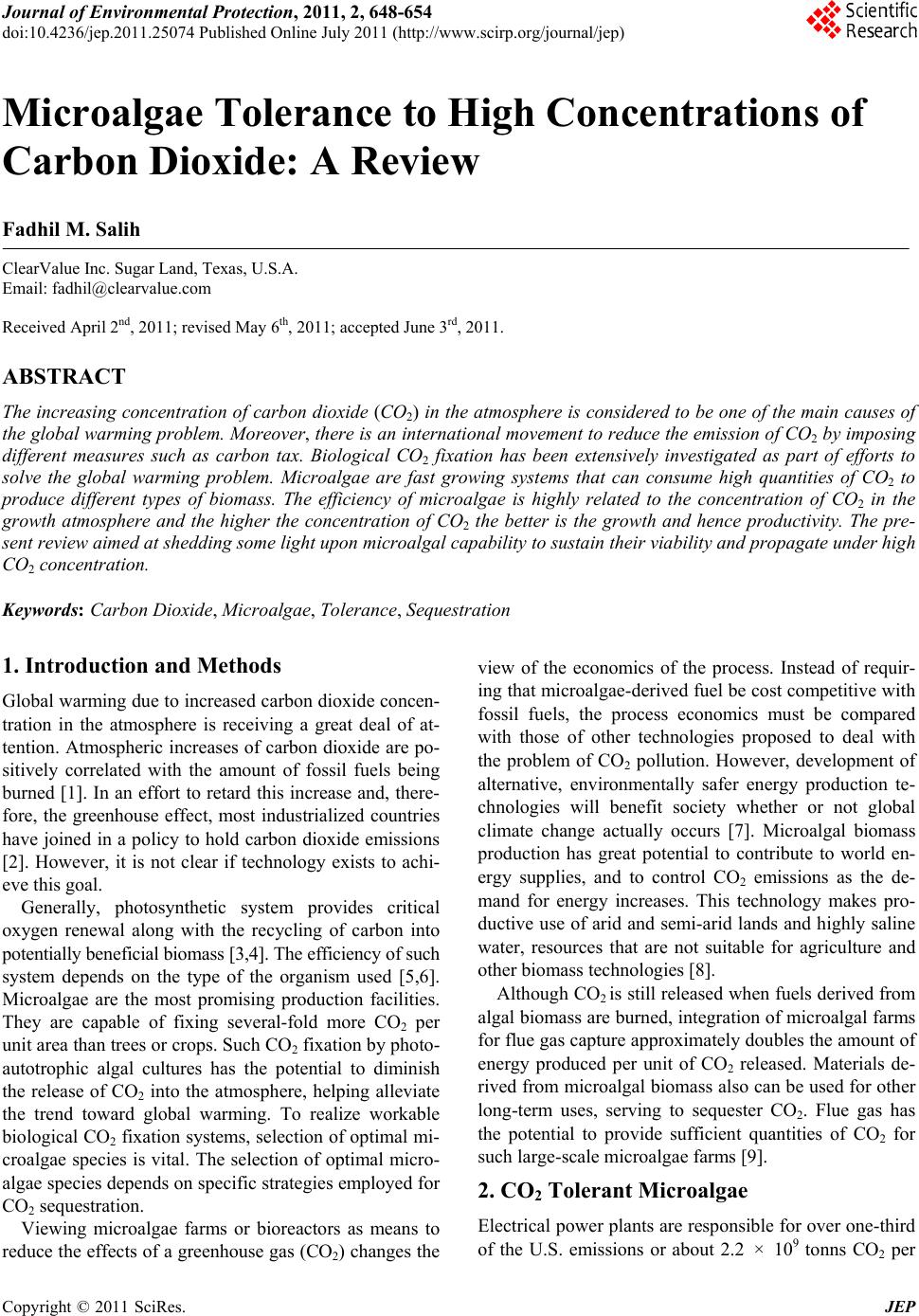 Journal of Environmental Protection, 2011, 2, 648-654 doi:10.4236/jep.2011.25074 Published Online July 2011 (http://www.scirp.org/journal/jep) Copyright © 2011 SciRes. JEP Microalgae Tolerance to High Concentrations of Carbon Dioxide: A Review Fadhil M. Salih ClearValue Inc. Sugar Land, Texas, U.S.A. Email: fadhil@clearvalue.com Received April 2nd, 2011; revised May 6th, 2011; accepted June 3rd, 2011. ABSTRACT The increasing concentration of carbon dioxide (CO2) in the atmosphere is considered to be one of the main causes of the global warming problem. Moreover, there is an international movement to redu ce the emission of CO2 by imposing different measures such as carbon tax. Biological CO2 fixation has been extensively investigated as part of efforts to solve the global warming problem. Microalgae are fast growing systems that can consume high quantities of CO2 to produce different types of biomass. The efficiency of microalgae is highly related to the concentration of CO2 in the growth atmosphere and the higher the concentration of CO2 the better is the growth and hence productivity. The pre- sent review aimed at shedding some light upon microalgal capab ility to sustain their viab ility and propaga te unde r high CO2 concentration. Keywords: Carbon Dioxide, Microalgae, Tolerance, Sequestration 1. Introduction and Methods Global warming due to increased carbon dioxide concen- tration in the atmosphere is receiving a great deal of at- tention. Atmospheric increases of carbon dioxide are po- sitively correlated with the amount of fossil fuels being burned [1]. In an effort to retard this increase and, there- fore, the greenhouse effect, most industrialized countries have joined in a policy to hold carbon dioxide emissions [2]. However, it is not clear if technology exists to achi- eve this goal. Generally, photosynthetic system provides critical oxygen renewal along with the recycling of carbon into potentially beneficial biomass [3,4]. The efficiency of such system depends on the type of the organism used [5,6]. Microalgae are the most promising production facilities. They are capable of fixing several-fold more CO2 per unit area than trees or crops. Such CO2 fixation by photo- autotrophic algal cultures has the potential to diminish the release of CO2 into the atmosphere, helping alleviate the trend toward global warming. To realize workable biological CO2 fixation systems, selection of optimal mi- croalgae species is vital. The selection of optimal micro- algae species depends on specific strategies employed for CO2 sequestration. Viewing microalgae farms or bioreactors as means to reduce the effects of a greenhouse gas (CO2) changes the view of the economics of the process. Instead of requir- ing that microalgae-derived fuel be cost competitive with fossil fuels, the process economics must be compared with those of other technologies proposed to deal with the problem of CO2 pollution. However, development of alternative, environmentally safer energy production te- chnologies will benefit society whether or not global climate change actually occurs [7]. Microalgal biomass production has great potential to contribute to world en- ergy supplies, and to control CO2 emissions as the de- mand for energy increases. This technology makes pro- ductive use of arid and semi-arid lands and highly saline water, resources that are not suitable for agriculture and other biomass technologies [8]. Although CO2 is still released when fuels derived from algal biomass are burned, integration of microalgal farms for flue gas capture approximately doubles the amount of energy produced per unit of CO2 released. Materials de- rived from microalgal biomass also can be used for other long-term uses, serving to sequester CO2. Flue gas has the potential to provide sufficient quantities of CO2 for such large-scale microalgae farms [9]. 2. CO2 Tolerant Microalgae Electrical power plants are responsible for over one-third of the U.S. emissions or about 2.2 × 109 tonns CO2 per
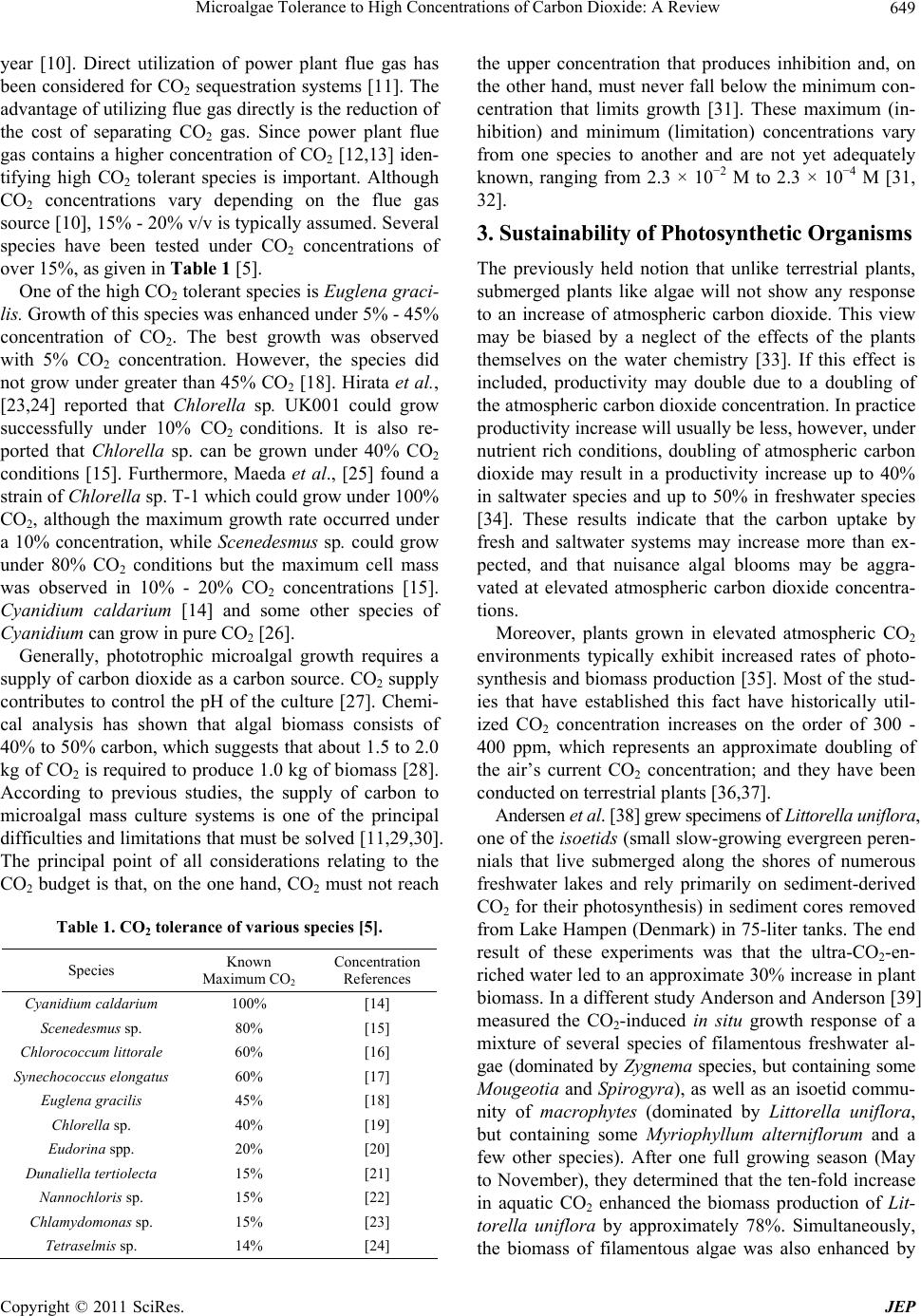 Microalgae Tolerance to High Concentrations of Carbon Dioxide: A Review649 year [10]. Direct utilization of power plant flue gas has been considered for CO2 sequestration systems [11]. The advantage of utilizing flue gas directly is the reduction of the cost of separating CO2 gas. Since power plant flue gas contains a higher concentration of CO2 [12,13] iden- tifying high CO2 tolerant species is important. Although CO2 concentrations vary depending on the flue gas source [10], 15% - 20% v/v is typically assumed. Several species have been tested under CO2 concentrations of over 15%, as given in Table 1 [5]. One of the high CO2 tolerant species is Euglena graci- lis. Growth of this species was enhanced under 5% - 45% concentration of CO2. The best growth was observed with 5% CO2 concentration. However, the species did not grow under greater than 45% CO2 [18]. Hirata et al., [23,24] reported that Chlorella sp. UK001 could grow successfully under 10% CO2 conditions. It is also re- ported that Chlorella sp. can be grown under 40% CO2 conditions [15]. Furthermore, Maeda et al., [25] found a strain of Chlorella sp. T-1 which could grow under 100% CO2, although the maximum growth rate occurred under a 10% concentration, while Scenedesmus sp. could grow under 80% CO2 conditions but the maximum cell mass was observed in 10% - 20% CO2 concentrations [15]. Cyanidium caldarium [14] and some other species of Cyanidium can grow in pure CO2 [26]. Generally, phototrophic microalgal growth requires a supply of carbon dioxide as a carbon source. CO2 supply contributes to control the pH of the culture [27]. Chemi- cal analysis has shown that algal biomass consists of 40% to 50% carbon, which suggests that about 1.5 to 2.0 kg of CO2 is required to produce 1.0 kg of biomass [28]. According to previous studies, the supply of carbon to microalgal mass culture systems is one of the principal difficulties and limitations that must be solved [11,29,30]. The principal point of all considerations relating to the CO2 budget is that, on the one hand, CO2 must not reach Table 1. CO2 tolerance of various species [5]. Species Known Maximum CO2 Concentration References Cyanidium caldarium 100% [14] Scenedesmus sp. 80% [15] Chlorococcum littorale 60% [16] Synechococcus elongatus 60% [17] Euglena gracilis 45% [18] Chlorella sp. 40% [19] Eudorina spp. 20% [20] Dunaliella tertiolecta 15% [21] Nannochloris sp. 15% [22] Chlamydomonas sp. 15% [23] Tetraselmis sp. 14% [24] the upper concentration that produces inhibition and, on the other hand, must never fall below the minimum con- centration that limits growth [31]. These maximum (in- hibition) and minimum (limitation) concentrations vary from one species to another and are not yet adequately known, ranging from 2.3 × 10−2 M to 2.3 × 10−4 M [31, 32]. 3. Sustainability of Photosynthetic Organisms The previously held notion that unlike terrestrial plants, submerged plants like algae will not show any response to an increase of atmospheric carbon dioxide. This view may be biased by a neglect of the effects of the plants themselves on the water chemistry [33]. If this effect is included, productivity may double due to a doubling of the atmospheric carbon dioxide concentration. In practice productivity increase will usually be less, however, under nutrient rich conditions, doubling of atmospheric carbon dioxide may result in a productivity increase up to 40% in saltwater species and up to 50% in freshwater species [34]. These results indicate that the carbon uptake by fresh and saltwater systems may increase more than ex- pected, and that nuisance algal blooms may be aggra- vated at elevated atmospheric carbon dioxide concentra- tions. Moreover, plants grown in elevated atmospheric CO2 environments typically exhibit increased rates of photo- synthesis and biomass production [35]. Most of the stud- ies that have established this fact have historically util- ized CO2 concentration increases on the order of 300 - 400 ppm, which represents an approximate doubling of the air’s current CO2 concentration; and they have been conducted on terrestrial plants [36,37]. Andersen et al. [38] grew specimens of Littorel la uniflora, one of the isoetids (small slow-growing evergreen peren- nials that live submerged along the shores of numerous freshwater lakes and rely primarily on sediment-derived CO2 for their photosynthesis) in sediment cores removed from Lake Hampen (Denmark) in 75-liter tanks. The end result of these experiments was that the ultra-CO2-en- riched water led to an approximate 30% increase in plant biomass. In a different study Anderson and Anderson [39] measured the CO2-induced in situ growth response of a mixture of several species of filamentous freshwater al- gae (dominated by Zygnema species, but containing some Mougeotia and Spirogyra), as well as an isoetid commu- nity of macrophytes (dominated by Littorella uniflora, but containing some Myriophyllum alterniflorum and a few other species). After one full growing season (May to November), they determined that the ten-fold increase in aquatic CO2 enhanced the biomass production of Lit- torella uniflora by approximately 78%. Simultaneously, the biomass of filamentous algae was also enhanced by Copyright © 2011 SciRes. JEP
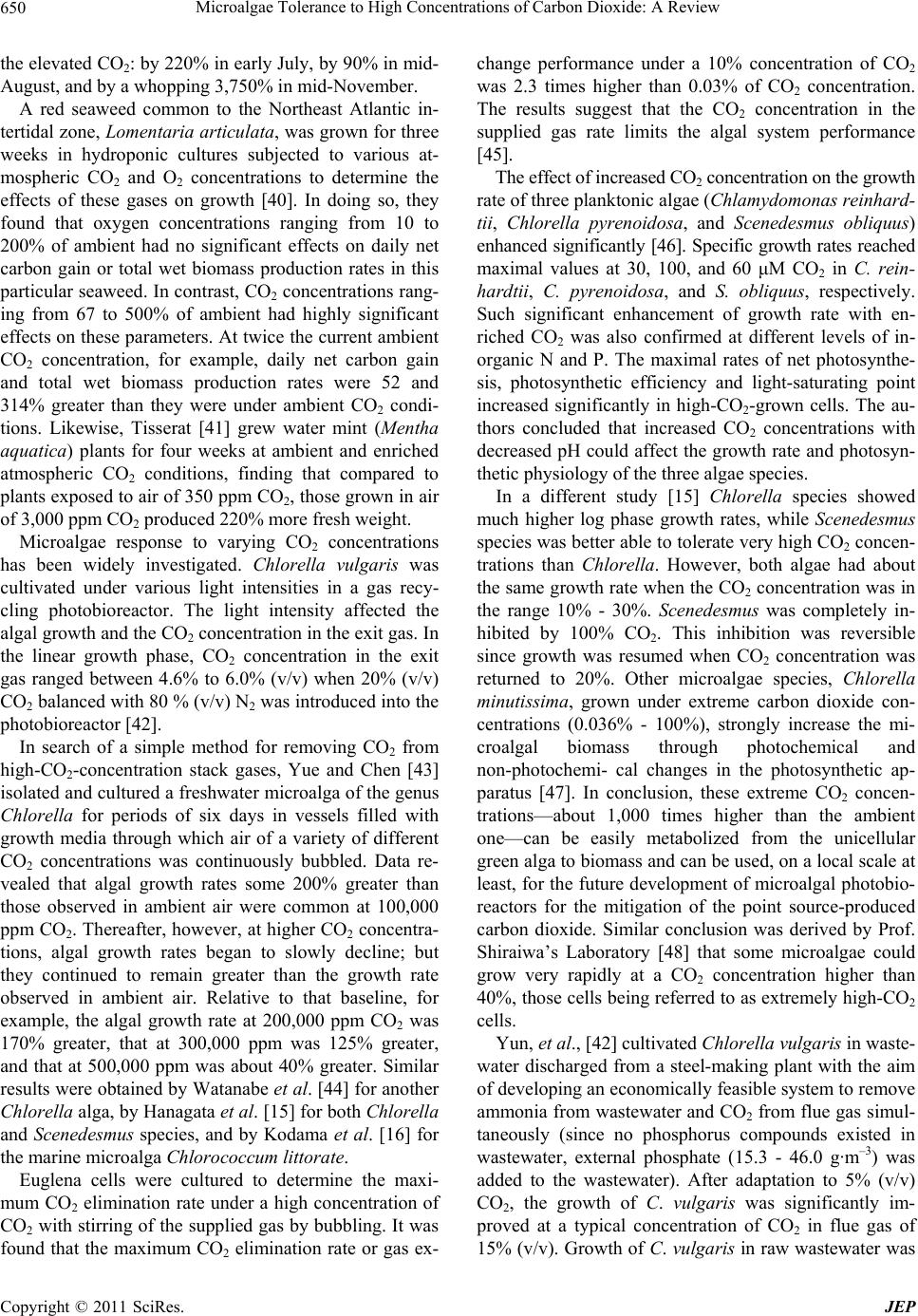 Microalgae Tolerance to High Concentrations of Carbon Dioxide: A Review 650 the elevated CO2: by 220% in early July, by 90% in mid- August, and by a whopping 3,750% in mid-November. A red seaweed common to the Northeast Atlantic in- tertidal zone, Lomentaria a rticulata, was grown for three weeks in hydroponic cultures subjected to various at- mospheric CO2 and O2 concentrations to determine the effects of these gases on growth [40]. In doing so, they found that oxygen concentrations ranging from 10 to 200% of ambient had no significant effects on daily net carbon gain or total wet biomass production rates in this particular seaweed. In contrast, CO2 concentrations rang- ing from 67 to 500% of ambient had highly significant effects on these parameters. At twice the current ambient CO2 concentration, for example, daily net carbon gain and total wet biomass production rates were 52 and 314% greater than they were under ambient CO2 condi- tions. Likewise, Tisserat [41] grew water mint (Mentha aquatica) plants for four weeks at ambient and enriched atmospheric CO2 conditions, finding that compared to plants exposed to air of 350 ppm CO2, those grown in air of 3,000 ppm CO2 produced 220% more fresh weight. Microalgae response to varying CO2 concentrations has been widely investigated. Chlorella vulgaris was cultivated under various light intensities in a gas recy- cling photobioreactor. The light intensity affected the algal growth and the CO2 concentration in the exit gas. In the linear growth phase, CO2 concentration in the exit gas ranged between 4.6% to 6.0% (v/v) when 20% (v/v) CO2 balanced with 80 % (v/v) N2 was introduced into the photobioreactor [42]. In search of a simple method for removing CO2 from high-CO2-concentration stack gases, Yue and Chen [43] isolated and cultured a freshwater microalga of the genus Chlorella for periods of six days in vessels filled with growth media through which air of a variety of different CO2 concentrations was continuously bubbled. Data re- vealed that algal growth rates some 200% greater than those observed in ambient air were common at 100,000 ppm CO2. Thereafter, however, at higher CO2 concentra- tions, algal growth rates began to slowly decline; but they continued to remain greater than the growth rate observed in ambient air. Relative to that baseline, for example, the algal growth rate at 200,000 ppm CO2 was 170% greater, that at 300,000 ppm was 125% greater, and that at 500,000 ppm was about 40% greater. Similar results were obtained by Watanabe et al. [44] for another Chlorella alga, by Hanagata et al. [15] for both Chlorella and Scenedesmus species, and by Kodama et al. [16] for the marine microalga Chlorococcum littora te. Euglena cells were cultured to determine the maxi- mum CO2 elimination rate under a high concentration of CO2 with stirring of the supplied gas by bubbling. It was found that the maximum CO2 elimination rate or gas ex- change performance under a 10% concentration of CO2 was 2.3 times higher than 0.03% of CO2 concentration. The results suggest that the CO2 concentration in the supplied gas rate limits the algal system performance [45]. The effect of increased CO2 concentration on the growth rate of three planktonic algae (Chlamydomonas reinhard- tii, Chlorella pyrenoidosa, and Scenedesmus obliquus) enhanced significantly [46]. Specific growth rates reached maximal values at 30, 100, and 60 μM CO2 in C. rein- hardtii, C. pyrenoidosa, and S. obliquus, respectively. Such significant enhancement of growth rate with en- riched CO2 was also confirmed at different levels of in- organic N and P. The maximal rates of net photosynthe- sis, photosynthetic efficiency and light-saturating point increased significantly in high-CO2-grown cells. The au- thors concluded that increased CO2 concentrations with decreased pH could affect the growth rate and photosyn- thetic physiology of the three algae species. In a different study [15] Chlorella species showed much higher log phase growth rates, while Scenedesmus species was better able to tolerate very high CO2 concen- trations than Chlorella. However, both algae had about the same growth rate when the CO2 concentration was in the range 10% - 30%. Scenedesmus was completely in- hibited by 100% CO2. This inhibition was reversible since growth was resumed when CO2 concentration was returned to 20%. Other microalgae species, Chlorella minutissima, grown under extreme carbon dioxide con- centrations (0.036% - 100%), strongly increase the mi- croalgal biomass through photochemical and non-photochemi- cal changes in the photosynthetic ap- paratus [47]. In conclusion, these extreme CO2 concen- trations—about 1,000 times higher than the ambient one—can be easily metabolized from the unicellular green alga to biomass and can be used, on a local scale at least, for the future development of microalgal photobio- reactors for the mitigation of the point source-produced carbon dioxide. Similar conclusion was derived by Prof. Shiraiwa’s Laboratory [48] that some microalgae could grow very rapidly at a CO2 concentration higher than 40%, those cells being referred to as extremely high-CO2 cells. Yun, et al., [42] cultivated Chlorella vulgaris in waste- water discharged from a steel-making plant with the aim of developing an economically feasible system to remove ammonia from wastewater and CO2 from flue gas simul- taneously (since no phosphorus compounds existed in wastewater, external phosphate (15.3 - 46.0 g·m–3) was added to the wastewater). After adaptation to 5% (v/v) CO2, the growth of C. vulgaris was significantly im- proved at a typical concentration of CO2 in flue gas of 15% (v/v). Growth of C. vulgaris in raw wastewater was C opyright © 2011 SciRes. JEP
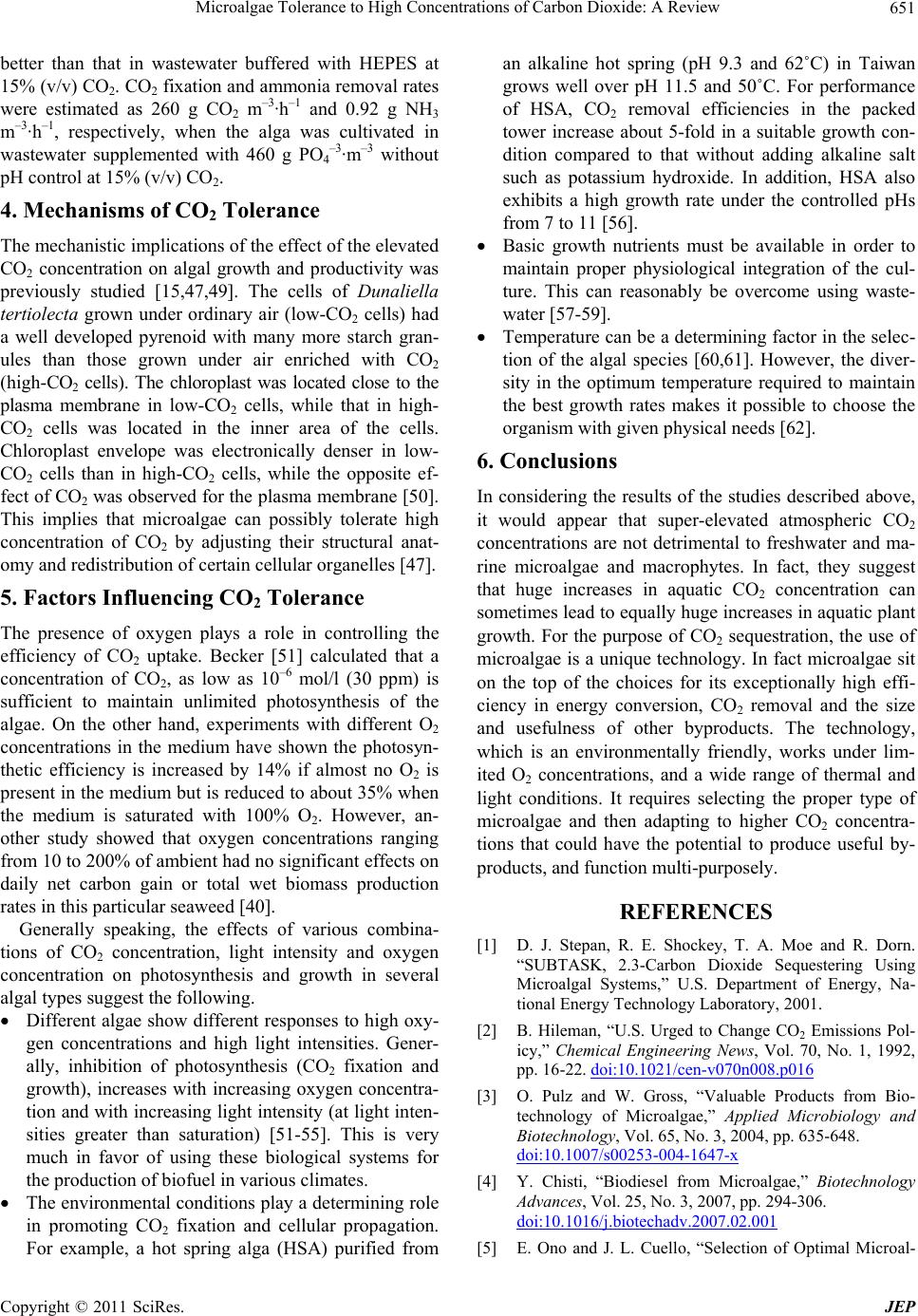 Microalgae Tolerance to High Concentrations of Carbon Dioxide: A Review651 better than that in wastewater buffered with HEPES at 15% (v/v) CO2. CO2 fixation and ammonia removal rates were estimated as 260 g CO2 m –3·h–1 and 0.92 g NH3 m–3·h–1, respectively, when the alga was cultivated in wastewater supplemented with 460 g PO4 –3·m–3 without pH control at 15% (v/v) CO2. 4. Mechanisms of CO2 Tolerance The mechanistic implications of the effect of the elevated CO2 concentration on algal growth and productivity was previously studied [15,47,49]. The cells of Dunaliella tertiolecta grown under ordinary air (low-CO2 cells) had a well developed pyrenoid with many more starch gran- ules than those grown under air enriched with CO2 (high-CO2 cells). The chloroplast was located close to the plasma membrane in low-CO2 cells, while that in high- CO2 cells was located in the inner area of the cells. Chloroplast envelope was electronically denser in low- CO2 cells than in high-CO2 cells, while the opposite ef- fect of CO2 was observed for the plasma membrane [50]. This implies that microalgae can possibly tolerate high concentration of CO2 by adjusting their structural anat- omy and redistribution of certain cellular organelles [47]. 5. Factors Influencing CO2 Tolerance The presence of oxygen plays a role in controlling the efficiency of CO2 uptake. Becker [51] calculated that a concentration of CO2, as low as 10–6 mol/l (30 ppm) is sufficient to maintain unlimited photosynthesis of the algae. On the other hand, experiments with different O2 concentrations in the medium have shown the photosyn- thetic efficiency is increased by 14% if almost no O2 is present in the medium but is reduced to about 35% when the medium is saturated with 100% O2. However, an- other study showed that oxygen concentrations ranging from 10 to 200% of ambient had no significant effects on daily net carbon gain or total wet biomass production rates in this particular seaweed [40]. Generally speaking, the effects of various combina- tions of CO2 concentration, light intensity and oxygen concentration on photosynthesis and growth in several algal types suggest the following. Different algae show different responses to high oxy- gen concentrations and high light intensities. Gener- ally, inhibition of photosynthesis (CO2 fixation and growth), increases with increasing oxygen concentra- tion and with increasing light intensity (at light inten- sities greater than saturation) [51-55]. This is very much in favor of using these biological systems for the production of biofuel in various climates. The environmental conditions play a determining role in promoting CO2 fixation and cellular propagation. For example, a hot spring alga (HSA) purified from an alkaline hot spring (pH 9.3 and 62˚C) in Taiwan grows well over pH 11.5 and 50˚C. For performance of HSA, CO2 removal efficiencies in the packed tower increase about 5-fold in a suitable growth con- dition compared to that without adding alkaline salt such as potassium hydroxide. In addition, HSA also exhibits a high growth rate under the controlled pHs from 7 to 11 [56]. Basic growth nutrients must be available in order to maintain proper physiological integration of the cul- ture. This can reasonably be overcome using waste- water [57-59]. Temperature can be a determining factor in the selec- tion of the algal species [60,61]. However, the diver- sity in the optimum temperature required to maintain the best growth rates makes it possible to choose the organism with given physical needs [62]. 6. Conclusions In considering the results of the studies described above, it would appear that super-elevated atmospheric CO2 concentrations are not detrimental to freshwater and ma- rine microalgae and macrophytes. In fact, they suggest that huge increases in aquatic CO2 concentration can sometimes lead to equally huge increases in aquatic plant growth. For the purpose of CO2 sequestration, the use of microalgae is a unique technology. In fact microalgae sit on the top of the choices for its exceptionally high effi- ciency in energy conversion, CO2 removal and the size and usefulness of other byproducts. The technology, which is an environmentally friendly, works under lim- ited O2 concentrations, and a wide range of thermal and light conditions. It requires selecting the proper type of microalgae and then adapting to higher CO2 concentra- tions that could have the potential to produce useful by- products, and function multi-purposely. REFERENCES [1] D. J. Stepan, R. E. Shockey, T. A. Moe and R. Dorn. “SUBTASK, 2.3-Carbon Dioxide Sequestering Using Microalgal Systems,” U.S. Department of Energy, Na- tional Energy Technology Laboratory, 2001. [2] B. Hileman, “U.S. Urged to Change CO2 Emissions Pol- icy,” Chemical Engineering News, Vol. 70, No. 1, 1992, pp. 16-22. doi:10.1021/cen-v070n008.p016 [3] O. Pulz and W. Gross, “Valuable Products from Bio- technology of Microalgae,” Applied Microbiology and Biotechnology, Vol. 65, No. 3, 2004, pp. 635-648. doi:10.1007/s00253-004-1647-x [4] Y. Chisti, “Biodiesel from Microalgae,” Biotechnology Advances, Vol. 25, No. 3, 2007, pp. 294-306. doi:10.1016/j.biotechadv.2007.02.001 [5] E. Ono and J. L. Cuello, “Selection of Optimal Microal- Copyright © 2011 SciRes. JEP
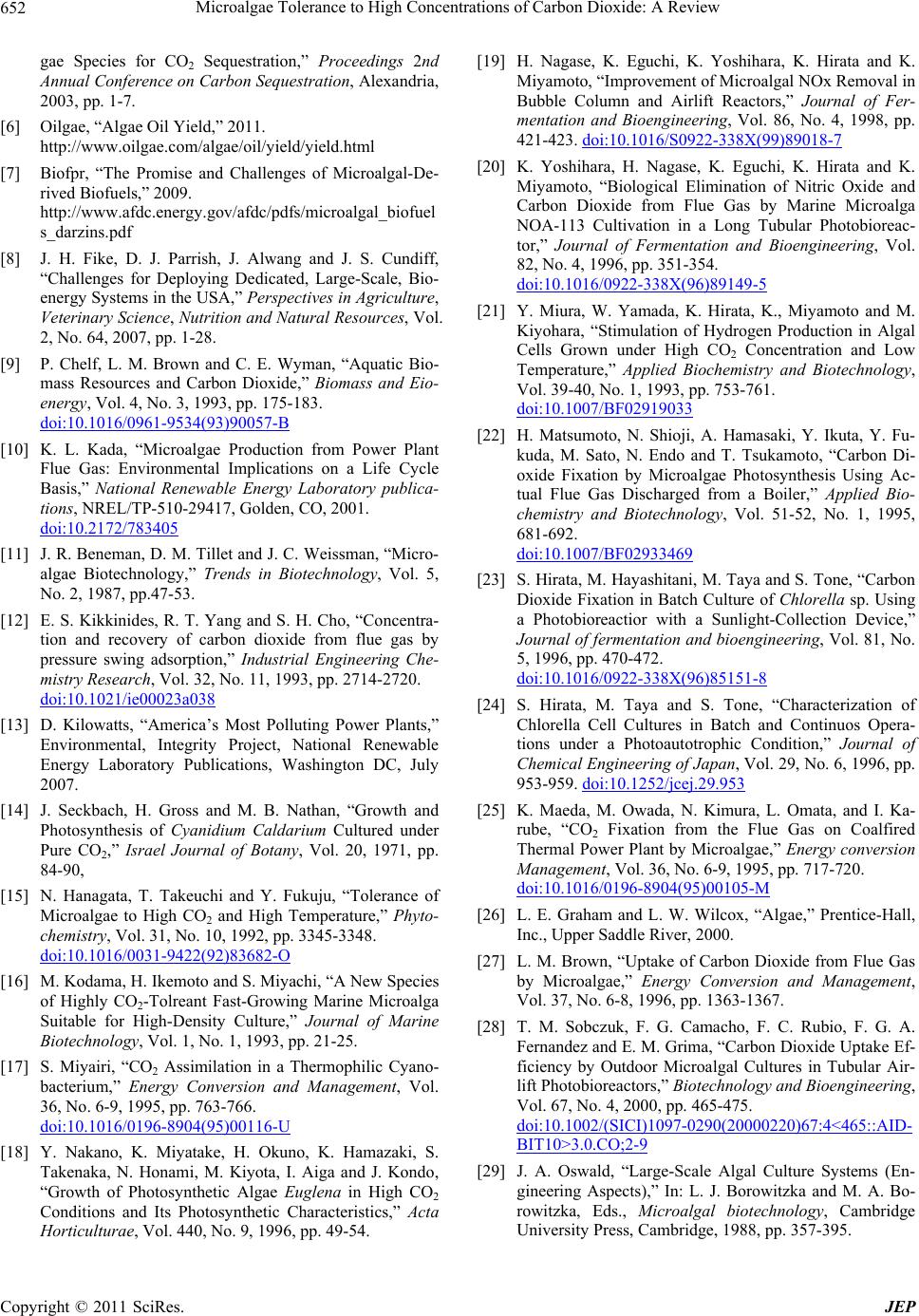 Microalgae Tolerance to High Concentrations of Carbon Dioxide: A Review 652 gae Species for CO2 Sequestration,” Proceedings 2nd Annual Conference on Carbon Sequestration, Alexandria, 2003, pp. 1-7. [6] Oilgae, “Algae Oil Yield,” 2011. http://www.oilgae.com/algae/oil/yield/yield.html [7] Biofpr, “The Promise and Challenges of Microalgal-De- rived Biofuels,” 2009. http://www.afdc.energy.gov/afdc/pdfs/microalgal_biofuel s_darzins.pdf [8] J. H. Fike, D. J. Parrish, J. Alwang and J. S. Cundiff, “Challenges for Deploying Dedicated, Large-Scale, Bio- energy Systems in the USA,” Perspectives in Agriculture, Veterinary Scienc e, Nutrition and Natural Resources, Vol. 2, No. 64, 2007, pp. 1-28. [9] P. Chelf, L. M. Brown and C. E. Wyman, “Aquatic Bio- mass Resources and Carbon Dioxide,” Biomass and Eio- energy, Vol. 4, No. 3, 1993, pp. 175-183. doi:10.1016/0961-9534(93)90057-B [10] K. L. Kada, “Microalgae Production from Power Plant Flue Gas: Environmental Implications on a Life Cycle Basis,” National Renewable Energy Laboratory publica- tions, NREL/TP-510-29417, Golden, CO, 2001. doi:10.2172/783405 [11] J. R. Beneman, D. M. Tillet and J. C. Weissman, “Micro- algae Biotechnology,” Trends in Biotechnology, Vol. 5, No. 2, 1987, pp.47-53. [12] E. S. Kikkinides, R. T. Yang and S. H. Cho, “Concentra- tion and recovery of carbon dioxide from flue gas by pressure swing adsorption,” Industrial Engineering Che- mistry Research, Vol. 32, No. 11, 1993, pp. 2714-2720. doi:10.1021/ie00023a038 [13] D. Kilowatts, “America’s Most Polluting Power Plants,” Environmental, Integrity Project, National Renewable Energy Laboratory Publications, Washington DC, July 2007. [14] J. Seckbach, H. Gross and M. B. Nathan, “Growth and Photosynthesis of Cyanidium Caldarium Cultured under Pure CO2,” Israel Journal of Botany, Vol. 20, 1971, pp. 84-90, [15] N. Hanagata, T. Takeuchi and Y. Fukuju, “Tolerance of Microalgae to High CO2 and High Temperature,” Phyto- chemistry, Vol. 31, No. 10, 1992, pp. 3345-3348. doi:10.1016/0031-9422(92)83682-O [16] M. Kodama, H. Ikemoto and S. Miyachi, “A New Species of Highly CO2-Tolreant Fast-Growing Marine Microalga Suitable for High-Density Culture,” Journal of Marine Biotechnology, Vol. 1, No. 1, 1993, pp. 21-25. [17] S. Miyairi, “CO2 Assimilation in a Thermophilic Cyano- bacterium,” Energy Conversion and Management, Vol. 36, No. 6-9, 1995, pp. 763-766. doi:10.1016/0196-8904(95)00116-U [18] Y. Nakano, K. Miyatake, H. Okuno, K. Hamazaki, S. Takenaka, N. Honami, M. Kiyota, I. Aiga and J. Kondo, “Growth of Photosynthetic Algae Euglena in High CO2 Conditions and Its Photosynthetic Characteristics,” Acta Horticulturae, Vol. 440, No. 9, 1996, pp. 49-54. [19] H. Nagase, K. Eguchi, K. Yoshihara, K. Hirata and K. Miyamoto, “Improvement of Microalgal NOx Removal in Bubble Column and Airlift Reactors,” Journal of Fer- mentation and Bioengineering, Vol. 86, No. 4, 1998, pp. 421-423. doi:10.1016/S0922-338X(99)89018-7 [20] K. Yoshihara, H. Nagase, K. Eguchi, K. Hirata and K. Miyamoto, “Biological Elimination of Nitric Oxide and Carbon Dioxide from Flue Gas by Marine Microalga NOA-113 Cultivation in a Long Tubular Photobioreac- tor,” Journal of Fermentation and Bioengineering, Vol. 82, No. 4, 1996, pp. 351-354. doi:10.1016/0922-338X(96)89149-5 [21] Y. Miura, W. Yamada, K. Hirata, K., Miyamoto and M. Kiyohara, “Stimulation of Hydrogen Production in Algal Cells Grown under High CO2 Concentration and Low Temperature,” Applied Biochemistry and Biotechnology, Vol. 39-40, No. 1, 1993, pp. 753-761. doi:10.1007/BF02919033 [22] H. Matsumoto, N. Shioji, A. Hamasaki, Y. Ikuta, Y. Fu- kuda, M. Sato, N. Endo and T. Tsukamoto, “Carbon Di- oxide Fixation by Microalgae Photosynthesis Using Ac- tual Flue Gas Discharged from a Boiler,” Applied Bio- chemistry and Biotechnology, Vol. 51-52, No. 1, 1995, 681-692. doi:10.1007/BF02933469 [23] S. Hirata, M. Hayashitani, M. Taya and S. Tone, “Carbon Dioxide Fixation in Batch Culture of Chlorella sp. Using a Photobioreactior with a Sunlight-Collection Device,” Journal of fermentation and bioengineering, Vol. 81, No. 5, 1996, pp. 470-472. doi:10.1016/0922-338X(96)85151-8 [24] S. Hirata, M. Taya and S. Tone, “Characterization of Chlorella Cell Cultures in Batch and Continuos Opera- tions under a Photoautotrophic Condition,” Journal of Chemical Engineering of Japan, Vol. 29, No. 6, 1996, pp. 953-959. doi:10.1252/jcej.29.953 [25] K. Maeda, M. Owada, N. Kimura, L. Omata, and I. Ka- rube, “CO2 Fixation from the Flue Gas on Coalfired Thermal Power Plant by Microalgae,” Energy conversion Management, Vol. 36, No. 6-9, 1995, pp. 717-720. doi:10.1016/0196-8904(95)00105-M [26] L. E. Graham and L. W. Wilcox, “Algae,” Prentice-Hall, Inc., Upper Saddle River, 2000. [27] L. M. Brown, “Uptake of Carbon Dioxide from Flue Gas by Microalgae,” Energy Conversion and Management, Vol. 37, No. 6-8, 1996, pp. 1363-1367. [28] T. M. Sobczuk, F. G. Camacho, F. C. Rubio, F. G. A. Fernandez and E. M. Grima, “Carbon Dioxide Uptake Ef- ficiency by Outdoor Microalgal Cultures in Tubular Air- lift Photobioreactors,” Biotechnology and Bioengineering, Vol. 67, No. 4, 2000, pp. 465-475. doi:10.1002/(SICI)1097-0290(20000220)67:4<465::AID- BIT10>3.0.CO;2-9 [29] J. A. Oswald, “Large-Scale Algal Culture Systems (En- gineering Aspects),” In: L. J. Borowitzka and M. A. Bo- rowitzka, Eds., Microalgal biotechnology, Cambridge University Press, Cambridge, 1988, pp. 357-395. C opyright © 2011 SciRes. JEP
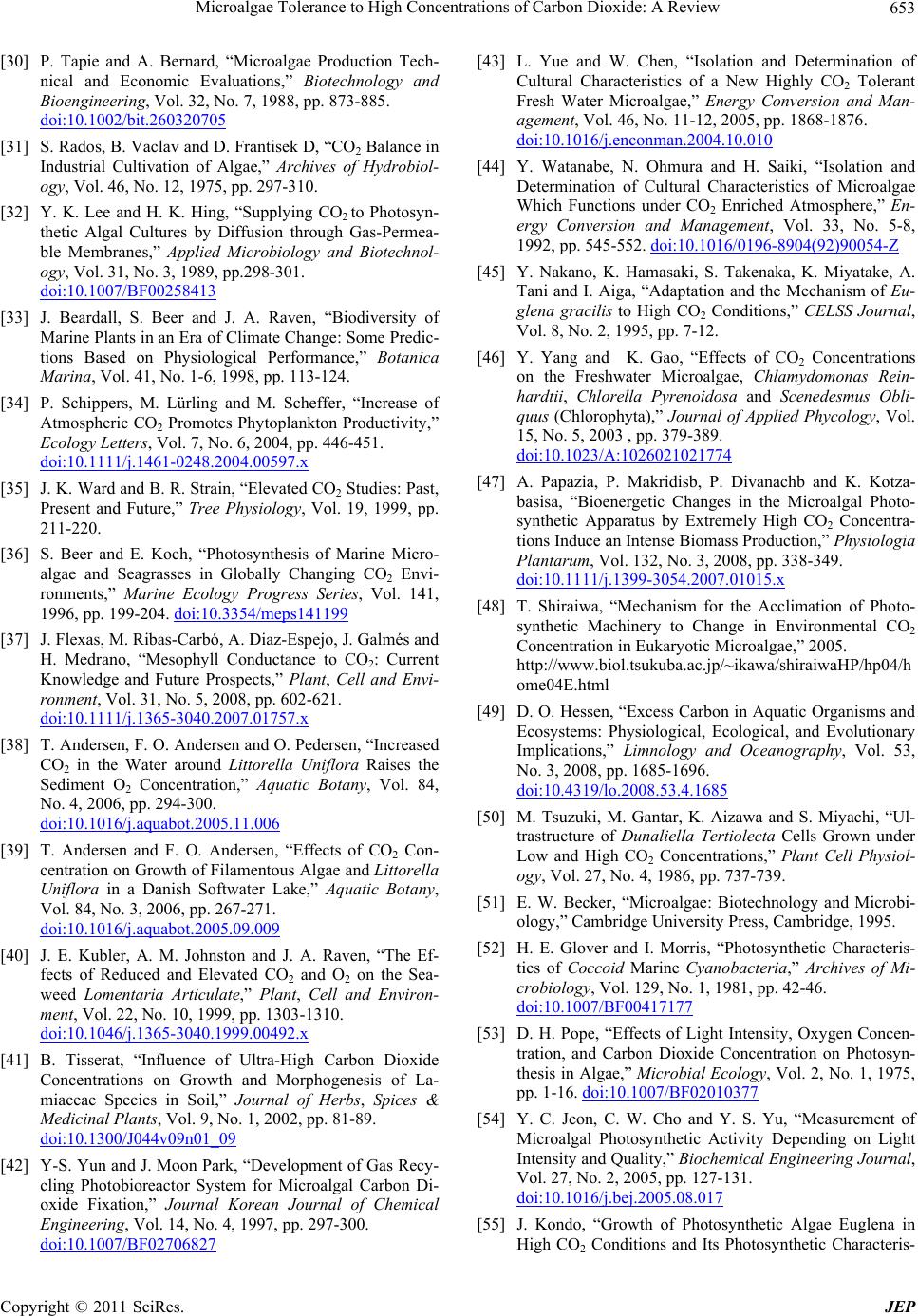 Microalgae Tolerance to High Concentrations of Carbon Dioxide: A Review653 [30] P. Tapie and A. Bernard, “Microalgae Production Tech- nical and Economic Evaluations,” Biotechnology and Bioengineering, Vol. 32, No. 7, 1988, pp. 873-885. doi:10.1002/bit.260320705 [31] S. Rados, B. Vaclav and D. Frantisek D, “CO2 Balance in Industrial Cultivation of Algae,” Archives of Hydrobiol- ogy, Vol. 46, No. 12, 1975, pp. 297-310. [32] Y. K. Lee and H. K. Hing, “Supplying CO2 to Photosyn- thetic Algal Cultures by Diffusion through Gas-Permea- ble Membranes,” Applied Microbiology and Biotechnol- ogy, Vol. 31, No. 3, 1989, pp.298-301. doi:10.1007/BF00258413 [33] J. Beardall, S. Beer and J. A. Raven, “Biodiversity of Marine Plants in an Era of Climate Change: Some Predic- tions Based on Physiological Performance,” Botanica Marina, Vol. 41, No. 1-6, 1998, pp. 113-124. [34] P. Schippers, M. Lürling and M. Scheffer, “Increase of Atmospheric CO2 Promotes Phytoplankton Productivity,” Ecology Letters, Vol. 7, No. 6, 2004, pp. 446-451. doi:10.1111/j.1461-0248.2004.00597.x [35] J. K. Ward and B. R. Strain, “Elevated CO2 Studies: Past, Present and Future,” Tree Physiology, Vol. 19, 1999, pp. 211-220. [36] S. Beer and E. Koch, “Photosynthesis of Marine Micro- algae and Seagrasses in Globally Changing CO2 Envi- ronments,” Marine Ecology Progress Series, Vol. 141, 1996, pp. 199-204. doi:10.3354/meps141199 [37] J. Flexas, M. Ribas-Carbó, A. Diaz-Espejo, J. Galmés and H. Medrano, “Mesophyll Conductance to CO2: Current Knowledge and Future Prospects,” Plant, Cell and Envi- ronment, Vol. 31, No. 5, 2008, pp. 602-621. doi:10.1111/j.1365-3040.2007.01757.x [38] T. Andersen, F. O. Andersen and O. Pedersen, “Increased CO2 in the Water around Littorella Uniflora Raises the Sediment O2 Concentration,” Aquatic Botany, Vol. 84, No. 4, 2006, pp. 294-300. doi:10.1016/j.aquabot.2005.11.006 [39] T. Andersen and F. O. Andersen, “Effects of CO2 Con- centration on Growth of Filamentous Algae and Littorella Uniflora in a Danish Softwater Lake,” Aquatic Botany, Vol. 84, No. 3, 2006, pp. 267-271. doi:10.1016/j.aquabot.2005.09.009 [40] J. E. Kubler, A. M. Johnston and J. A. Raven, “The Ef- fects of Reduced and Elevated CO2 and O2 on the Sea- weed Lomentaria Articulate,” Plant, Cell and Environ- ment, Vol. 22, No. 10, 1999, pp. 1303-1310. doi:10.1046/j.1365-3040.1999.00492.x [41] B. Tisserat, “Influence of Ultra-High Carbon Dioxide Concentrations on Growth and Morphogenesis of La- miaceae Species in Soil,” Journal of Herbs, Spices & Medicinal Plan ts, Vol. 9, No. 1, 2002, pp. 81-89. doi:10.1300/J044v09n01_09 [42] Y-S. Yun and J. Moon Park, “Development of Gas Recy- cling Photobioreactor System for Microalgal Carbon Di- oxide Fixation,” Journal Korean Journal of Chemical Engineering, Vol. 14, No. 4, 1997, pp. 297-300. doi:10.1007/BF02706827 [43] L. Yue and W. Chen, “Isolation and Determination of Cultural Characteristics of a New Highly CO2 Tolerant Fresh Water Microalgae,” Energy Conversion and Man- agement, Vol. 46, No. 11-12, 2005, pp. 1868-1876. doi:10.1016/j.enconman.2004.10.010 [44] Y. Watanabe, N. Ohmura and H. Saiki, “Isolation and Determination of Cultural Characteristics of Microalgae Which Functions under CO2 Enriched Atmosphere,” En- ergy Conversion and Management, Vol. 33, No. 5-8, 1992, pp. 545-552. doi:10.1016/0196-8904(92)90054-Z [45] Y. Nakano, K. Hamasaki, S. Takenaka, K. Miyatake, A. Tani and I. Aiga, “Adaptation and the Mechanism of Eu- glena gracilis to High CO2 Conditions,” CELSS Journal, Vol. 8, No. 2, 1995, pp. 7-12. [46] Y. Yang and K. Gao, “Effects of CO2 Concentrations on the Freshwater Microalgae, Chlamydomonas Rein- hardtii, Chlorella Pyrenoidosa and Scenedesmus Obli- quus (Chlorophyta),” Journal of Applied Phycology, Vol. 15, No. 5, 2003 , pp. 379-389. doi:10.1023/A:1026021021774 [47] A. Papazia, P. Makridisb, P. Divanachb and K. Kotza- basisa, “Bioenergetic Changes in the Microalgal Photo- synthetic Apparatus by Extremely High CO2 Concentra- tions Induce an Intense Biomass Production,” Physiologia Plantarum, Vol. 132, No. 3, 2008, pp. 338-349. doi:10.1111/j.1399-3054.2007.01015.x [48] T. Shiraiwa, “Mechanism for the Acclimation of Photo- synthetic Machinery to Change in Environmental CO2 Concentration in Eukaryotic Microalgae,” 2005. http://www.biol.tsukuba.ac.jp/~ikawa/shiraiwaHP/hp04/h ome04E.html [49] D. O. Hessen, “Excess Carbon in Aquatic Organisms and Ecosystems: Physiological, Ecological, and Evolutionary Implications,” Limnology and Oceanography, Vol. 53, No. 3, 2008, pp. 1685-1696. doi:10.4319/lo.2008.53.4.1685 [50] M. Tsuzuki, M. Gantar, K. Aizawa and S. Miyachi, “Ul- trastructure of Dunaliella Tertiolecta Cells Grown under Low and High CO2 Concentrations,” Plant Cell Physiol- ogy, Vol. 27, No. 4, 1986, pp. 737-739. [51] E. W. Becker, “Microalgae: Biotechnology and Microbi- ology,” Cambridge University Press, Cambridge, 1995. [52] H. E. Glover and I. Morris, “Photosynthetic Characteris- tics of Coccoid Marine Cyanobacteria,” Archives of Mi- crobiology, Vol. 129, No. 1, 1981, pp. 42-46. doi:10.1007/BF00417177 [53] D. H. Pope, “Effects of Light Intensity, Oxygen Concen- tration, and Carbon Dioxide Concentration on Photosyn- thesis in Algae,” Microbial Ecology, Vol. 2, No. 1, 1975, pp. 1-16. doi:10.1007/BF02010377 [54] Y. C. Jeon, C. W. Cho and Y. S. Yu, “Measurement of Microalgal Photosynthetic Activity Depending on Light Intensity and Quality,” Biochemical Engineering Journal, Vol. 27, No. 2, 2005, pp. 127-131. doi:10.1016/j.bej.2005.08.017 [55] J. Kondo, “Growth of Photosynthetic Algae Euglena in High CO2 Conditions and Its Photosynthetic Characteris- Copyright © 2011 SciRes. JEP
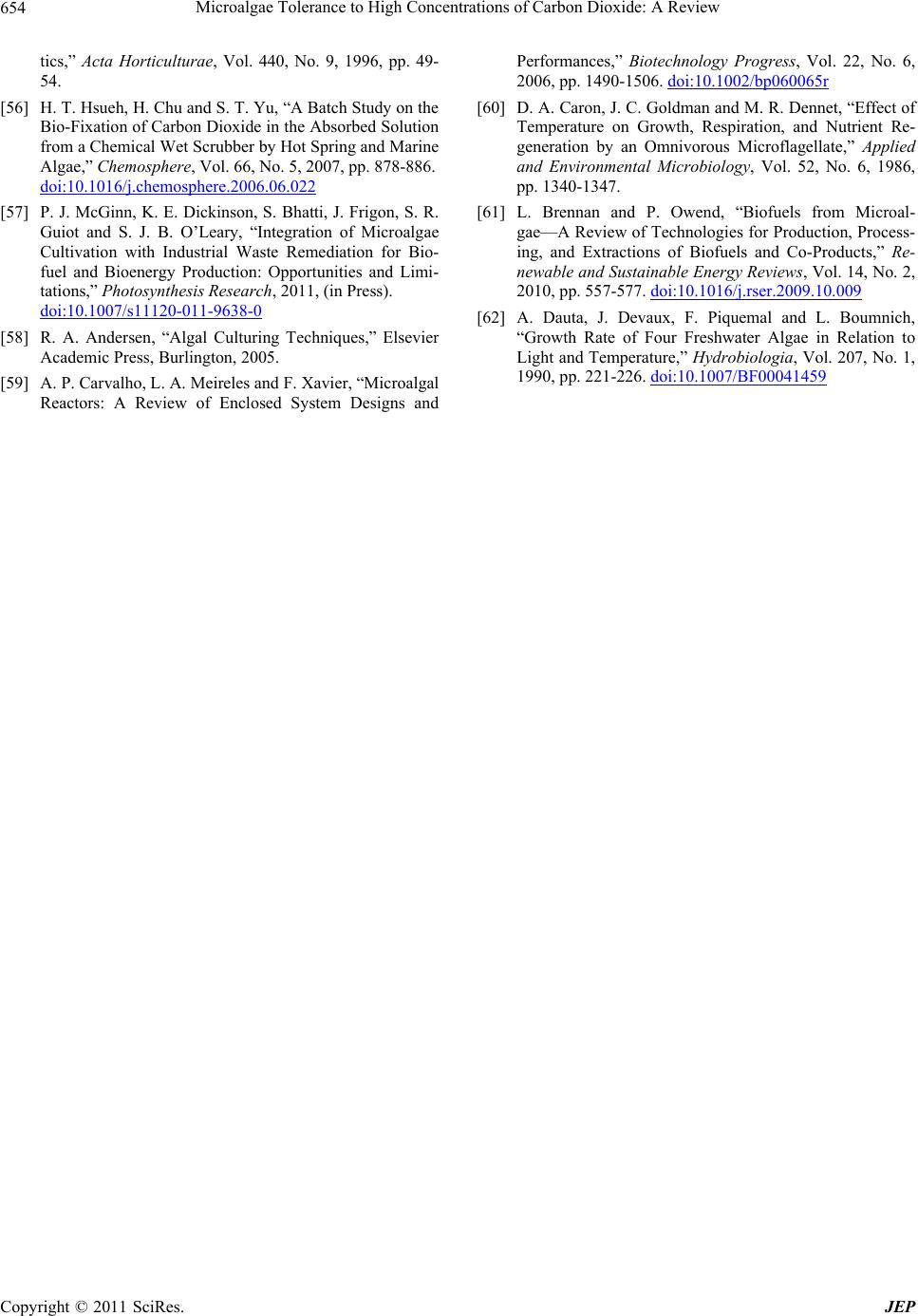 Microalgae Tolerance to High Concentrations of Carbon Dioxide: A Review Copyright © 2011 SciRes. JEP 654 tics,” Acta Horticulturae, Vol. 440, No. 9, 1996, pp. 49- 54. [56] H. T. Hsueh, H. Chu and S. T. Yu, “A Batch Study on the Bio-Fixation of Carbon Dioxide in the Absorbed Solution from a Chemical Wet Scrubber by Hot Spring and Marine Algae,” Chemosphere, Vol. 66, No. 5, 2007, pp. 878-886. doi:10.1016/j.chemosphere.2006.06.022 [57] P. J. McGinn, K. E. Dickinson, S. Bhatti, J. Frigon, S. R. Guiot and S. J. B. O’Leary, “Integration of Microalgae Cultivation with Industrial Waste Remediation for Bio- fuel and Bioenergy Production: Opportunities and Limi- tations,” Phot osynthesis Research, 2011, (in Press). doi:10.1007/s11120-011-9638-0 [58] R. A. Andersen, “Algal Culturing Techniques,” Elsevier Academic Press, Burlington, 2005. [59] A. P. Carvalho, L. A. Meireles and F. Xavier, “Microalgal Reactors: A Review of Enclosed System Designs and Performances,” Biotechnology Progress, Vol. 22, No. 6, 2006, pp. 1490-1506. doi:10.1002/bp060065r [60] D. A. Caron, J. C. Goldman and M. R. Dennet, “Effect of Temperature on Growth, Respiration, and Nutrient Re- generation by an Omnivorous Microflagellate,” Applied and Environmental Microbiology, Vol. 52, No. 6, 1986, pp. 1340-1347. [61] L. Brennan and P. Owend, “Biofuels from Microal- gae—A Review of Technologies for Production, Process- ing, and Extractions of Biofuels and Co-Products,” Re- newable and Sustainable Energy Reviews, Vol. 14, No. 2, 2010, pp. 557-577. doi:10.1016/j.rser.2009.10.009 [62] A. Dauta, J. Devaux, F. Piquemal and L. Boumnich, “Growth Rate of Four Freshwater Algae in Relation to Light and Temperature,” Hydrobiologia, Vol. 207, No. 1, 1990, pp. 221-226. doi:10.1007/BF00041459
|