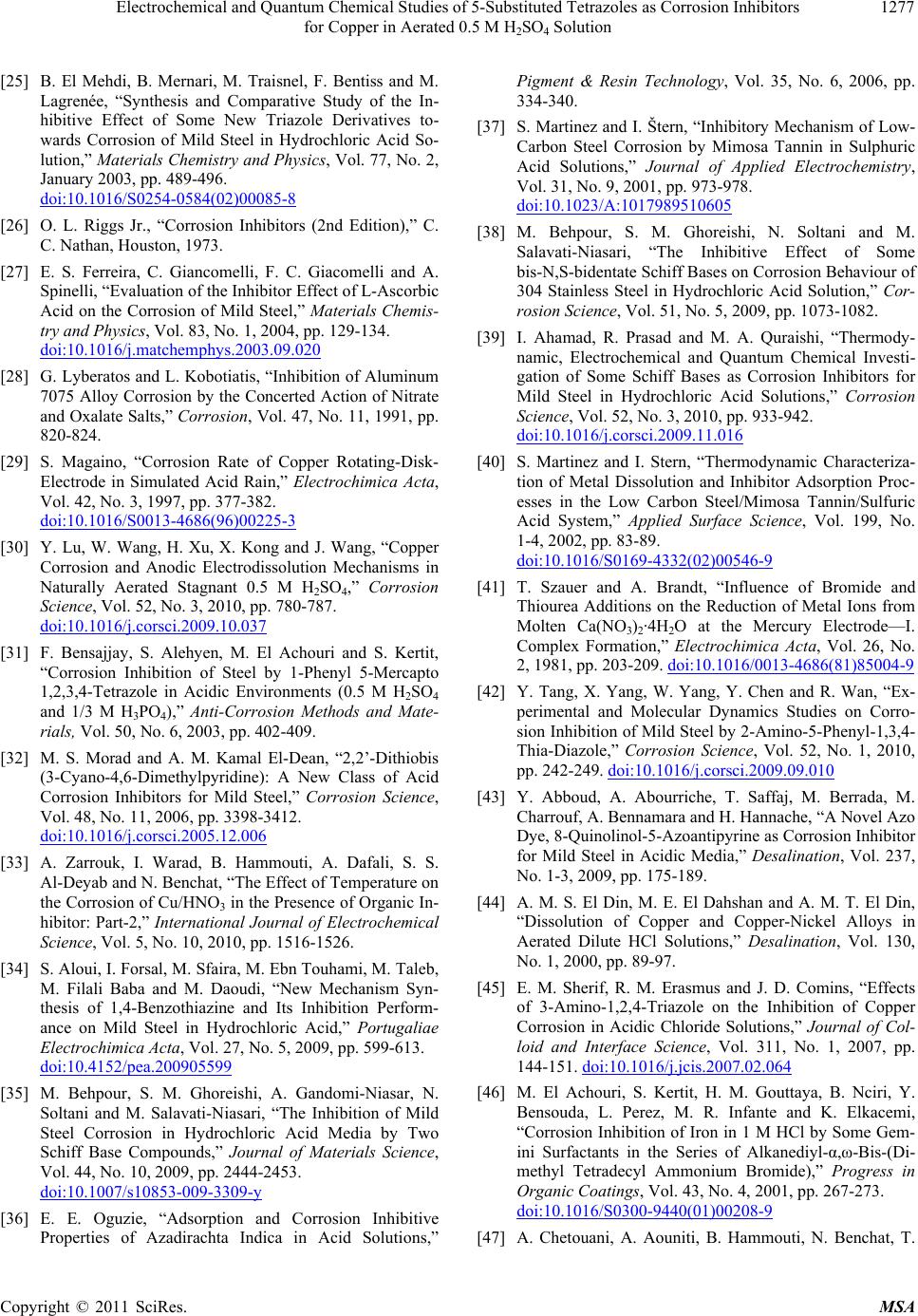 Electrochemical and Quantum Chemical Studies of 5-Substituted Tetrazoles as Corrosion Inhibitors 1277 for Copper in Aerated 0.5 M H2SO4 Solution [25] B. El Mehdi, B. Mernari, M. Traisnel, F. Bentiss and M. Lagrenée, “Synthesis and Comparative Study of the In- hibitive Effect of Some New Triazole Derivatives to- wards Corrosion of Mild Steel in Hydrochloric Acid So- lution,” Materials Chemistry and Physics, Vol. 77, No. 2, January 2003, pp. 489-496. doi:10.1016/S0254-0584(02)00085-8 [26] O. L. Riggs Jr., “Corrosion Inhibitors (2nd Edition),” C. C. Nathan, Houston, 1973. [27] E. S. Ferreira, C. Giancomelli, F. C. Giacomelli and A. Spinelli, “Evaluation of the Inhibitor Effect of L-Ascorbic Acid on the Corrosion of Mild Steel,” Materials Chemis- try and Physics, Vol. 83, No. 1, 2004, pp. 129-134. doi:10.1016/j.matchemphys.2003.09.020 [28] G. Lyberatos and L. Kobotiatis, “Inhibition of Aluminum 7075 Alloy Corrosion by the Concerted Action of Nitrate and Oxalate Salts,” Corrosion, Vol. 47, No. 11, 1991, pp. 820-824. [29] S. Magaino, “Corrosion Rate of Copper Rotating-Disk- Electrode in Simulated Acid Rain,” Electrochimica Acta, Vol. 42, No. 3, 1997, pp. 377-382. doi:10.1016/S0013-4686(96)00225-3 [30] Y. Lu, W. Wang, H. Xu, X. Kong and J. Wang, “Copper Corrosion and Anodic Electrodissolution Mechanisms in Naturally Aerated Stagnant 0.5 M H2SO4,” Corrosion Science, Vol. 52, No. 3, 2010, pp. 780-787. doi:10.1016/j.corsci.2009.10.037 [31] F. Bensajjay, S. Alehyen, M. El Achouri and S. Kertit, “Corrosion Inhibition of Steel by 1-Phenyl 5-Mercapto 1,2,3,4-Tetrazole in Acidic Environments (0.5 M H2SO4 and 1/3 M H3PO4),” Anti-Corrosion Methods and Mate- rials, Vol. 50, No. 6, 2003, pp. 402-409. [32] M. S. Morad and A. M. Kamal El-Dean, “2,2’-Dithiobis (3-Cyano-4,6-Dimethylpyridine): A New Class of Acid Corrosion Inhibitors for Mild Steel,” Corrosion Science, Vol. 48, No. 11, 2006, pp. 3398-3412. doi:10.1016/j.corsci.2005.12.006 [33] A. Zarrouk, I. Warad, B. Hammouti, A. Dafali, S. S. Al-Deyab and N. Benchat, “The Effect of Temperature on the Corrosion of Cu/HNO3 in the Presence of Organic In- hibitor: Part-2,” International Journal of Electrochemical Science, Vol. 5, No. 10, 2010, pp. 1516-1526. [34] S. Aloui, I. Forsal, M. Sfaira, M. Ebn Touhami, M. Taleb, M. Filali Baba and M. Daoudi, “New Mechanism Syn- thesis of 1,4-Benzothiazine and Its Inhibition Perform- ance on Mild Steel in Hydrochloric Acid,” Portugaliae Electrochimica Acta, Vol. 27, No. 5, 2009, pp. 599-613. doi:10.4152/pea.200905599 [35] M. Behpour, S. M. Ghoreishi, A. Gandomi-Niasar, N. Soltani and M. Salavati-Niasari, “The Inhibition of Mild Steel Corrosion in Hydrochloric Acid Media by Two Schiff Base Compounds,” Journal of Materials Science, Vol. 44, No. 10, 2009, pp. 2444-2453. doi:10.1007/s10853-009-3309-y [36] E. E. Oguzie, “Adsorption and Corrosion Inhibitive Properties of Azadirachta Indica in Acid Solutions,” Pigment & Resin Technology, Vol. 35, No. 6, 2006, pp. 334-340. [37] S. Martinez and I. Štern, “Inhibitory Mechanism of Low- Carbon Steel Corrosion by Mimosa Tannin in Sulphuric Acid Solutions,” Journal of Applied Electrochemistry, Vol. 31, No. 9, 2001, pp. 973-978. doi:10.1023/A:1017989510605 [38] M. Behpour, S. M. Ghoreishi, N. Soltani and M. Salavati-Niasari, “The Inhibitive Effect of Some bis-N,S-bidentate Schiff Bases on Corrosion Behaviour of 304 Stainless Steel in Hydrochloric Acid Solution,” Cor- rosion Science, Vol. 51, No. 5, 2009, pp. 1073-1082. [39] I. Ahamad, R. Prasad and M. A. Quraishi, “Thermody- namic, Electrochemical and Quantum Chemical Investi- gation of Some Schiff Bases as Corrosion Inhibitors for Mild Steel in Hydrochloric Acid Solutions,” Corrosion Science, Vol. 52, No. 3, 2010, pp. 933-942. doi:10.1016/j.corsci.2009.11.016 [40] S. Martinez and I. Stern, “Thermodynamic Characteriza- tion of Metal Dissolution and Inhibitor Adsorption Proc- esses in the Low Carbon Steel/Mimosa Tannin/Sulfuric Acid System,” Applied Surface Science, Vol. 199, No. 1-4, 2002, pp. 83-89. doi:10.1016/S0169-4332(02)00546-9 [41] T. Szauer and A. Brandt, “Influence of Bromide and Thiourea Additions on the Reduction of Metal Ions from Molten Ca(NO3)2·4H2O at the Mercury Electrode—I. Complex Formation,” Electrochimica Acta, Vol. 26, No. 2, 1981, pp. 203-209. doi:10.1016/0013-4686(81)85004-9 [42] Y. Tang, X. Yang, W. Yang, Y. Chen and R. Wan, “Ex- perimental and Molecular Dynamics Studies on Corro- sion Inhibition of Mild Steel by 2-Amino-5-Phenyl-1,3,4- Thia-Diazole,” Corrosion Science, Vol. 52, No. 1, 2010, pp. 242-249. doi:10.1016/j.corsci.2009.09.010 [43] Y. Abboud, A. Abourriche, T. Saffaj, M. Berrada, M. Charrouf, A. Bennamara and H. Hannache, “A Novel Azo Dye, 8-Quinolinol-5-Azoantipyrine as Corrosion Inhibitor for Mild Steel in Acidic Media,” Desalination, Vol. 237, No. 1-3, 2009, pp. 175-189. [44] A. M. S. El Din, M. E. El Dahshan and A. M. T. El Din, “Dissolution of Copper and Copper-Nickel Alloys in Aerated Dilute HCl Solutions,” Desalination, Vol. 130, No. 1, 2000, pp. 89-97. [45] E. M. Sherif, R. M. Erasmus and J. D. Comins, “Effects of 3-Amino-1,2,4-Triazole on the Inhibition of Copper Corrosion in Acidic Chloride Solutions,” Journal of Col- loid and Interface Science, Vol. 311, No. 1, 2007, pp. 144-151. doi:10.1016/j.jcis.2007.02.064 [46] M. El Achouri, S. Kertit, H. M. Gouttaya, B. Nciri, Y. Bensouda, L. Perez, M. R. Infante and K. Elkacemi, “Corrosion Inhibition of Iron in 1 M HCl by Some Gem- ini Surfactants in the Series of Alkanediyl-α,ω-Bis-(Di- methyl Tetradecyl Ammonium Bromide),” Progress in Organic Coatings, Vol. 43, No. 4, 2001, pp. 267-273. doi:10.1016/S0300-9440(01)00208-9 [47] A. Chetouani, A. Aouniti, B. Hammouti, N. Benchat, T. Copyright © 2011 SciRes. MSA
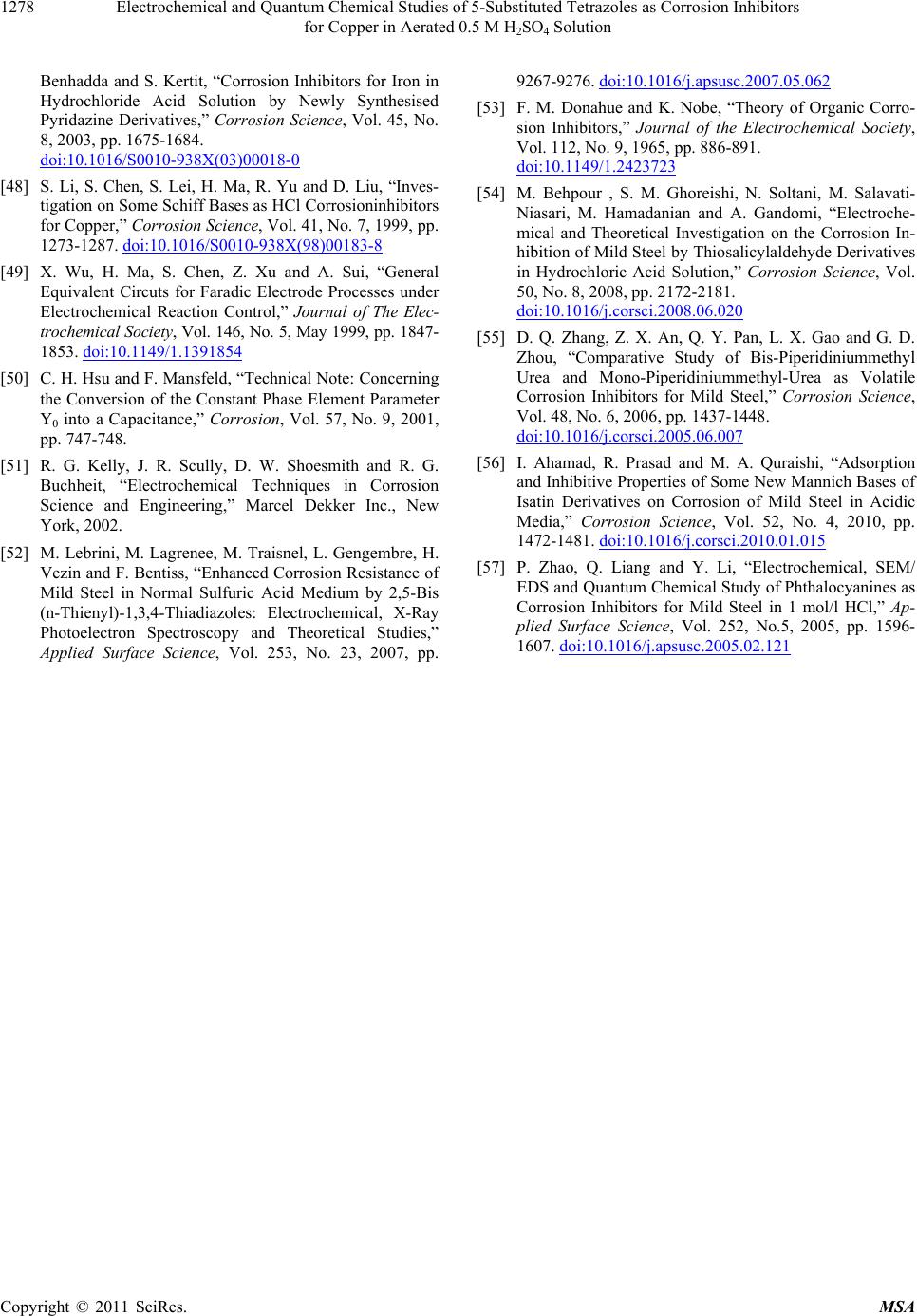 Electrochemical and Quantum Chemical Studies of 5-Substituted Tetrazoles as Corrosion Inhibitors for Copper in Aerated 0.5 M H2SO4 Solution Copyright © 2011 SciRes. MSA 1278 Benhadda and S. Kertit, “Corrosion Inhibitors for Iron in Hydrochloride Acid Solution by Newly Synthesised Pyridazine Derivatives,” Corrosion Science, Vol. 45, No. 8, 2003, pp. 1675-1684. doi:10.1016/S0010-938X(03)00018-0 [48] S. Li, S. Chen, S. Lei, H. Ma, R. Yu and D. Liu, “Inves- tigation on Some Schiff Bases as HCl Corrosioninhibitors for Copper,” Corrosion Science, Vol. 41, No. 7, 1999, pp. 1273-1287. doi:10.1016/S0010-938X(98)00183-8 [49] X. Wu, H. Ma, S. Chen, Z. Xu and A. Sui, “General Equivalent Circuts for Faradic Electrode Processes under Electrochemical Reaction Control,” Journal of The Elec- trochemical Society, Vol. 146, No. 5, May 1999, pp. 1847- 1853. doi:10.1149/1.1391854 [50] C. H. Hsu and F. Mansfeld, “Technical Note: Concerning the Conversion of the Constant Phase Element Parameter Y0 into a Capacitance,” Corrosion, Vol. 57, No. 9, 2001, pp. 747-748. [51] R. G. Kelly, J. R. Scully, D. W. Shoesmith and R. G. Buchheit, “Electrochemical Techniques in Corrosion Science and Engineering,” Marcel Dekker Inc., New York, 2002. [52] M. Lebrini, M. Lagrenee, M. Traisnel, L. Gengembre, H. Vezin and F. Bentiss, “Enhanced Corrosion Resistance of Mild Steel in Normal Sulfuric Acid Medium by 2,5-Bis (n-Thienyl)-1,3,4-Thiadiazoles: Electrochemical, X-Ray Photoelectron Spectroscopy and Theoretical Studies,” Applied Surface Science, Vol. 253, No. 23, 2007, pp. 9267-9276. doi:10.1016/j.apsusc.2007.05.062 [53] F. M. Donahue and K. Nobe, “Theory of Organic Corro- sion Inhibitors,” Journal of the Electrochemical Society, Vol. 112, No. 9, 1965, pp. 886-891. doi:10.1149/1.2423723 [54] M. Behpour , S. M. Ghoreishi, N. Soltani, M. Salavati- Niasari, M. Hamadanian and A. Gandomi, “Electroche- mical and Theoretical Investigation on the Corrosion In- hibition of Mild Steel by Thiosalicylaldehyde Derivatives in Hydrochloric Acid Solution,” Corrosion Science, Vol. 50, No. 8, 2008, pp. 2172-2181. doi:10.1016/j.corsci.2008.06.020 [55] D. Q. Zhang, Z. X. An, Q. Y. Pan, L. X. Gao and G. D. Zhou, “Comparative Study of Bis-Piperidiniummethyl Urea and Mono-Piperidiniummethyl-Urea as Volatile Corrosion Inhibitors for Mild Steel,” Corrosion Science, Vol. 48, No. 6, 2006, pp. 1437-1448. doi:10.1016/j.corsci.2005.06.007 [56] I. Ahamad, R. Prasad and M. A. Quraishi, “Adsorption and Inhibitive Properties of Some New Mannich Bases of Isatin Derivatives on Corrosion of Mild Steel in Acidic Media,” Corrosion Science, Vol. 52, No. 4, 2010, pp. 1472-1481. doi:10.1016/j.corsci.2010.01.015 [57] P. Zhao, Q. Liang and Y. Li, “Electrochemical, SEM/ EDS and Quantum Chemical Study of Phthalocyanines as Corrosion Inhibitors for Mild Steel in 1 mol/l HCl,” Ap- plied Surface Science, Vol. 252, No.5, 2005, pp. 1596- 1607. doi:10.1016/j.apsusc.2005.02.121
|