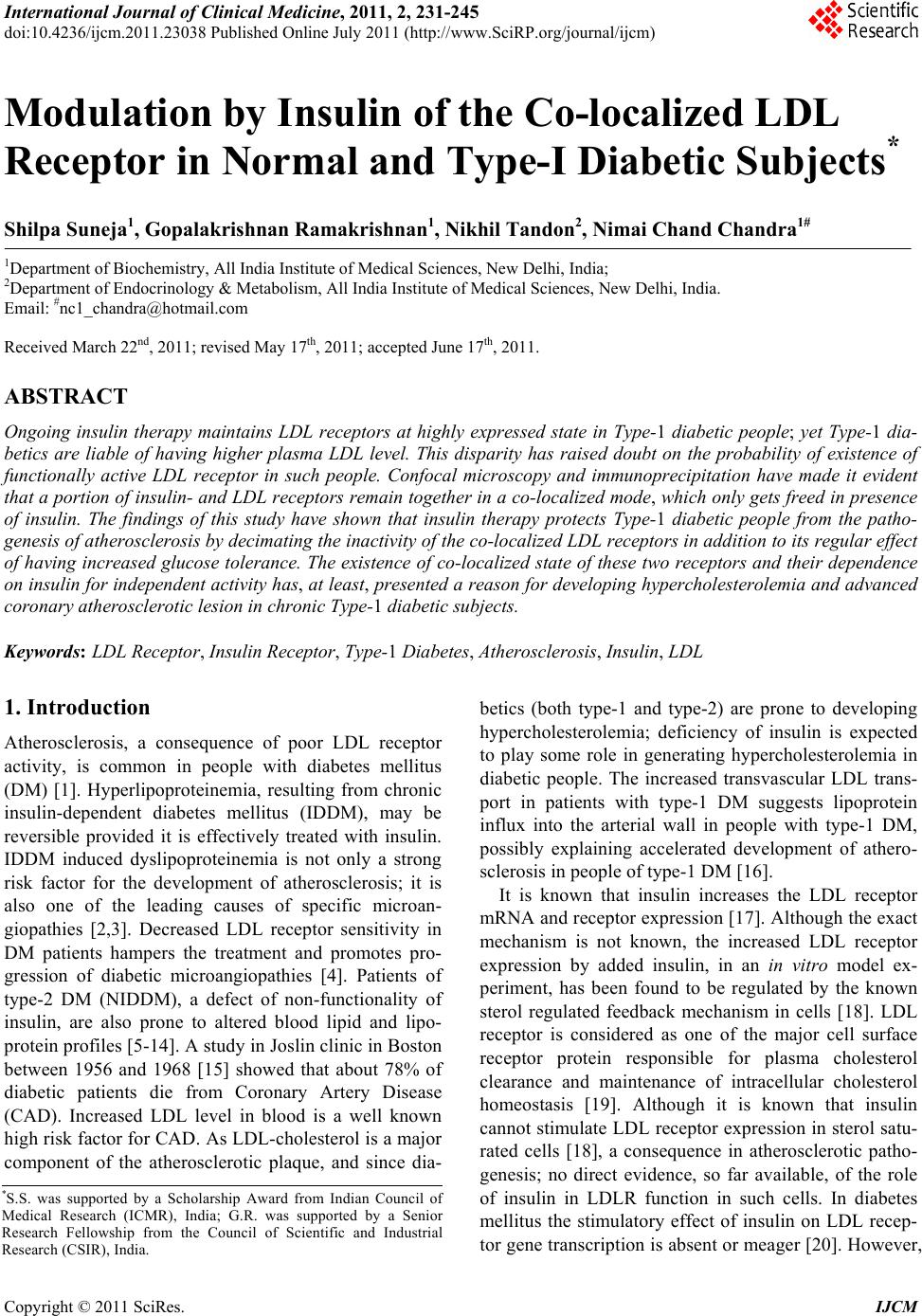 International Journal of Clinical Medicine, 2011, 2, 231-245 doi:10.4236/ijcm.2011.23038 Published Online July 2011 (http://www.SciRP.org/journal/ijcm) Copyright © 2011 SciRes. IJCM 231 Modulation by Insulin of the Co-localized LDL Receptor in Normal and Type-I Diabetic Subjects* Shilpa Suneja1, Gopalakrishnan Ramakrishnan1, Nikhil Tandon2, Nimai Chand Chandra1# 1Department of Biochemistry, All India Institute of Medical Sciences, New Delhi, India; 2Department of Endocrinology & Metabolism, All India Institute of Medical Sciences, New Delhi, India. Email: #nc1_chandra@hotmail.com Received March 22nd, 2011; revised May 17th, 2011; accepted June 17th, 2011. ABSTRACT Ongoing insulin therapy maintains LDL receptors at highly expressed state in Type-1 diabetic people; yet Type-1 dia- betics are liable of having higher plasma LDL level. This disparity has raised doubt on the probability of existence of functionally active LDL receptor in such people. Confocal microscopy and immunoprecipitation have made it evident that a portion of insulin- and LDL receptors remain together in a co-localized mode, which only gets freed in presence of insulin. The findings of this study have shown that insulin therapy protects Type-1 diabetic people from the patho- genesis of atherosclerosis by decimating the inactivity of the co-localized LDL receptors in addition to its regular effect of having increased glucose tolerance. The existence of co-localized state of these two receptors and their dependence on insulin for independent activity has, at least, presented a reason for developing hypercholesterolemia and advanced coronary atherosclerotic lesion in chronic Type-1 diabetic subjects. Keywords: LDL Receptor, Insulin Receptor, Type-1 Diabetes, Atherosclerosis, Insulin, LDL 1. Introduction Atherosclerosis, a consequence of poor LDL receptor activity, is common in people with diabetes mellitus (DM) [1]. Hyperlipoproteinemia, resulting from chronic insulin-dependent diabetes mellitus (IDDM), may be reversible provided it is effectively treated with insulin. IDDM induced dyslipoproteinemia is not only a strong risk factor for the development of atherosclerosis; it is also one of the leading causes of specific microan- giopathies [2,3]. Decreased LDL receptor sensitivity in DM patients hampers the treatment and promotes pro- gression of diabetic microangiopathies [4]. Patients of type-2 DM (NIDDM), a defect of non-functionality of insulin, are also prone to altered blood lipid and lipo- protein profiles [5-14]. A study in Joslin clinic in Boston between 1956 and 1968 [15] showed that about 78% of diabetic patients die from Coronary Artery Disease (CAD). Increased LDL level in blood is a well known high risk factor for CAD. As LDL-cholesterol is a major component of the atherosclerotic plaque, and since dia- betics (both type-1 and type-2) are prone to developing hypercholesterolemia; deficiency of insulin is expected to play some role in generating hypercholesterolemia in diabetic people. The increased transvascular LDL trans- port in patients with type-1 DM suggests lipoprotein influx into the arterial wall in people with type-1 DM, possibly explaining accelerated development of athero- sclerosis in people of type-1 DM [16]. It is known that insulin increases the LDL receptor mRNA and receptor expression [17]. Although the exact mechanism is not known, the increased LDL receptor expression by added insulin, in an in vitro model ex- periment, has been found to be regulated by the known sterol regulated feedback mechanism in cells [18]. LDL receptor is considered as one of the major cell surface receptor protein responsible for plasma cholesterol clearance and maintenance of intracellular cholesterol homeostasis [19]. Although it is known that insulin cannot stimulate LDL receptor expression in sterol satu- rated cells [18], a consequence in atherosclerotic patho- genesis; no direct evidence, so far available, of the role of insulin in LDLR function in such cells. In diabetes mellitus the stimulatory effect of insulin on LDL recep- tor gene transcription is absent or meager [20]. However, *S.S. was supported by a Scholarship Award from Indian Council o Medical Research (ICMR), India; G.R. was supported by a Senio Research Fellowship from the Council of Scientific and Industrial Research (CSIR), India.
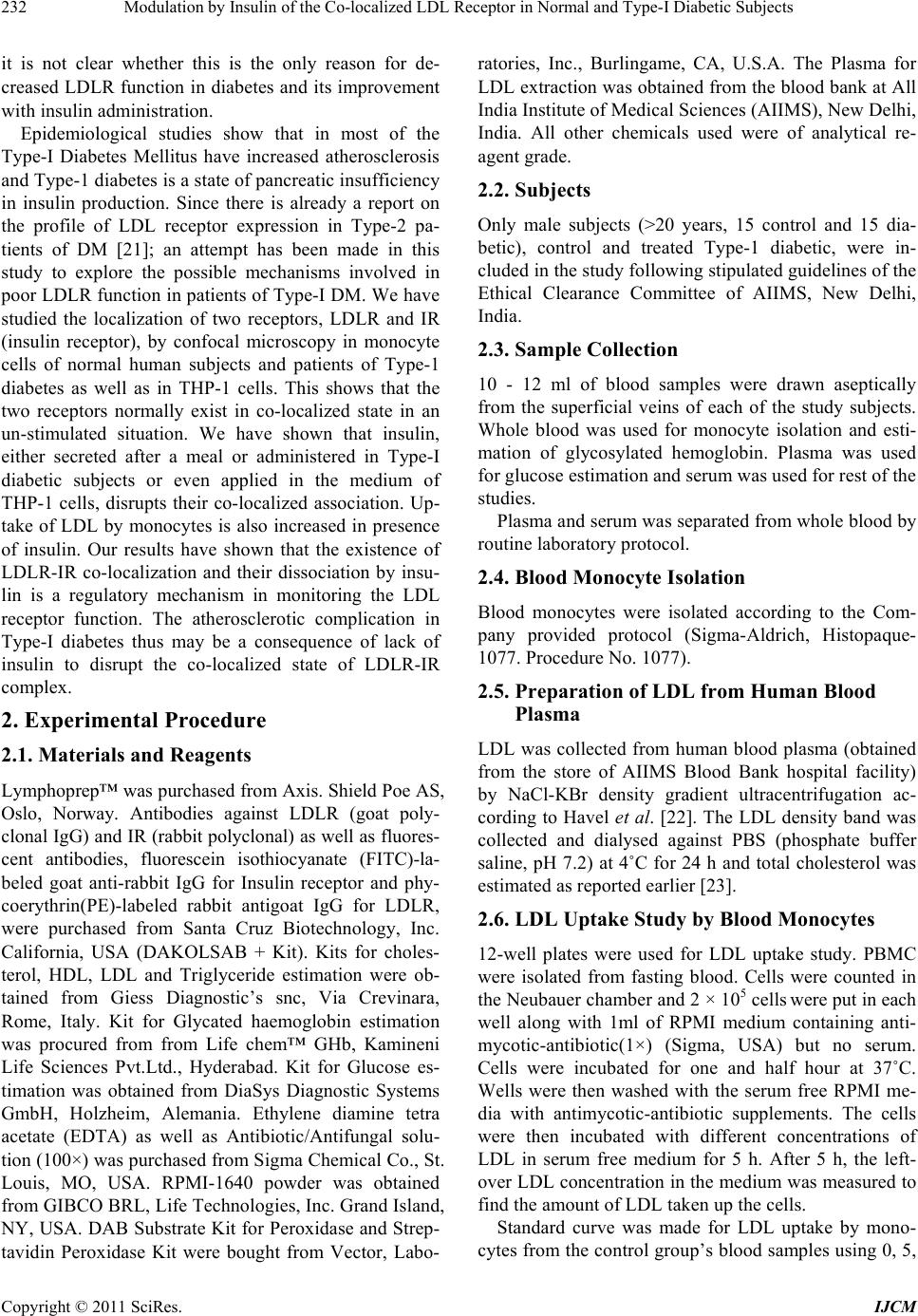 Modulation by Insulin of the Co-localized LDL Receptor in Normal and Type-I Diabetic Subjects 232 it is not clear whether this is the only reason for de- creased LDLR function in diabetes and its improvement with insulin administration. Epidemiological studies show that in most of the Type-I Diabetes Mellitus have increased atherosclerosis and Type-1 diabetes is a state of pancreatic insufficiency in insulin production. Since there is already a report on the profile of LDL receptor expression in Type-2 pa- tients of DM [21]; an attempt has been made in this study to explore the possible mechanisms involved in poor LDLR function in patients of Type-I DM. We have studied the localization of two receptors, LDLR and IR (insulin receptor), by confocal microscopy in monocyte cells of normal human subjects and patients of Type-1 diabetes as well as in THP-1 cells. This shows that the two receptors normally exist in co-localized state in an un-stimulated situation. We have shown that insulin, either secreted after a meal or administered in Type-I diabetic subjects or even applied in the medium of THP-1 cells, disrupts their co-localized association. Up- take of LDL by monocytes is also increased in presence of insulin. Our results have shown that the existence of LDLR-IR co-localization and their dissociation by insu- lin is a regulatory mechanism in monitoring the LDL receptor function. The atherosclerotic complication in Type-I diabetes thus may be a consequence of lack of insulin to disrupt the co-localized state of LDLR-IR complex. 2. Experimental Procedure 2.1. Materials and Reagents Lymphoprep™ was purchased from Axis. Shield Poe AS, Oslo, Norway. Antibodies against LDLR (goat poly- clonal IgG) and IR (rabbit polyclonal) as well as fluores- cent antibodies, fluorescein isothiocyanate (FITC)-la- beled goat anti-rabbit IgG for Insulin receptor and phy- coerythrin(PE)-labeled rabbit antigoat IgG for LDLR, were purchased from Santa Cruz Biotechnology, Inc. California, USA (DAKOLSAB + Kit). Kits for choles- terol, HDL, LDL and Triglyceride estimation were ob- tained from Giess Diagnostic’s snc, Via Crevinara, Rome, Italy. Kit for Glycated haemoglobin estimation was procured from from Life chem™ GHb, Kamineni Life Sciences Pvt.Ltd., Hyderabad. Kit for Glucose es- timation was obtained from DiaSys Diagnostic Systems GmbH, Holzheim, Alemania. Ethylene diamine tetra acetate (EDTA) as well as Antibiotic/Antifungal solu- tion (100×) was purchased from Sigma Chemical Co., St. Louis, MO, USA. RPMI-1640 powder was obtained from GIBCO BRL, Life Technologies, Inc. Grand Island, NY, USA. DAB Substrate Kit for Peroxidase and Strep- tavidin Peroxidase Kit were bought from Vector, Labo- ratories, Inc., Burlingame, CA, U.S.A. The Plasma for LDL extraction was obtained from the blood bank at All India Institute of Medical Sciences (AIIMS), New Delhi, India. All other chemicals used were of analytical re- agent grade. 2.2. Subjects Only male subjects (>20 years, 15 control and 15 dia- betic), control and treated Type-1 diabetic, were in- cluded in the study following stipulated guidelines of the Ethical Clearance Committee of AIIMS, New Delhi, India. 2.3. Sample Collection 10 - 12 ml of blood samples were drawn aseptically from the superficial veins of each of the study subjects. Whole blood was used for monocyte isolation and esti- mation of glycosylated hemoglobin. Plasma was used for glucose estimation and serum was used for rest of the studies. Plasma and serum was separated from whole blood by routine laboratory protocol. 2.4. Blood Monocyte Isolation Blood monocytes were isolated according to the Com- pany provided protocol (Sigma-Aldrich, Histopaque- 1077. Procedure No. 1077). 2.5. Preparation of LDL from Human Blood Plasma LDL was collected from human blood plasma (obtained from the store of AIIMS Blood Bank hospital facility) by NaCl-KBr density gradient ultracentrifugation ac- cording to Havel et al. [22]. The LDL density band was collected and dialysed against PBS (phosphate buffer saline, pH 7.2) at 4˚C for 24 h and total cholesterol was estimated as reported earlier [23]. 2.6. LDL Uptake Study by Blood Monocytes 12-well plates were used for LDL uptake study. PBMC were isolated from fasting blood. Cells were counted in the Neubauer chamber and 2 × 105 cells were put in each well along with 1ml of RPMI medium containing anti- mycotic-antibiotic(1×) (Sigma, USA) but no serum. Cells were incubated for one and half hour at 37˚C. Wells were then washed with the serum free RPMI me- dia with antimycotic-antibiotic supplements. The cells were then incubated with different concentrations of LDL in serum free medium for 5 h. After 5 h, the left- over LDL concentration in the medium was measured to find the amount of LDL taken up the cells. Standard curve was made for LDL uptake by mono- cytes from the control group’s blood samples using 0, 5, Copyright © 2011 SciRes. IJCM
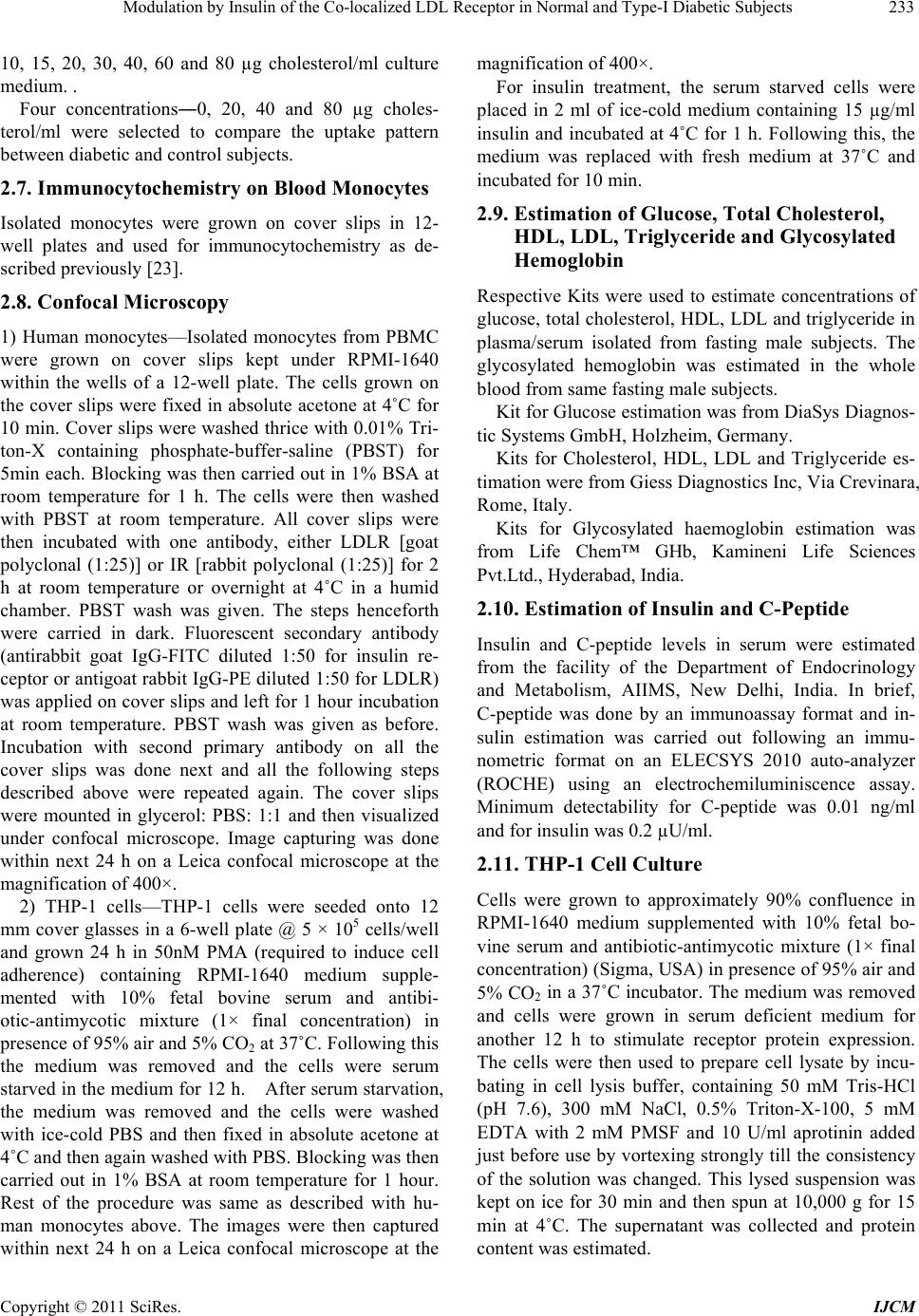 Modulation by Insulin of the Co-localized LDL Receptor in Normal and Type-I Diabetic Subjects233 10, 15, 20, 30, 40, 60 and 80 µg cholesterol/ml culture medium. . Four concentrations―0, 20, 40 and 80 µg choles- terol/ml were selected to compare the uptake pattern between diabetic and control subjects. 2.7. Immunocytochemistry on Blood Monocytes Isolated monocytes were grown on cover slips in 12- well plates and used for immunocytochemistry as de- scribed previously [23]. 2.8. Confocal Microscopy 1) Human monocytes—Isolated monocytes from PBMC were grown on cover slips kept under RPMI-1640 within the wells of a 12-well plate. The cells grown on the cover slips were fixed in absolute acetone at 4˚C for 10 min. Cover slips were washed thrice with 0.01% Tri- ton-X containing phosphate-buffer-saline (PBST) for 5min each. Blocking was then carried out in 1% BSA at room temperature for 1 h. The cells were then washed with PBST at room temperature. All cover slips were then incubated with one antibody, either LDLR [goat polyclonal (1:25)] or IR [rabbit polyclonal (1:25)] for 2 h at room temperature or overnight at 4˚C in a humid chamber. PBST wash was given. The steps henceforth were carried in dark. Fluorescent secondary antibody (antirabbit goat IgG-FITC diluted 1:50 for insulin re- ceptor or antigoat rabbit IgG-PE diluted 1:50 for LDLR) was applied on cover slips and left for 1 hour incubation at room temperature. PBST wash was given as before. Incubation with second primary antibody on all the cover slips was done next and all the following steps described above were repeated again. The cover slips were mounted in glycerol: PBS: 1:1 and then visualized under confocal microscope. Image capturing was done within next 24 h on a Leica confocal microscope at the magnification of 400×. 2) THP-1 cells—THP-1 cells were seeded onto 12 mm cover glasses in a 6-well plate @ 5 × 105 cells/well and grown 24 h in 50nM PMA (required to induce cell adherence) containing RPMI-1640 medium supple- mented with 10% fetal bovine serum and antibi- otic-antimycotic mixture (1× final concentration) in presence of 95% air and 5% CO2 at 37˚C. Following this the medium was removed and the cells were serum starved in the medium for 12 h. After serum starvation, the medium was removed and the cells were washed with ice-cold PBS and then fixed in absolute acetone at 4˚C and then again washed with PBS. Blocking was then carried out in 1% BSA at room temperature for 1 hour. Rest of the procedure was same as described with hu- man monocytes above. The images were then captured within next 24 h on a Leica confocal microscope at the magnification of 400×. For insulin treatment, the serum starved cells were placed in 2 ml of ice-cold medium containing 15 µg/ml insulin and incubated at 4˚C for 1 h. Following this, the medium was replaced with fresh medium at 37˚C and incubated for 10 min. 2.9. Estimation of Glucose, Total Cholesterol, HDL, LDL, Triglyceride and Glycosylated Hemoglobin Respective Kits were used to estimate concentrations of glucose, total cholesterol, HDL, LDL and triglyceride in plasma/serum isolated from fasting male subjects. The glycosylated hemoglobin was estimated in the whole blood from same fasting male subjects. Kit for Glucose estimation was from DiaSys Diagnos- tic Systems GmbH, Holzheim, Germany. Kits for Cholesterol, HDL, LDL and Triglyceride es- timation were from Giess Diagnostics Inc, Via Crevinara, Rome, Italy. Kits for Glycosylated haemoglobin estimation was from Life Chem™ GHb, Kamineni Life Sciences Pvt.Ltd., Hyderabad, India. 2.10. Estimation of Insulin and C-Peptide Insulin and C-peptide levels in serum were estimated from the facility of the Department of Endocrinology and Metabolism, AIIMS, New Delhi, India. In brief, C-peptide was done by an immunoassay format and in- sulin estimation was carried out following an immu- nometric format on an ELECSYS 2010 auto-analyzer (ROCHE) using an electrochemiluminiscence assay. Minimum detectability for C-peptide was 0.01 ng/ml and for insulin was 0.2 µU/ml. 2.11. THP-1 Cell Culture Cells were grown to approximately 90% confluence in RPMI-1640 medium supplemented with 10% fetal bo- vine serum and antibiotic-antimycotic mixture (1× final concentration) (Sigma, USA) in presence of 95% air and 5% CO2 in a 37˚C incubator. The medium was removed and cells were grown in serum deficient medium for another 12 h to stimulate receptor protein expression. The cells were then used to prepare cell lysate by incu- bating in cell lysis buffer, containing 50 mM Tris-HCl (pH 7.6), 300 mM NaCl, 0.5% Triton-X-100, 5 mM EDTA with 2 mM PMSF and 10 U/ml aprotinin added just before use by vortexing strongly till the consistency of the solution was changed. This lysed suspension was kept on ice for 30 min and then spun at 10,000 g for 15 min at 4˚C. The supernatant was collected and protein content was estimated. Copyright © 2011 SciRes. IJCM
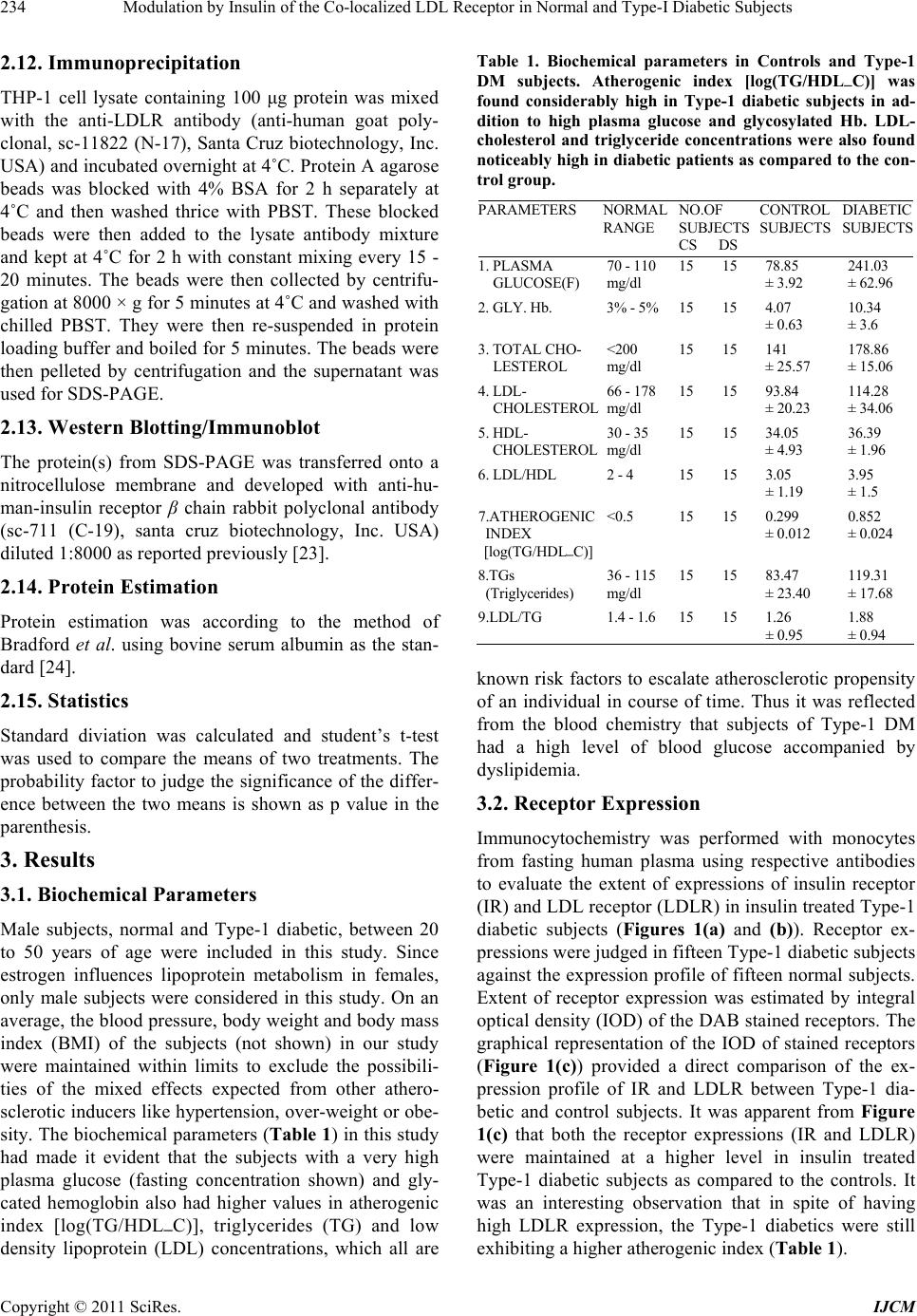 Modulation by Insulin of the Co-localized LDL Receptor in Normal and Type-I Diabetic Subjects 234 2.12. Immunoprecipitation THP-1 cell lysate containing 100 μg protein was mixed with the anti-LDLR antibody (anti-human goat poly- clonal, sc-11822 (N-17), Santa Cruz biotechnology, Inc. USA) and incubated overnight at 4˚C. Protein A agarose beads was blocked with 4% BSA for 2 h separately at 4˚C and then washed thrice with PBST. These blocked beads were then added to the lysate antibody mixture and kept at 4˚C for 2 h with constant mixing every 15 - 20 minutes. The beads were then collected by centrifu- gation at 8000 × g for 5 minutes at 4˚C and washed with chilled PBST. They were then re-suspended in protein loading buffer and boiled for 5 minutes. The beads were then pelleted by centrifugation and the supernatant was used for SDS-PAGE. 2.13. Western Blotting/Immunoblot The protein(s) from SDS-PAGE was transferred onto a nitrocellulose membrane and developed with anti-hu- man-insulin receptor β chain rabbit polyclonal antibody (sc-711 (C-19), santa cruz biotechnology, Inc. USA) diluted 1:8000 as reported previously [23]. 2.14. Protein Estimation Protein estimation was according to the method of Bradford et al. using bovine serum albumin as the stan- dard [24]. 2.15. Statistics Standard diviation was calculated and student’s t-test was used to compare the means of two treatments. The probability factor to judge the significance of the differ- ence between the two means is shown as p value in the parenthesis. 3. Results 3.1. Biochemical Parameters Male subjects, normal and Type-1 diabetic, between 20 to 50 years of age were included in this study. Since estrogen influences lipoprotein metabolism in females, only male subjects were considered in this study. On an average, the blood pressure, body weight and body mass index (BMI) of the subjects (not shown) in our study were maintained within limits to exclude the possibili- ties of the mixed effects expected from other athero- sclerotic inducers like hypertension, over-weight or obe- sity. The biochemical parameters (Table 1) in this study had made it evident that the subjects with a very high plasma glucose (fasting concentration shown) and gly- cated hemoglobin also had higher values in atherogenic index [log(TG/HDL─C)], triglycerides (TG) and low density lipoprotein (LDL) concentrations, which all are Table 1. Biochemical parameters in Controls and Type-1 DM subjects. Atherogenic index [log(TG/HDL─C)] was found considerably high in Type-1 diabetic subjects in ad- dition to high plasma glucose and glycosylated Hb. LDL- cholesterol and triglyceride concentrations were also found noticeably high in diabetic patients as compared to the con- trol group. known risk factors to escalate atherosclerotic propensity of an individual in course of time. Thus it was reflected from the blood chemistry that subjects of Type-1 DM had a high level of blood glucose accompanied by dyslipidemia. 3.2. Receptor Expression Immunocytochemistry was performed with monocytes from fasting human plasma using respective antibodies to evaluate the extent of expressions of insulin receptor (IR) and LDL receptor (LDLR) in insulin treated Type-1 diabetic subjects (Figures 1(a) and (b)). Receptor ex- pressions were judged in fifteen Type-1 diabetic subjects against the expression profile of fifteen normal subjects. Extent of receptor expression was estimated by integral optical density (IOD) of the DAB stained receptors. The graphical representation of the IOD of stained receptors (Figure 1(c)) provided a direct comparison of the ex- pression profile of IR and LDLR between Type-1 dia- betic and control subjects. It was apparent from Figure 1(c) that both the receptor expressions (IR and LDLR) were maintained at a higher level in insulin treated Type-1 diabetic subjects as compared to the controls. It was an interesting observation that in spite of having high LDLR expression, the Type-1 diabetics were still exhibiting a higher atherogenic index (Table 1). PARAMETERS NORMAL RANGE NO.OF SUBJECTS CS DS CONTROL SUBJECTS DIABETIC SUBJECTS 1. PLASMA GLUCOSE(F) 70 - 110 mg/dl 15 15 78.85 ± 3.92 241.03 ± 62.96 2. GLY. Hb. 3% - 5% 15 15 4.07 ± 0.63 10.34 ± 3.6 3. TOTAL CHO- LESTEROL <200 mg/dl 15 15 141 ± 25.57 178.86 ± 15.06 4. LDL- CHOLESTEROL 66 - 178 mg/dl 15 15 93.84 ± 20.23 114.28 ± 34.06 5. HDL- CHOLESTEROL 30 - 35 mg/dl 15 15 34.05 ± 4.93 36.39 ± 1.96 6. LDL/HDL 2 - 4 15 15 3.05 ± 1.19 3.95 ± 1.5 7.ATHEROGENIC INDEX [log(TG/HDL─C)] <0.5 15 15 0.299 ± 0.012 0.852 ± 0.024 8.TGs (Triglycerides) 36 - 115 mg/dl 15 15 83.47 ± 23.40 119.31 ± 17.68 9.LDL/TG 1.4 - 1.6 15 15 1.26 ± 0.95 1.88 ± 0.94 Copyright © 2011 SciRes. IJCM
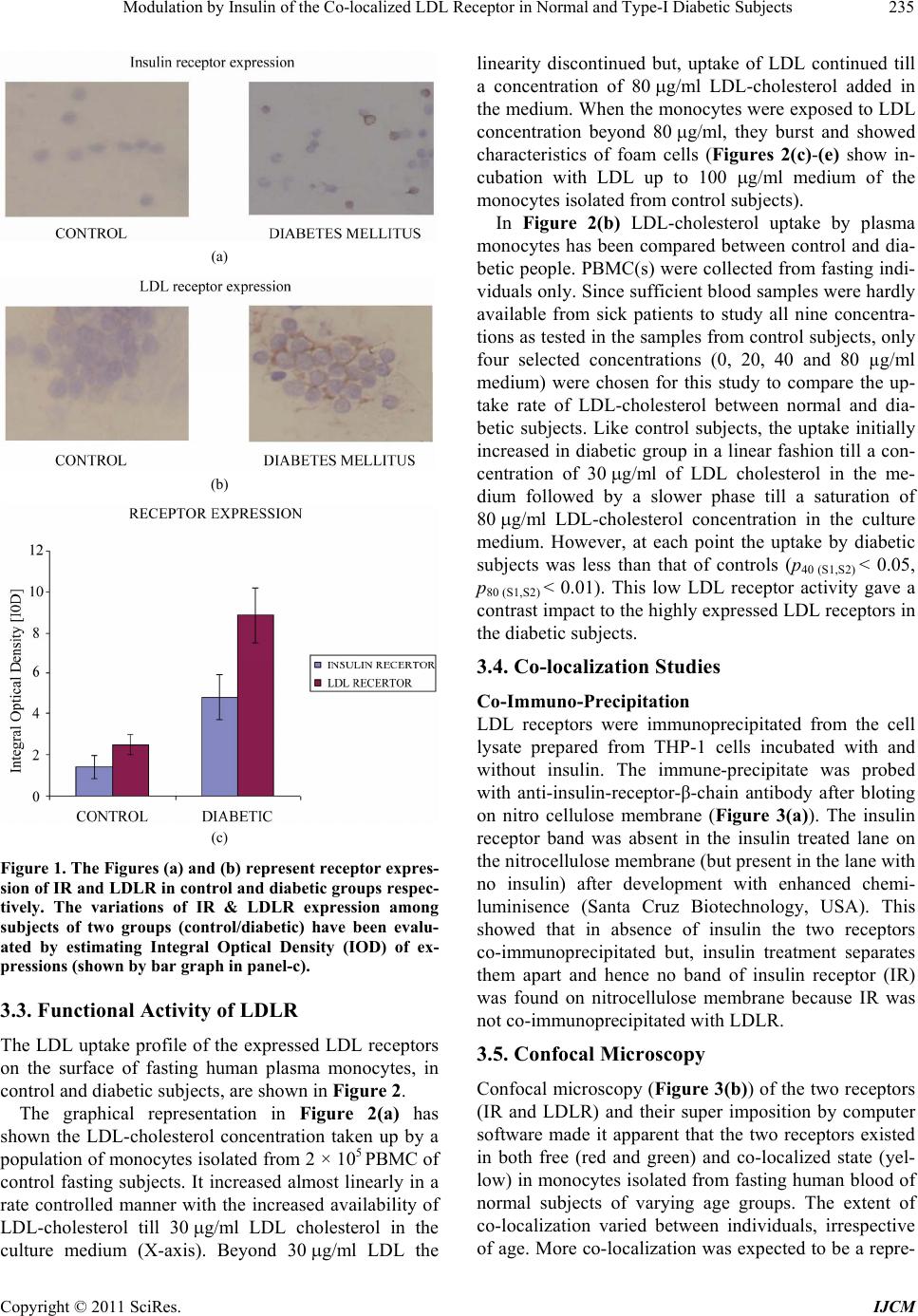 Modulation by Insulin of the Co-localized LDL Receptor in Normal and Type-I Diabetic Subjects235 (a) (b) (c) Figure 1. The Figures (a) and (b) represent receptor expres- sion of IR and LDLR in control and diabetic groups respec- tively. The variations of IR & LDLR expression among subjects of two groups (control/diabetic) have been evalu- ated by estimating Integral Optical Density (IOD) of ex- pressions (shown by bar graph in panel-c). 3.3. Functional Activity of LDLR The LDL uptake profile of the expressed LDL receptors on the surface of fasting human plasma monocytes, in control and diabetic subjects, are shown in Figure 2. The graphical representation in Figure 2(a) has shown the LDL-cholesterol concentration taken up by a population of monocytes isolated from 2 × 105 PBMC of control fasting subjects. It increased almost linearly in a rate controlled manner with the increased availability of LDL-cholesterol till g/ml LDL cholesterol in the culture medium (X-axis). Beyond g/ml LDL the linearity discontinued but, uptake of LDL continued till a concentration of g/ml LDL-cholesterol added in the medium. When the monocytes were exposed to LDL concentration beyond g/ml, they burst and showed characteristics of foam cells (Figures 2(c)-(e) show in- cubation with LDL up to 100 g/ml medium of the monocytes isolated from control subjects). In Figure 2(b) LDL-cholesterol uptake by plasma monocytes has been compared between control and dia- betic people. PBMC(s) were collected from fasting indi- viduals only. Since sufficient blood samples were hardly available from sick patients to study all nine concentra- tions as tested in the samples from control subjects, only four selected concentrations (0, 20, 40 and 80 µg/ml medium) were chosen for this study to compare the up- take rate of LDL-cholesterol between normal and dia- betic subjects. Like control subjects, the uptake initially increased in diabetic group in a linear fashion till a con- centration of g/ml of LDL cholesterol in the me- dium followed by a slower phase till a saturation of g/ml LDL-cholesterol concentration in the culture medium. However, at each point the uptake by diabetic subjects was less than that of controls (p40 (S1,S2) < 0.05, p80 (S1,S2) < 0.01). This low LDL receptor activity gave a contrast impact to the highly expressed LDL receptors in the diabetic subjects. 3.4. Co-localization Studies Co-Immuno-Precipitation LDL receptors were immunoprecipitated from the cell lysate prepared from THP-1 cells incubated with and without insulin. The immune-precipitate was probed with anti-insulin-receptor-β-chain antibody after bloting on nitro cellulose membrane (Figure 3(a)). The insulin receptor band was absent in the insulin treated lane on the nitrocellulose membrane (but present in the lane with no insulin) after development with enhanced chemi- luminisence (Santa Cruz Biotechnology, USA). This showed that in absence of insulin the two receptors co-immunoprecipitated but, insulin treatment separates them apart and hence no band of insulin receptor (IR) was found on nitrocellulose membrane because IR was not co-immunoprecipitated with LDLR. 3.5. Confocal Microscopy Confocal microscopy (Figure 3(b)) of the two receptors (IR and LDLR) and their super imposition by computer software made it apparent that the two receptors existed in both free (red and green) and co-localized state (yel- low) in monocytes isolated from fasting human blood of normal subjects of varying age groups. The extent of co-localization varied between individuals, irrespective of age. More co-localization was expected to be a repre- Copyright © 2011 SciRes. IJCM
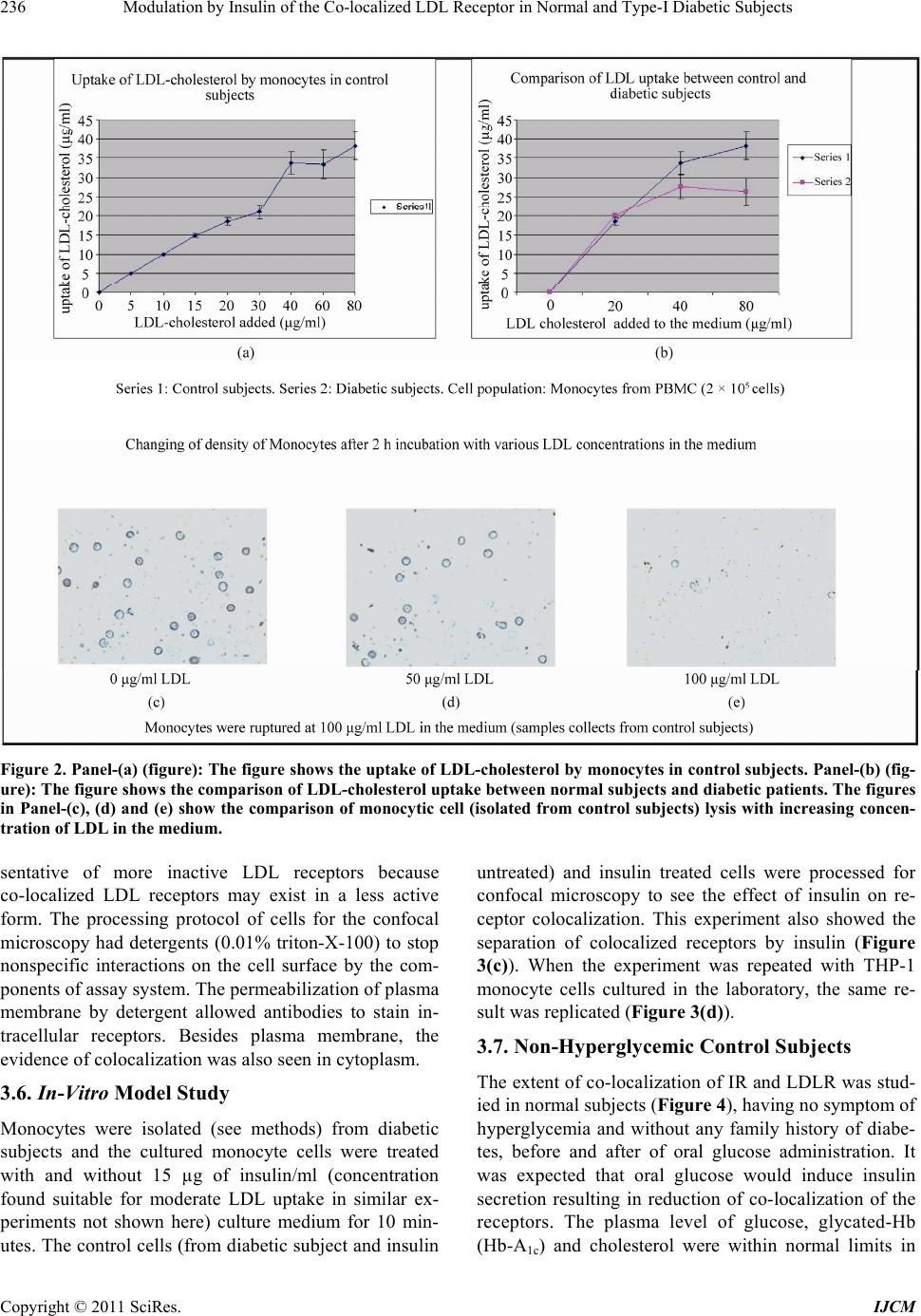 Modulation by Insulin of the Co-localized LDL Receptor in Normal and Type-I Diabetic Subjects Copyright © 2011 SciRes. IJCM 236 Figure 2. Panel-(a) (figure): The figure shows the uptake of LDL-cholesterol by monocytes in control subjects. Panel-(b) (fig- ure): The figure shows the comparison of LDL-cholesterol uptake between normal subjects and diabetic patients. The figures in Panel-(c), (d) and (e) show the comparison of monocytic cell (isolated from control subjects) lysis with increasing concen- tration of LDL in the medium. sentative of more inactive LDL receptors because co-localized LDL receptors may exist in a less active form. The processing protocol of cells for the confocal microscopy had detergents (0.01% triton-X-100) to stop nonspecific interactions on the cell surface by the com- ponents of assay system. The permeabilization of plasma membrane by detergent allowed antibodies to stain in- tracellular receptors. Besides plasma membrane, the evidence of colocalization was also seen in cytoplasm. 3.6. In-Vitro Model Study Monocytes were isolated (see methods) from diabetic subjects and the cultured monocyte cells were treated with and without 15 µg of insulin/ml (concentration found suitable for moderate LDL uptake in similar ex- periments not shown here) culture medium for 10 min- utes. The control cells (from diabetic subject and insulin untreated) and insulin treated cells were processed for confocal microscopy to see the effect of insulin on re- ceptor colocalization. This experiment also showed the separation of colocalized receptors by insulin (Figure 3(c)). When the experiment was repeated with THP-1 monocyte cells cultured in the laboratory, the same re- sult was replicated (Figure 3(d)). 3.7. Non-Hyperglycemic Control Subjects The extent of co-localization of IR and LDLR was stud- ied in normal subjects (Figure 4), having no symptom of hyperglycemia and without any family history of diabe- tes, before and after of oral glucose administration. It was expected that oral glucose would induce insulin secretion resulting in reduction of co-localization of the receptors. The plasma level of glucose, glycated-Hb (Hb-A1c) and cholesterol were within normal limits in
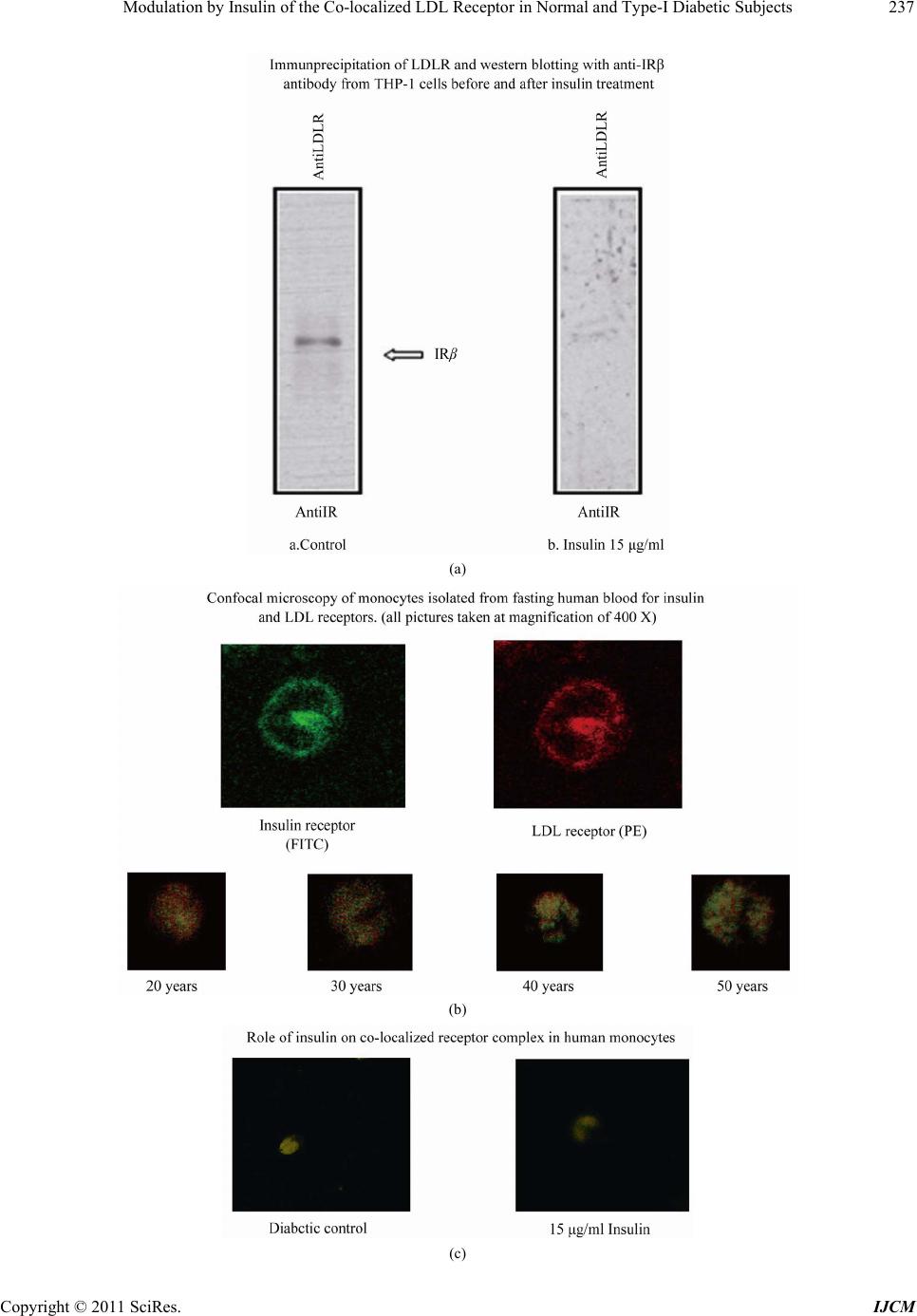 Modulation by Insulin of the Co-localized LDL Receptor in Normal and Type-I Diabetic Subjects237 (a) (b) (c) Copyright © 2011 SciRes. IJCM
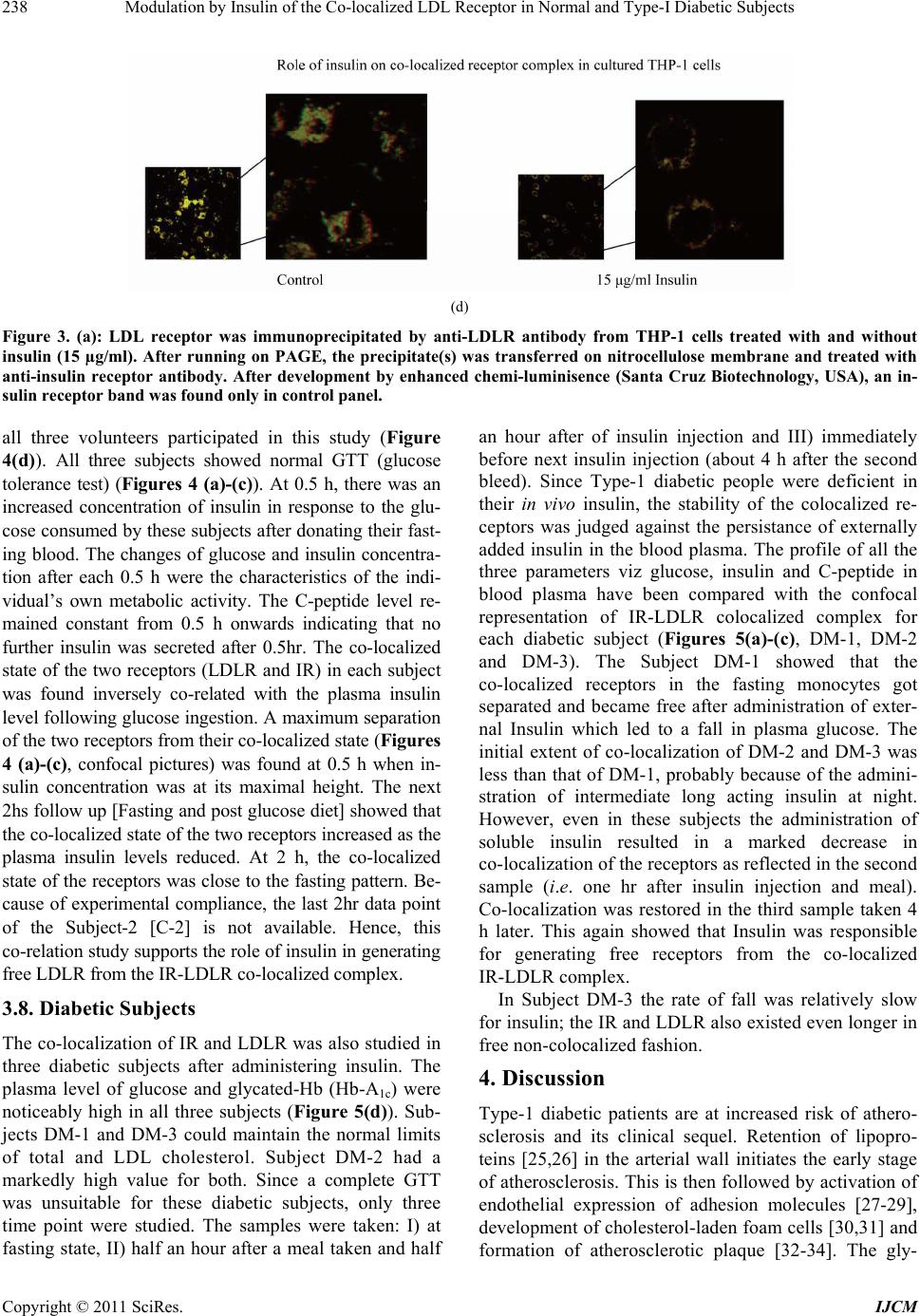 Modulation by Insulin of the Co-localized LDL Receptor in Normal and Type-I Diabetic Subjects 238 (d) Figure 3. (a): LDL receptor was immunoprecipitated by anti-LDLR antibody from THP-1 cells treated with and without insulin (15 µg/ml). After running on PAGE, the precipitate(s) was transferred on nitrocellulose membrane and treated with anti-insulin receptor antibody. After development by enhanced chemi-luminisence (Santa Cruz Biotechnology, USA), an in- sulin receptor band was found only in control panel. all three volunteers participated in this study (Figure 4(d)). All three subjects showed normal GTT (glucose tolerance test) (Figures 4 (a)-(c)). At 0.5 h, there was an increased concentration of insulin in response to the glu- cose consumed by these subjects after donating their fast- ing blood. The changes of glucose and insulin concentra- tion after each 0.5 h were the characteristics of the indi- vidual’s own metabolic activity. The C-peptide level re- mained constant from 0.5 h onwards indicating that no further insulin was secreted after 0.5hr. The co-localized state of the two receptors (LDLR and IR) in each subject was found inversely co-related with the plasma insulin level following glucose ingestion. A maximum separation of the two receptors from their co-localized state (Figures 4 (a)-(c), confocal pictures) was found at 0.5 h when in- sulin concentration was at its maximal height. The next 2hs follow up [Fasting and post glucose diet] showed that the co-localized state of the two receptors increased as the plasma insulin levels reduced. At 2 h, the co-localized state of the receptors was close to the fasting pattern. Be- cause of experimental compliance, the last 2hr data point of the Subject-2 [C-2] is not available. Hence, this co-relation study supports the role of insulin in generating free LDLR from the IR-LDLR co-localized complex. 3.8. Diabetic Subjects The co-localization of IR and LDLR was also studied in three diabetic subjects after administering insulin. The plasma level of glucose and glycated-Hb (Hb-A1c) were noticeably high in all three subjects (Figure 5(d)). Sub- jects DM-1 and DM-3 could maintain the normal limits of total and LDL cholesterol. Subject DM-2 had a markedly high value for both. Since a complete GTT was unsuitable for these diabetic subjects, only three time point were studied. The samples were taken: I) at fasting state, II) half an hour after a meal taken and half an hour after of insulin injection and III) immediately before next insulin injection (about 4 h after the second bleed). Since Type-1 diabetic people were deficient in their in vivo insulin, the stability of the colocalized re- ceptors was judged against the persistance of externally added insulin in the blood plasma. The profile of all the three parameters viz glucose, insulin and C-peptide in blood plasma have been compared with the confocal representation of IR-LDLR colocalized complex for each diabetic subject (Figures 5(a)-(c), DM-1, DM-2 and DM-3). The Subject DM-1 showed that the co-localized receptors in the fasting monocytes got separated and became free after administration of exter- nal Insulin which led to a fall in plasma glucose. The initial extent of co-localization of DM-2 and DM-3 was less than that of DM-1, probably because of the admini- stration of intermediate long acting insulin at night. However, even in these subjects the administration of soluble insulin resulted in a marked decrease in co-localization of the receptors as reflected in the second sample (i.e. one hr after insulin injection and meal). Co-localization was restored in the third sample taken 4 h later. This again showed that Insulin was responsible for generating free receptors from the co-localized IR-LDLR complex. In Subject DM-3 the rate of fall was relatively slow for insulin; the IR and LDLR also existed even longer in free non-colocalized fashion. 4. Discussion Type-1 diabetic patients are at increased risk of athero- sclerosis and its clinical sequel. Retention of lipopro- teins [25,26] in the arterial wall initiates the early stage of atherosclerosis. This is then followed by activation of endothelial expression of adhesion molecules [27-29], development of cholesterol-laden foam cells [30,31] and formation of atherosclerotic plaque [32-34]. The gly- Copyright © 2011 SciRes. IJCM
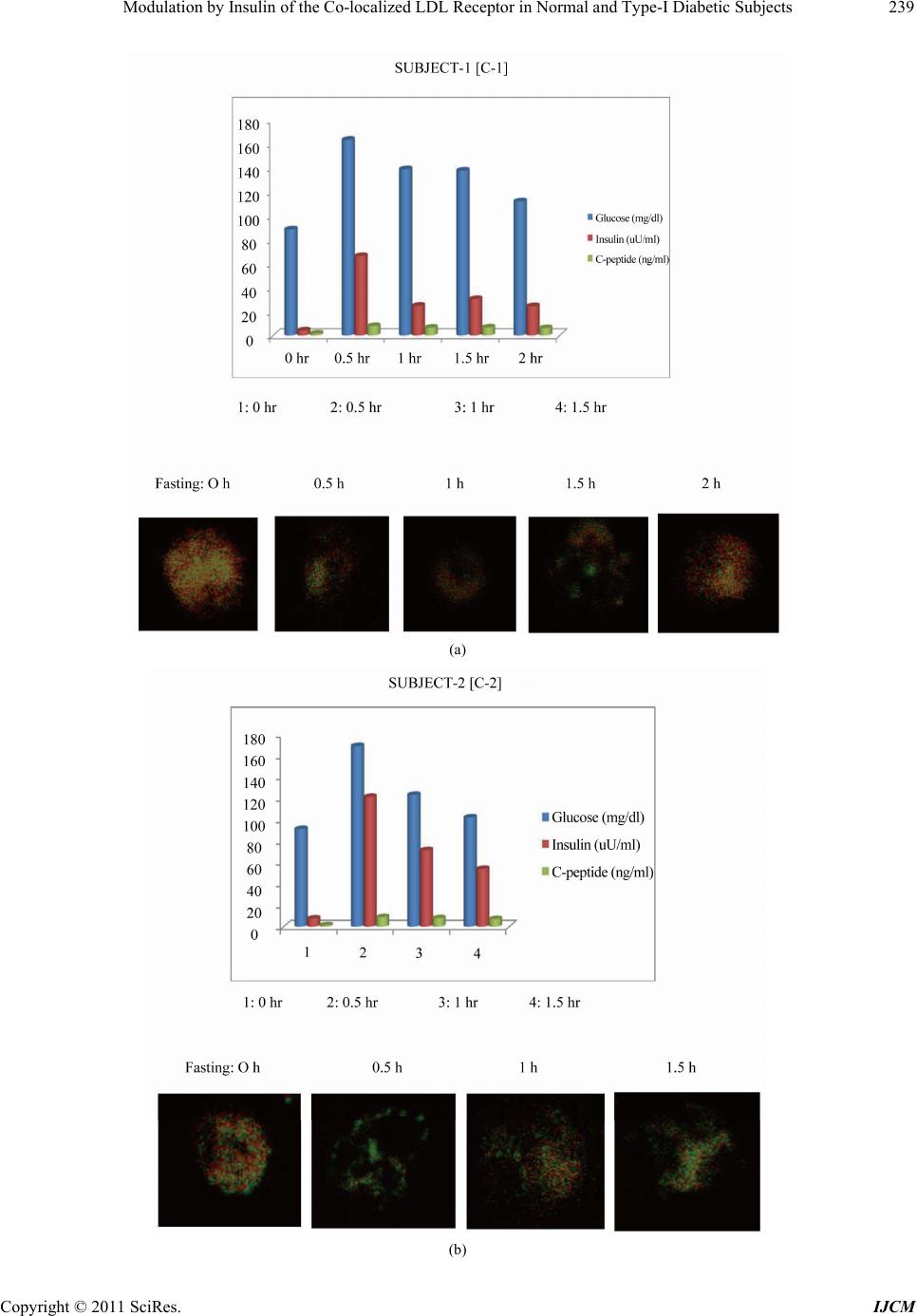 Modulation by Insulin of the Co-localized LDL Receptor in Normal and Type-I Diabetic Subjects239 (a) (b) Copyright © 2011 SciRes. IJCM
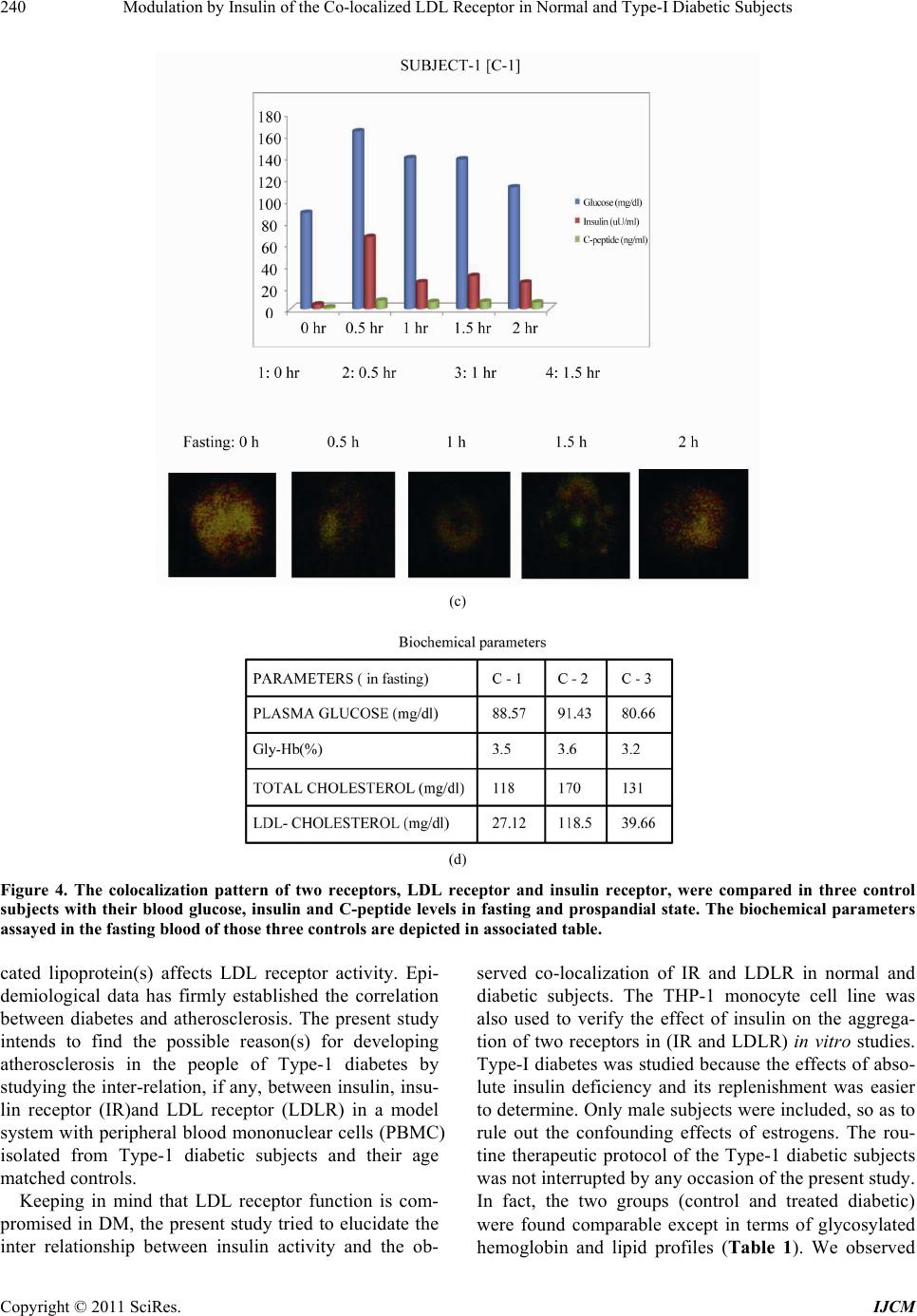 Modulation by Insulin of the Co-localized LDL Receptor in Normal and Type-I Diabetic Subjects 240 (c) (d) Figure 4. The colocalization pattern of two receptors, LDL receptor and insulin receptor, were compared in three control subjects with their blood glucose, insulin and C-peptide levels in fasting and prospandial state. The biochemical parameters assayed in the fasting blood of those three controls are depicted in associated table. cated lipoprotein(s) affects LDL receptor activity. Epi- demiological data has firmly established the correlation between diabetes and atherosclerosis. The present study intends to find the possible reason(s) for developing atherosclerosis in the people of Type-1 diabetes by studying the inter-relation, if any, between insulin, insu- lin receptor (IR)and LDL receptor (LDLR) in a model system with peripheral blood mononuclear cells (PBMC) isolated from Type-1 diabetic subjects and their age matched controls. Keeping in mind that LDL receptor function is com- promised in DM, the present study tried to elucidate the inter relationship between insulin activity and the ob- served co-localization of IR and LDLR in normal and diabetic subjects. The THP-1 monocyte cell line was also used to verify the effect of insulin on the aggrega- tion of two receptors in (IR and LDLR) in vitro studies. Type-I diabetes was studied because the effects of abso- lute insulin deficiency and its replenishment was easier to determine. Only male subjects were included, so as to rule out the confounding effects of estrogens. The rou- tine therapeutic protocol of the Type-1 diabetic subjects was not interrupted by any occasion of the present study. In fact, the two groups (control and treated diabetic) were found comparable except in terms of glycosylated hemoglobin and lipid profiles (Table 1). We observed Copyright © 2011 SciRes. IJCM
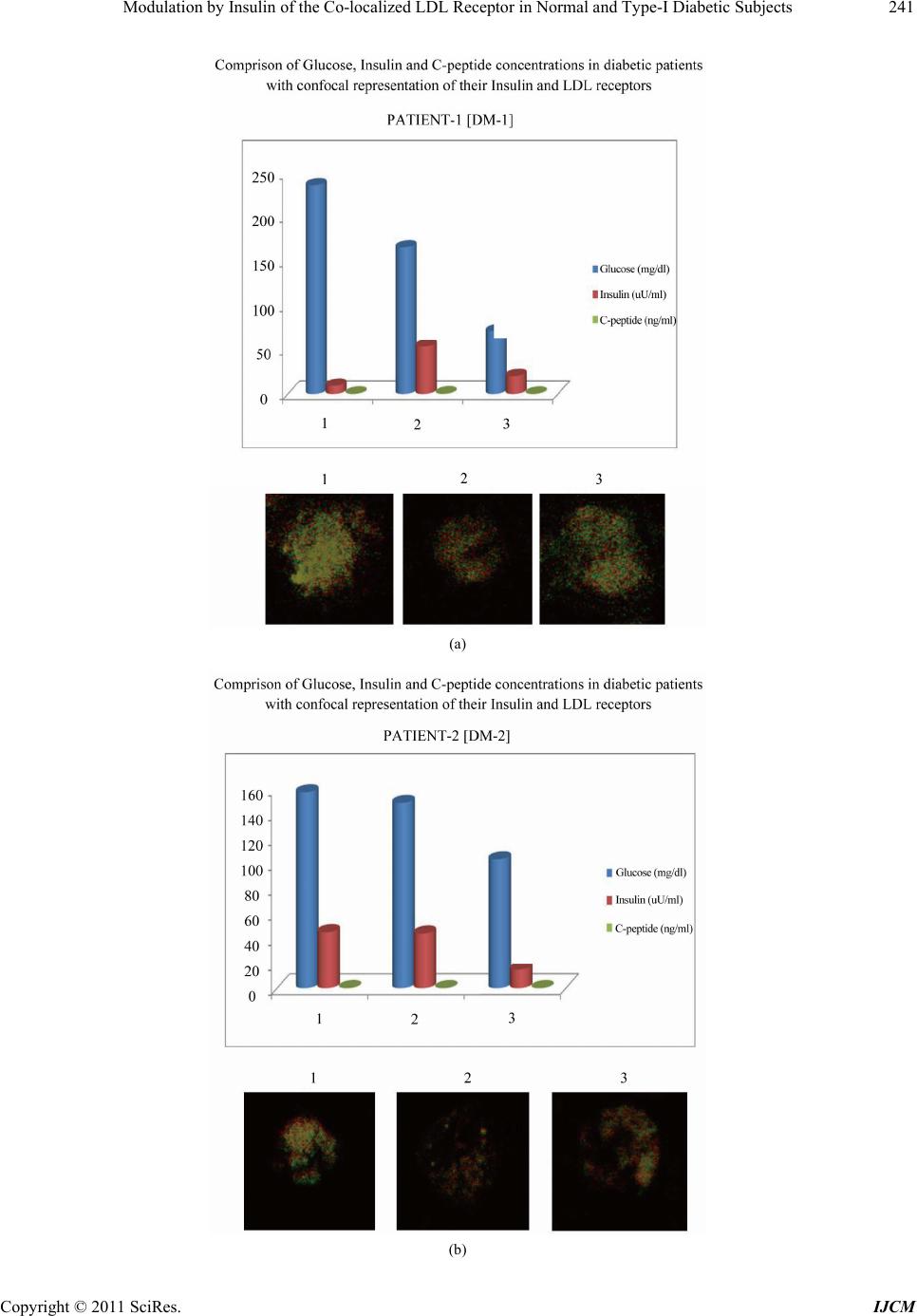 Modulation by Insulin of the Co-localized LDL Receptor in Normal and Type-I Diabetic Subjects241 (a) (b) Copyright © 2011 SciRes. IJCM
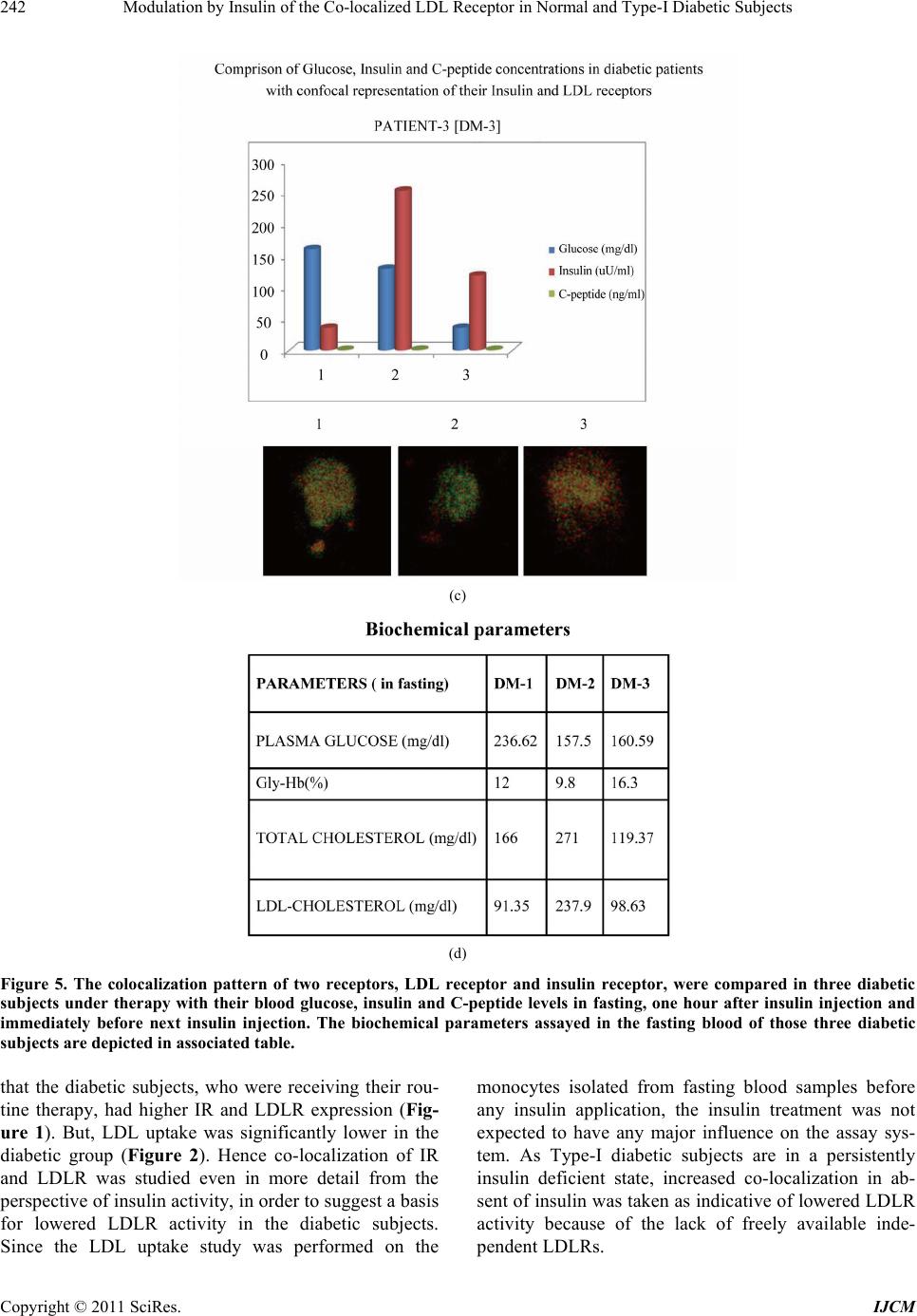 Modulation by Insulin of the Co-localized LDL Receptor in Normal and Type-I Diabetic Subjects 242 (c) (d) Figure 5. The colocalization pattern of two receptors, LDL receptor and insulin receptor, were compared in three diabetic subjects under therapy with their blood glucose, insulin and C-peptide levels in fasting, one hour after insulin injection and immediately before next insulin injection. The biochemical parameters assayed in the fasting blood of those three diabetic subjects are depicted in associated table. that the diabetic subjects, who were receiving their rou- tine therapy, had higher IR and LDLR expression (Fig- ure 1). But, LDL uptake was significantly lower in the diabetic group (Figure 2). Hence co-localization of IR and LDLR was studied even in more detail from the perspective of insulin activity, in order to suggest a basis for lowered LDLR activity in the diabetic subjects. Since the LDL uptake study was performed on the monocytes isolated from fasting blood samples before any insulin application, the insulin treatment was not expected to have any major influence on the assay sys- tem. As Type-I diabetic subjects are in a persistently insulin deficient state, increased co-localization in ab- sent of insulin was taken as indicative of lowered LDLR activity because of the lack of freely available inde- pendent LDLRs. Copyright © 2011 SciRes. IJCM
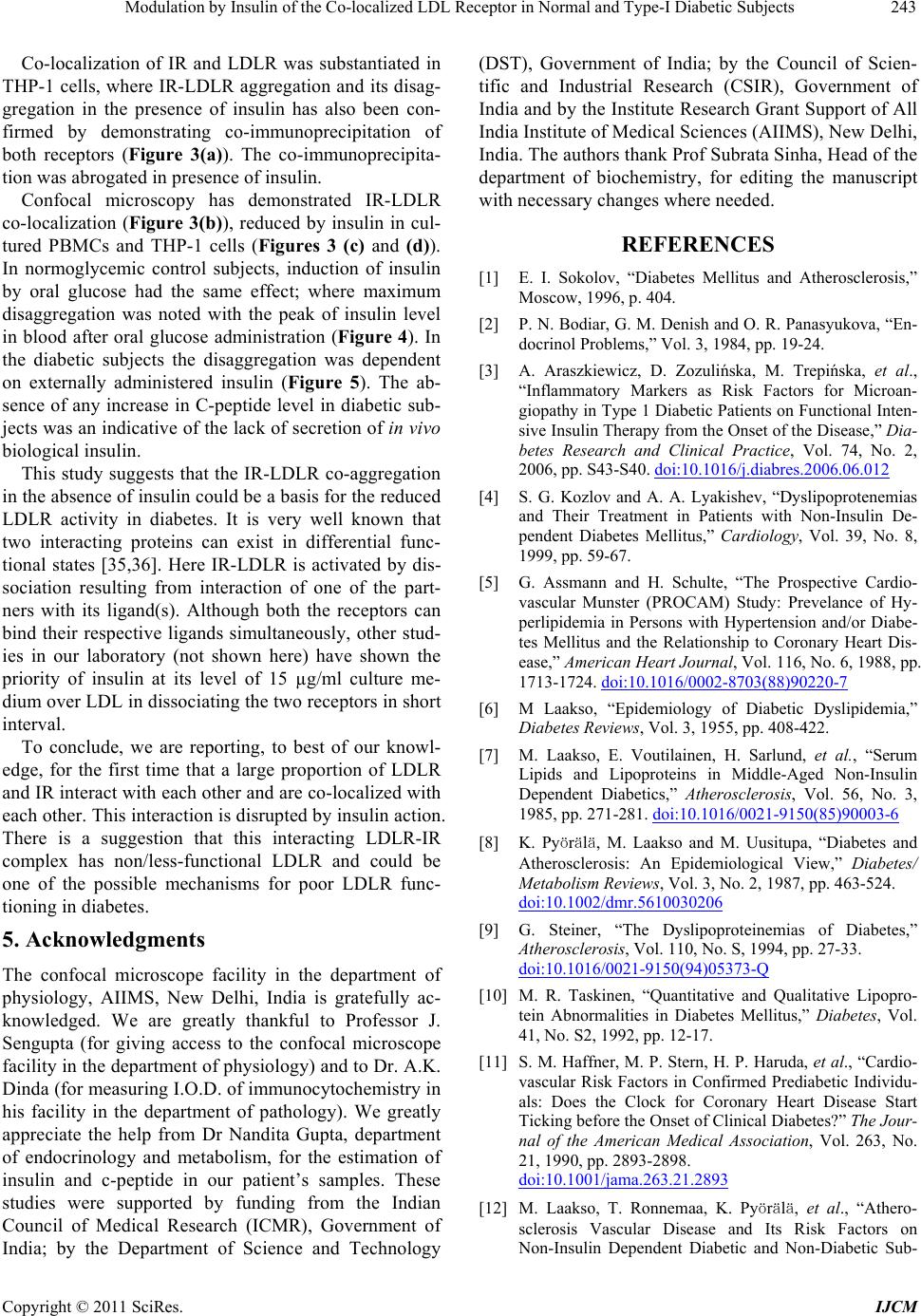 Modulation by Insulin of the Co-localized LDL Receptor in Normal and Type-I Diabetic Subjects243 Co-localization of IR and LDLR was substantiated in THP-1 cells, where IR-LDLR aggregation and its disag- gregation in the presence of insulin has also been con- firmed by demonstrating co-immunoprecipitation of both receptors (Figure 3(a)). The co-immunoprecipita- tion was abrogated in presence of insulin. Confocal microscopy has demonstrated IR-LDLR co-localization (Figure 3(b)), reduced by insulin in cul- tured PBMCs and THP-1 cells (Figures 3 (c) and (d)). In normoglycemic control subjects, induction of insulin by oral glucose had the same effect; where maximum disaggregation was noted with the peak of insulin level in blood after oral glucose administration (Figure 4). In the diabetic subjects the disaggregation was dependent on externally administered insulin (Figure 5). The ab- sence of any increase in C-peptide level in diabetic sub- jects was an indicative of the lack of secretion of in vivo biological insulin. This study suggests that the IR-LDLR co-aggregation in the absence of insulin could be a basis for the reduced LDLR activity in diabetes. It is very well known that two interacting proteins can exist in differential func- tional states [35,36]. Here IR-LDLR is activated by dis- sociation resulting from interaction of one of the part- ners with its ligand(s). Although both the receptors can bind their respective ligands simultaneously, other stud- ies in our laboratory (not shown here) have shown the priority of insulin at its level of 15 µg/ml culture me- dium over LDL in dissociating the two receptors in short interval. To conclude, we are reporting, to best of our knowl- edge, for the first time that a large proportion of LDLR and IR interact with each other and are co-localized with each other. This interaction is disrupted by insulin action. There is a suggestion that this interacting LDLR-IR complex has non/less-functional LDLR and could be one of the possible mechanisms for poor LDLR func- tioning in diabetes. 5. Acknowledgments The confocal microscope facility in the department of physiology, AIIMS, New Delhi, India is gratefully ac- knowledged. We are greatly thankful to Professor J. Sengupta (for giving access to the confocal microscope facility in the department of physiology) and to Dr. A.K. Dinda (for measuring I.O.D. of immunocytochemistry in his facility in the department of pathology). We greatly appreciate the help from Dr Nandita Gupta, department of endocrinology and metabolism, for the estimation of insulin and c-peptide in our patient’s samples. These studies were supported by funding from the Indian Council of Medical Research (ICMR), Government of India; by the Department of Science and Technology (DST), Government of India; by the Council of Scien- tific and Industrial Research (CSIR), Government of India and by the Institute Research Grant Support of All India Institute of Medical Sciences (AIIMS), New Delhi, India. The authors thank Prof Subrata Sinha, Head of the department of biochemistry, for editing the manuscript with necessary changes where needed. REFERENCES [1] E. I. Sokolov, “Diabetes Mellitus and Atherosclerosis,” Moscow, 1996, p. 404. [2] P. N. Bodiar, G. M. Denish and O. R. Panasyukova, “En- docrinol Problems,” Vol. 3, 1984, pp. 19-24. [3] A. Araszkiewicz, D. Zozulińska, M. Trepińska, et al., “Inflammatory Markers as Risk Factors for Microan- giopathy in Type 1 Diabetic Patients on Functional Inten- sive Insulin Therapy from the Onset of the Disease,” Dia- betes Research and Clinical Practice, Vol. 74, No. 2, 2006, pp. S43-S40. doi:10.1016/j.diabres.2006.06.012 [4] S. G. Kozlov and A. A. Lyakishev, “Dyslipoprotenemias and Their Treatment in Patients with Non-Insulin De- pendent Diabetes Mellitus,” Cardiology, Vol. 39, No. 8, 1999, pp. 59-67. [5] G. Assmann and H. Schulte, “The Prospective Cardio- vascular Munster (PROCAM) Study: Prevelance of Hy- perlipidemia in Persons with Hypertension and/or Diabe- tes Mellitus and the Relationship to Coronary Heart Dis- ease,” American Heart Journal, Vol. 116, No. 6, 1988, pp. 1713-1724. doi:10.1016/0002-8703(88)90220-7 [6] M Laakso, “Epidemiology of Diabetic Dyslipidemia,” Diabetes Reviews, Vol. 3, 1955, pp. 408-422. [7] M. Laakso, E. Voutilainen, H. Sarlund, et al., “Serum Lipids and Lipoproteins in Middle-Aged Non-Insulin Dependent Diabetics,” Atherosclerosis, Vol. 56, No. 3, 1985, pp. 271-281. doi:10.1016/0021-9150(85)90003-6 [8] K. Pyӧrӓlӓ, M. Laakso and M. Uusitupa, “Diabetes and Atherosclerosis: An Epidemiological View,” Diabetes/ Metabolism Reviews, Vol. 3, No. 2, 1987, pp. 463-524. doi:10.1002/dmr.5610030206 [9] G. Steiner, “The Dyslipoproteinemias of Diabetes,” Atherosclerosis, Vol. 110, No. S, 1994, pp. 27-33. doi:10.1016/0021-9150(94)05373-Q [10] M. R. Taskinen, “Quantitative and Qualitative Lipopro- tein Abnormalities in Diabetes Mellitus,” Diabetes, Vol. 41, No. S2, 1992, pp. 12-17. [11] S. M. Haffner, M. P. Stern, H. P. Haruda, et al., “Cardio- vascular Risk Factors in Confirmed Prediabetic Individu- als: Does the Clock for Coronary Heart Disease Start Ticking before the Onset of Clinical Diabetes?” The Jour- nal of the American Medical Association, Vol. 263, No. 21, 1990, pp. 2893-2898. doi:10.1001/jama.263.21.2893 [12] M. Laakso, T. Ronnemaa, K. Pyӧrӓlӓ, et al., “Athero- sclerosis Vascular Disease and Its Risk Factors on Non-Insulin Dependent Diabetic and Non-Diabetic Sub- Copyright © 2011 SciRes. IJCM
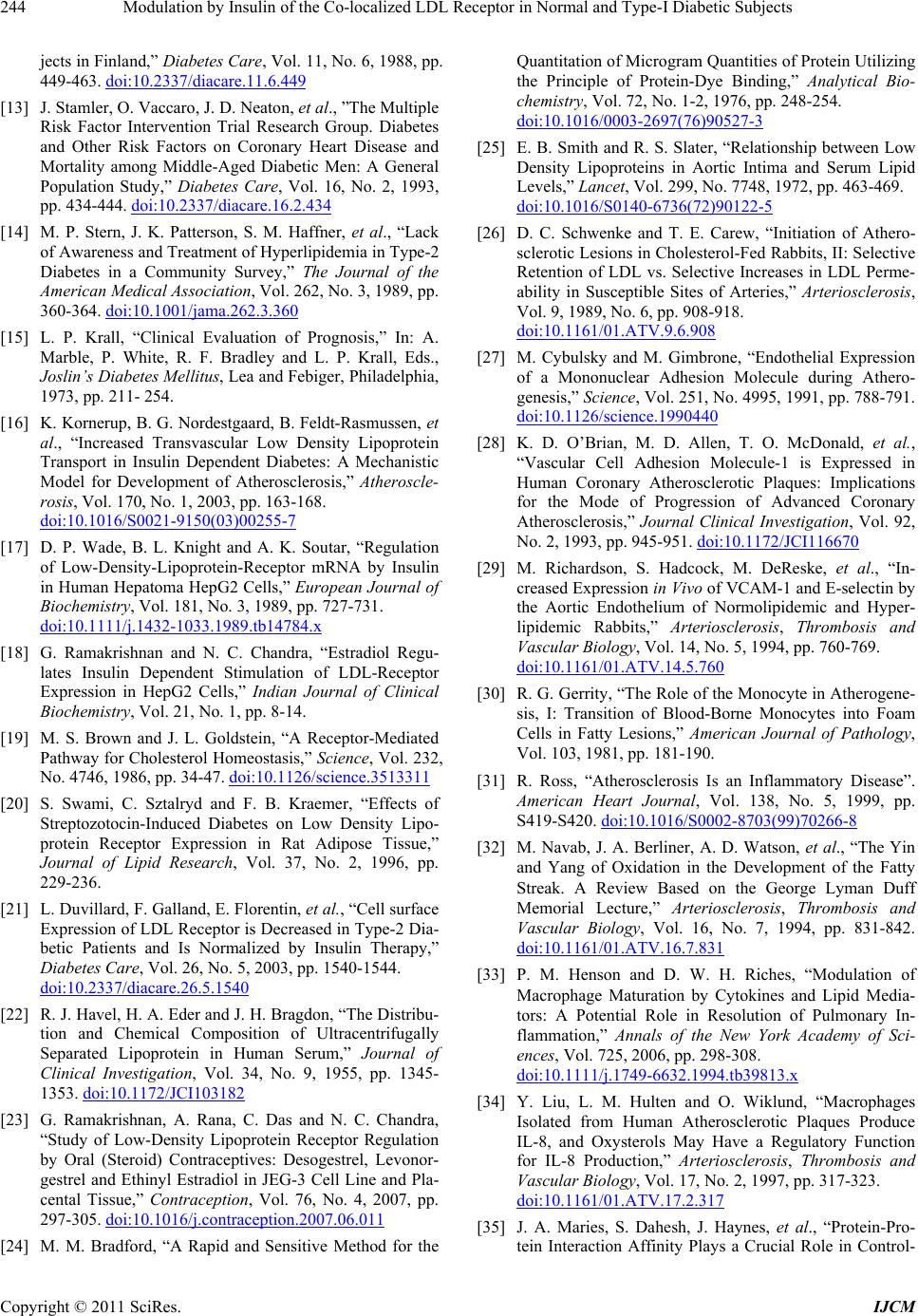 Modulation by Insulin of the Co-localized LDL Receptor in Normal and Type-I Diabetic Subjects 244 jects in Finland,” Diabetes Care, Vol. 11, No. 6, 1988, pp. 449-463. doi:10.2337/diacare.11.6.449 [13] J. Stamler, O. Vaccaro, J. D. Neaton, et al., ”The Multiple Risk Factor Intervention Trial Research Group. Diabetes and Other Risk Factors on Coronary Heart Disease and Mortality among Middle-Aged Diabetic Men: A General Population Study,” Diabetes Care, Vol. 16, No. 2, 1993, pp. 434-444. doi:10.2337/diacare.16.2.434 [14] M. P. Stern, J. K. Patterson, S. M. Haffner, et al., “Lack of Awareness and Treatment of Hyperlipidemia in Type-2 Diabetes in a Community Survey,” The Journal of the American Medical Association, Vol. 262, No. 3, 1989, pp. 360-364. doi:10.1001/jama.262.3.360 [15] L. P. Krall, “Clinical Evaluation of Prognosis,” In: A. Marble, P. White, R. F. Bradley and L. P. Krall, Eds., Joslin’s Diabetes Mellitus, Lea and Febiger, Philadelphia, 1973, pp. 211- 254. [16] K. Kornerup, B. G. Nordestgaard, B. Feldt-Rasmussen, et al., “Increased Transvascular Low Density Lipoprotein Transport in Insulin Dependent Diabetes: A Mechanistic Model for Development of Atherosclerosis,” Atheroscle- rosis, Vol. 170, No. 1, 2003, pp. 163-168. doi:10.1016/S0021-9150(03)00255-7 [17] D. P. Wade, B. L. Knight and A. K. Soutar, “Regulation of Low-Density-Lipoprotein-Receptor mRNA by Insulin in Human Hepatoma HepG2 Cells,” European Journal of Biochemistry, Vol. 181, No. 3, 1989, pp. 727-731. doi:10.1111/j.1432-1033.1989.tb14784.x [18] G. Ramakrishnan and N. C. Chandra, “Estradiol Regu- lates Insulin Dependent Stimulation of LDL-Receptor Expression in HepG2 Cells,” Indian Journal of Clinical Biochemistry, Vol. 21, No. 1, pp. 8-14. [19] M. S. Brown and J. L. Goldstein, “A Receptor-Mediated Pathway for Cholesterol Homeostasis,” Science, Vol. 232, No. 4746, 1986, pp. 34-47. doi:10.1126/science.3513311 [20] S. Swami, C. Sztalryd and F. B. Kraemer, “Effects of Streptozotocin-Induced Diabetes on Low Density Lipo- protein Receptor Expression in Rat Adipose Tissue,” Journal of Lipid Research, Vol. 37, No. 2, 1996, pp. 229-236. [21] L. Duvillard, F. Galland, E. Florentin, et al., “Cell surface Expression of LDL Receptor is Decreased in Type-2 Dia- betic Patients and Is Normalized by Insulin Therapy,” Diabetes Care, Vol. 26, No. 5, 2003, pp. 1540-1544. doi:10.2337/diacare.26.5.1540 [22] R. J. Havel, H. A. Eder and J. H. Bragdon, “The Distribu- tion and Chemical Composition of Ultracentrifugally Separated Lipoprotein in Human Serum,” Journal of Clinical Investigation, Vol. 34, No. 9, 1955, pp. 1345- 1353. doi:10.1172/JCI103182 [23] G. Ramakrishnan, A. Rana, C. Das and N. C. Chandra, “Study of Low-Density Lipoprotein Receptor Regulation by Oral (Steroid) Contraceptives: Desogestrel, Levonor- gestrel and Ethinyl Estradiol in JEG-3 Cell Line and Pla- cental Tissue,” Contraception, Vol. 76, No. 4, 2007, pp. 297-305. doi:10.1016/j.contraception.2007.06.011 [24] M. M. Bradford, “A Rapid and Sensitive Method for the Quantitation of Microgram Quantities of Protein Utilizing the Principle of Protein-Dye Binding,” Analytical Bio- chemistry, Vol. 72, No. 1-2, 1976, pp. 248-254. doi:10.1016/0003-2697(76)90527-3 [25] E. B. Smith and R. S. Slater, “Relationship between Low Density Lipoproteins in Aortic Intima and Serum Lipid Levels,” Lancet, Vol. 299, No. 7748, 1972, pp. 463-469. doi:10.1016/S0140-6736(72)90122-5 [26] D. C. Schwenke and T. E. Carew, “Initiation of Athero- sclerotic Lesions in Cholesterol-Fed Rabbits, II: Selective Retention of LDL vs. Selective Increases in LDL Perme- ability in Susceptible Sites of Arteries,” Arteriosclerosis, Vol. 9, 1989, No. 6, pp. 908-918. doi:10.1161/01.ATV.9.6.908 [27] M. Cybulsky and M. Gimbrone, “Endothelial Expression of a Mononuclear Adhesion Molecule during Athero- genesis,” Science, Vol. 251, No. 4995, 1991, pp. 788-791. doi:10.1126/science.1990440 [28] K. D. O’Brian, M. D. Allen, T. O. McDonald, et al., “Vascular Cell Adhesion Molecule-1 is Expressed in Human Coronary Atherosclerotic Plaques: Implications for the Mode of Progression of Advanced Coronary Atherosclerosis,” Journal Clinical Investigation, Vol. 92, No. 2, 1993, pp. 945-951. doi:10.1172/JCI116670 [29] M. Richardson, S. Hadcock, M. DeReske, et al., “In- creased Expression in Vivo of VCAM-1 and E-selectin by the Aortic Endothelium of Normolipidemic and Hyper- lipidemic Rabbits,” Arteriosclerosis, Thrombosis and Vascular Biology, Vol. 14, No. 5, 1994, pp. 760-769. doi:10.1161/01.ATV.14.5.760 [30] R. G. Gerrity, “The Role of the Monocyte in Atherogene- sis, I: Transition of Blood-Borne Monocytes into Foam Cells in Fatty Lesions,” American Journal of Pathology, Vol. 103, 1981, pp. 181-190. [31] R. Ross, “Atherosclerosis Is an Inflammatory Disease”. American Heart Journal, Vol. 138, No. 5, 1999, pp. S419-S420. doi:10.1016/S0002-8703(99)70266-8 [32] M. Navab, J. A. Berliner, A. D. Watson, et al., “The Yin and Yang of Oxidation in the Development of the Fatty Streak. A Review Based on the George Lyman Duff Memorial Lecture,” Arteriosclerosis, Thrombosis and Vascular Biology, Vol. 16, No. 7, 1994, pp. 831-842. doi:10.1161/01.ATV.16.7.831 [33] P. M. Henson and D. W. H. Riches, “Modulation of Macrophage Maturation by Cytokines and Lipid Media- tors: A Potential Role in Resolution of Pulmonary In- flammation,” Annals of the New York Academy of Sci- ences, Vol. 725, 2006, pp. 298-308. doi:10.1111/j.1749-6632.1994.tb39813.x [34] Y. Liu, L. M. Hulten and O. Wiklund, “Macrophages Isolated from Human Atherosclerotic Plaques Produce IL-8, and Oxysterols May Have a Regulatory Function for IL-8 Production,” Arteriosclerosis, Thrombosis and Vascular Biology, Vol. 17, No. 2, 1997, pp. 317-323. doi:10.1161/01.ATV.17.2.317 [35] J. A. Maries, S. Dahesh, J. Haynes, et al., “Protein-Pro- tein Interaction Affinity Plays a Crucial Role in Control- Copyright © 2011 SciRes. IJCM
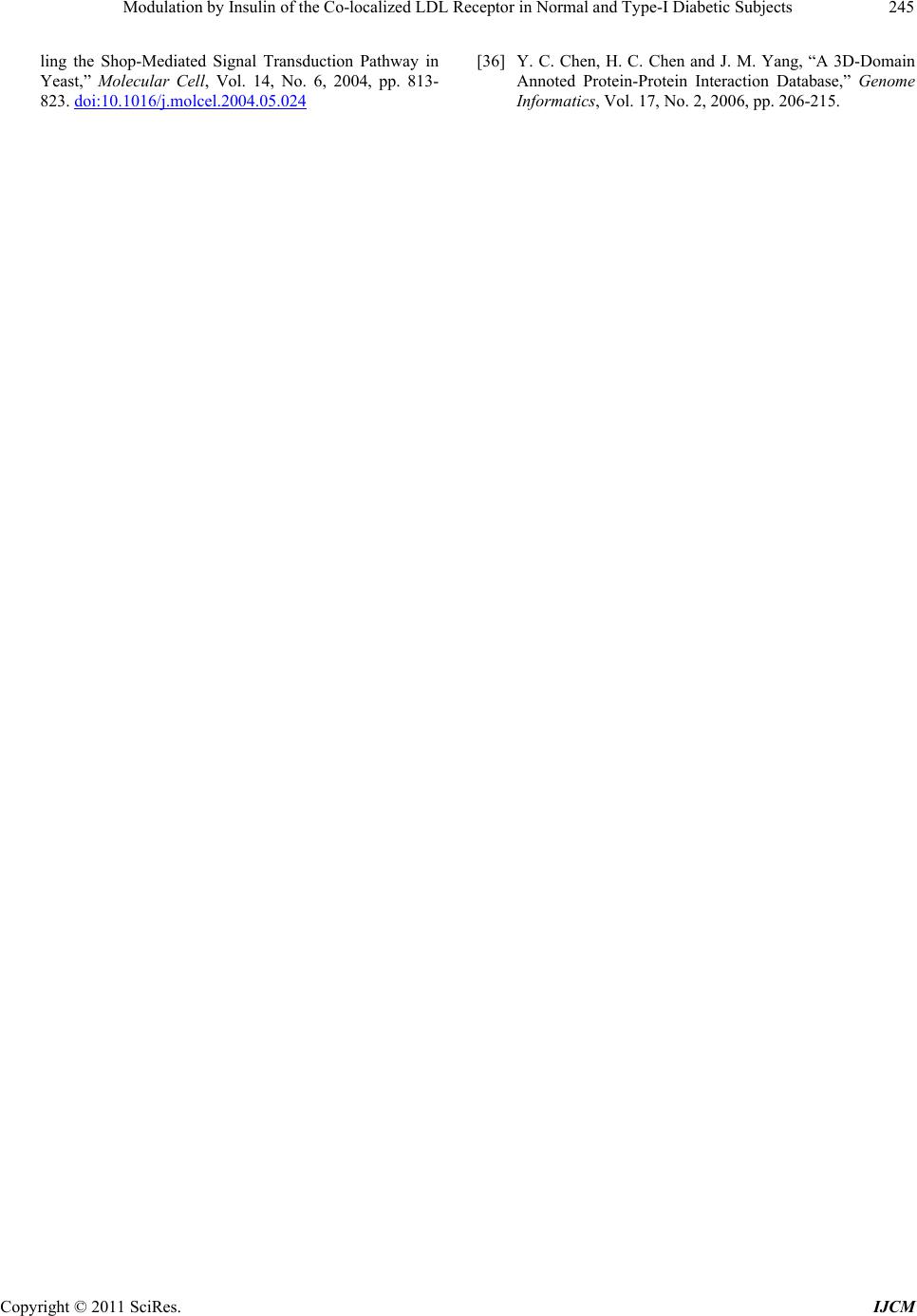 Modulation by Insulin of the Co-localized LDL Receptor in Normal and Type-I Diabetic Subjects Copyright © 2011 SciRes. IJCM 245 ling the Shop-Mediated Signal Transduction Pathway in Yeast,” Molecular Cell, Vol. 14, No. 6, 2004, pp. 813- 823. doi:10.1016/j.molcel.2004.05.024 [36] Y. C. Chen, H. C. Chen and J. M. Yang, “A 3D-Domain Annoted Protein-Protein Interaction Database,” Genome Informatics, Vol. 17, No. 2, 2006, pp. 206-215.
|