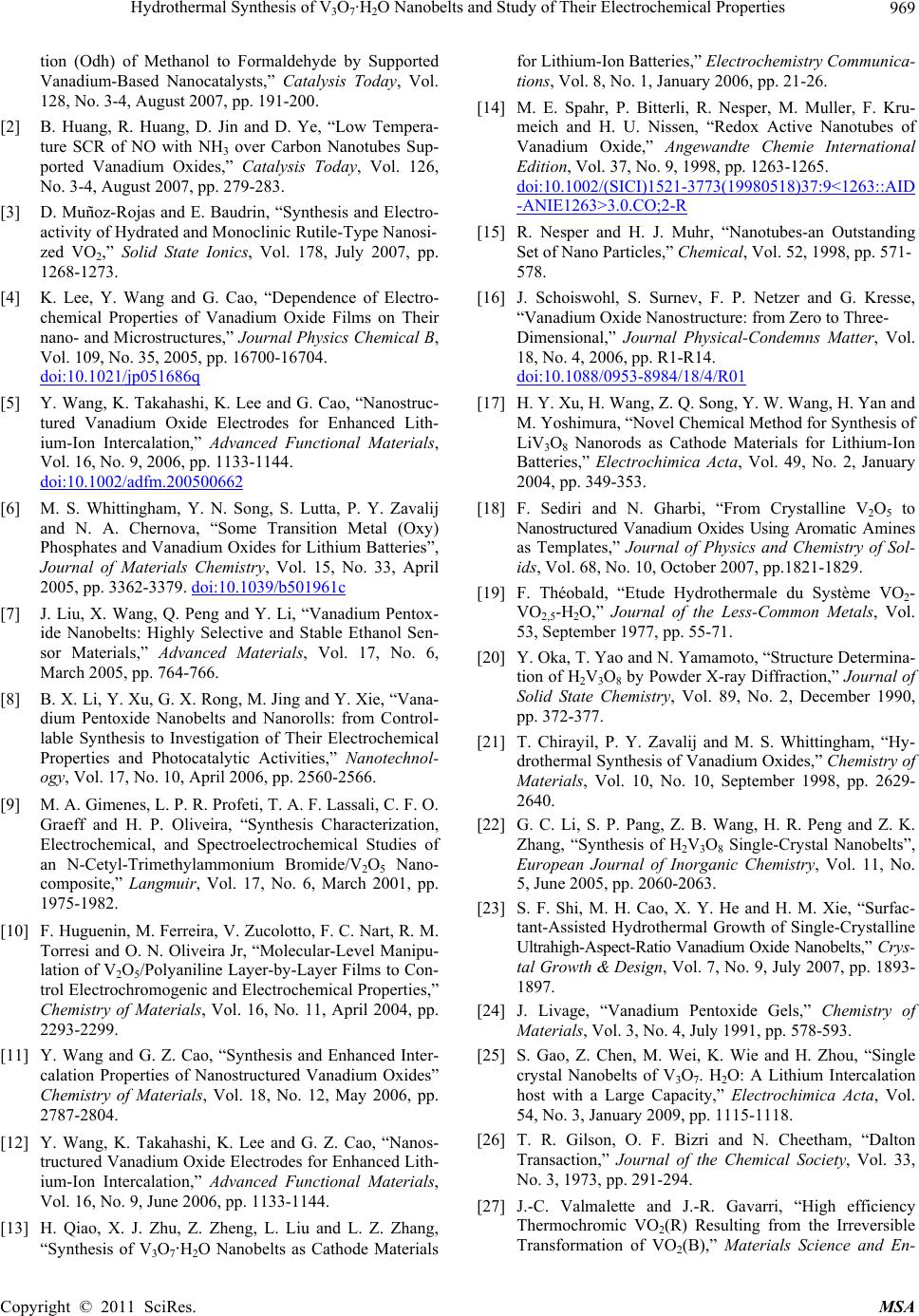
Hydrothermal Synthesis of VO·H O Nanobelts and Study of Their Electrochemical Properties969
3 72
tion (Odh) of Methanol to Formaldehyde by Supported
Vanadium-Based Nanocatalysts,” Catalysis Today, Vol.
128, No. 3-4, August 2007, pp. 191-200.
[2] B. Huang, R. Huang, D. Jin and D. Ye, “Low Tempera-
ture SCR of NO with NH3 over Carbon Nanotubes Sup-
ported Vanadium Oxides,” Catalysis Today, Vol. 126,
No. 3-4, August 2007, pp. 279-283.
[3] D. Muñoz-Rojas and E. Baudrin, “Synthesis and Electro-
activity of Hydrated and Monoclinic Rutile-Type Nanosi-
zed VO2,” Solid State Ionics, Vol. 178, July 2007, pp.
1268-1273.
[4] K. Lee, Y. Wang and G. Cao, “Dependence of Electro-
chemical Properties of Vanadium Oxide Films on Their
nano- and Microstructures,” Journal Physics Chemical B,
Vol. 109, No. 35, 2005, pp. 16700-16704.
doi:10.1021/jp051686q
[5] Y. Wang, K. Takahashi, K. Lee and G. Cao, “Nanostruc-
tured Vanadium Oxide Electrodes for Enhanced Lith-
ium-Ion Intercalation,” Advanced Functional Materials,
Vol. 16, No. 9, 2006, pp. 1133-1144.
doi:10.1002/adfm.200500662
[6] M. S. Whittingham, Y. N. Song, S. Lutta, P. Y. Zavalij
and N. A. Chernova, “Some Transition Metal (Oxy)
Phosphates and Vanadium Oxides for Lithium Batteries”,
Journal of Materials Chemistry, Vol. 15, No. 33, April
2005, pp. 3362-3379. doi:10.1039/b501961c
[7] J. Liu, X. Wang, Q. Peng and Y. Li, “Vanadium Pentox-
ide Nanobelts: Highly Selective and Stable Ethanol Sen-
sor Materials,” Advanced Materials, Vol. 17, No. 6,
March 2005, pp. 764-766.
[8] B. X. Li, Y. Xu, G. X. Rong, M. Jing and Y. Xie, “Vana-
dium Pentoxide Nanobelts and Nanorolls: from Control-
lable Synthesis to Investigation of Their Electrochemical
Properties and Photocatalytic Activities,” Nanotechnol-
ogy, Vol. 17, No. 10, April 2006, pp. 2560-2566.
[9] M. A. Gimenes, L. P. R. Profeti, T. A. F. Lassali, C. F. O.
Graeff and H. P. Oliveira, “Synthesis Characterization,
Electrochemical, and Spectroelectrochemical Studies of
an N-Cetyl-Trimethylammonium Bromide/V2O5 Nano-
composite,” Langmuir, Vol. 17, No. 6, March 2001, pp.
1975-1982.
[10] F. Huguenin, M. Ferreira, V. Zucolotto, F. C. Nart, R. M.
Torresi and O. N. Oliveira Jr, “Molecular-Level Manipu-
lation of V2O5/Polyaniline Layer-by-Layer Films to Con-
trol Electrochromogenic and Electrochemical Properties,”
Chemistry of Materials, Vol. 16, No. 11, April 2004, pp.
2293-2299.
[11] Y. Wang and G. Z. Cao, “Synthesis and Enhanced Inter-
calation Properties of Nanostructured Vanadium Oxides”
Chemistry of Materials, Vol. 18, No. 12, May 2006, pp.
2787-2804.
[12] Y. Wang, K. Takahashi, K. Lee and G. Z. Cao, “Nanos-
tructured Vanadium Oxide Electrodes for Enhanced Lith-
ium-Ion Intercalation,” Advanced Functional Materials,
Vol. 16, No. 9, June 2006, pp. 1133-1144.
[13] H. Qiao, X. J. Zhu, Z. Zheng, L. Liu and L. Z. Zhang,
“Synthesis of V3O7·H2O Nanobelts as Cathode Materials
for Lithium-Ion Batteries,” Electrochemistry Communica-
tions, Vol. 8, No. 1, January 2006, pp. 21-26.
[14] M. E. Spahr, P. Bitterli, R. Nesper, M. Muller, F. Kru-
meich and H. U. Nissen, “Redox Active Nanotubes of
Vanadium Oxide,” Angewandte Chemie International
Edition, Vol. 37, No. 9, 1998, pp. 1263-1265.
doi:10.1002/(SICI)1521-3773(19980518)37:9<1263::AID
-ANIE1263>3.0.CO;2-R
[15] R. Nesper and H. J. Muhr, “Nanotubes-an Outstanding
Set of Nano Particles,” Chemical, Vol. 52, 1998, pp. 571-
578.
[16] J. Schoiswohl, S. Surnev, F. P. Netzer and G. Kresse,
“Vanadium Oxide Nanostructure: from Zero to Three-
Dimensional,” Journal Physical-Condemns Matter, Vol.
18, No. 4, 2006, pp. R1-R14.
doi:10.1088/0953-8984/18/4/R01
[17] H. Y. Xu, H. Wang, Z. Q. Song, Y. W. Wang, H. Yan and
M. Yoshimura, “Novel Chemical Method for Synthesis of
LiV3O8 Nanorods as Cathode Materials for Lithium-Ion
Batteries,” Electrochimica Acta, Vol. 49, No. 2, January
2004, pp. 349-353.
[18] F. Sediri and N. Gharbi, “From Crystalline V2O5 to
Nanostructured Vanadium Oxides Using Aromatic Amines
as Templates,” Journal of Physics and Chemistry of Sol-
ids, Vol. 68, No. 10, October 2007, pp.1821-1829.
[19] F. Théobald, “Etude Hydrothermale du Système VO2-
VO2,5-H2O,” Journal of the Less-Common Metals, Vol.
53, September 1977, pp. 55-71.
[20] Y. Oka, T. Yao and N. Yamamoto, “Structure Determina-
tion of H2V3O8 by Powder X-ray Diffraction,” Journal of
Solid State Chemistry, Vol. 89, No. 2, December 1990,
pp. 372-377.
[21] T. Chirayil, P. Y. Zavalij and M. S. Whittingham, “Hy-
drothermal Synthesis of Vanadium Oxides,” Chemistry of
Materials, Vol. 10, No. 10, September 1998, pp. 2629-
2640.
[22] G. C. Li, S. P. Pang, Z. B. Wang, H. R. Peng and Z. K.
Zhang, “Synthesis of H2V3O8 Single-Crystal Nanobelts”,
European Journal of Inorganic Chemistry, Vol. 11, No.
5, June 2005, pp. 2060-2063.
[23] S. F. Shi, M. H. Cao, X. Y. He and H. M. Xie, “Surfac-
tant-Assisted Hydrothermal Growth of Single-Crystalline
Ultrahigh-Aspect-Ratio Vanadium Oxide Nanobelts,” Crys-
tal Growth & Design, Vol. 7, No. 9, July 2007, pp. 1893-
1897.
[24] J. Livage, “Vanadium Pentoxide Gels,” Chemistry of
Materials, Vol. 3, No. 4, July 1991, pp. 578-593.
[25] S. Gao, Z. Chen, M. Wei, K. Wie and H. Zhou, “Single
crystal Nanobelts of V3O7. H2O: A Lithium Intercalation
host with a Large Capacity,” Electrochimica Acta, Vol.
54, No. 3, January 2009, pp. 1115-1118.
[26] T. R. Gilson, O. F. Bizri and N. Cheetham, “Dalton
Transaction,” Journal of the Chemical Society, Vol. 33,
No. 3, 1973, pp. 291-294.
[27] J.-C. Valmalette and J.-R. Gavarri, “High efficiency
Thermochromic VO2(R) Resulting from the Irreversible
Transformation of VO2(B),” Materials Science and En-
Copyright © 2011 SciRes. MSA