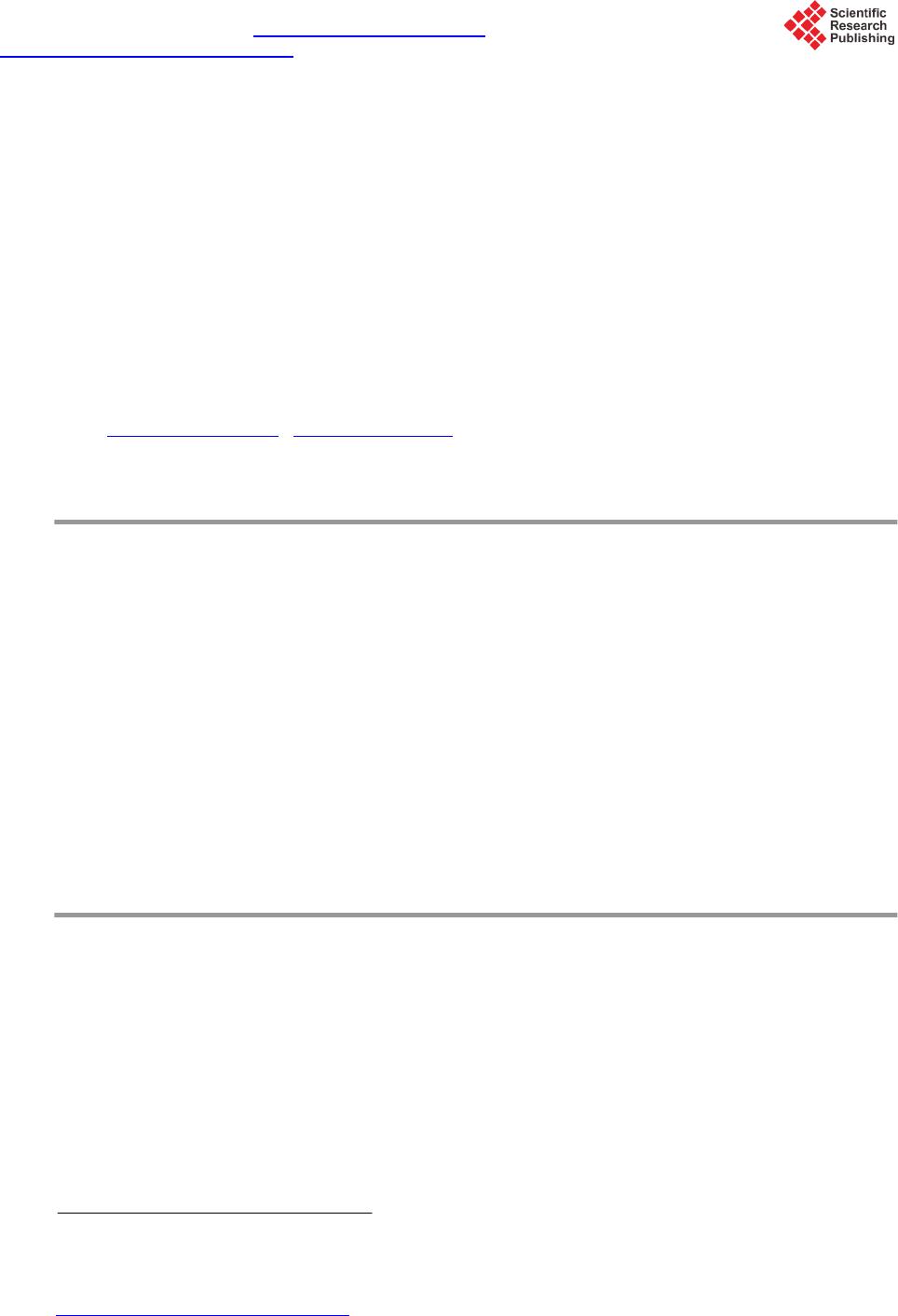 Journal of Geoscience and Environment Protection, 2015, 3, 54-61 Published Online July 2015 in SciRes. http://www.scirp.org/journal/gep http://dx.doi.org/10.4236/gep.2015.35007 How to cite this paper: Wang, E.D., Han, C., Xia, J.M. and Yun, S. (2015) Geochemistry and Tectonic Significance of Chlorite Amphibolite in Nanfen BIF, Benxi Area, Northeastern China. Journal of Geoscience and Environment Protection, 3, 54-61. http://dx.doi.org/10.4236/gep.2015.35007 Geochemistry and Tectonic Significance of Chlorite Amphibolite in Nanfen BIF, Benxi Area, Northeastern China Ende Wang1, Changik Han1,2*, Jianming Xia1, Sunggi Yun2 1College of Resources & Civil, Northeastern University, Shenyang, China 2College of Geoexploration Engineering, Kimchaek University of Technology, Pyongyang, DPR of Korea Email: wnd@mail.neu.edu.cn, *han_6130@sina.com Received 29 Ma rch 2015; accepted 10 July 2015; published 17 July 2015 Abstract Benxi area, Northeastern China, is the main distribution area of Archean BIF-hosted iron deposits in China. In this area, Nanfen iron deposit is well known as the largest open-pit iron deposit not only in China but also in Asia. So far, the tectonic nature during Archean BIF formation period in Benxi area has been long disputed and the tectonic setting of Nanfen BIF had not been found. In this study, the geochemical characters of chlorite amphibolites closely associated with BIF have been investigated for the tectonic environment of Nanfen BIF. Chlortie amphibolites show the geochemical affinity to the back-arc basin basalt (BABB), indicating that the tectonic environment of Nanfen BIF is the back-arc basin. In conjunction with geological evidence of other BIFs at Benxi area, it is identified that BIF in Benxi area might be formed in the subduction-related back-arc basin, which provides a favorable sedimentary environment of Algoma-type BIF. Keywords Chlorite Amphibolite, BAB B, Nanfen BIF, Tectonic Environment, Northeastern China 1. Introduction Iron deposits from Benxi area including Gongchangling, Nanfen and Waitoushan are the typical BIF-hosted de- posits in Northeastern China, which hosted within the Neoarchean middle Anshan Group. Recent studies of the iron deposits from Benxi area have formed the consistent view that they belong to Algoma-type BIF, but have the controversy about the tectonic nature during Archean BIF formation period because of the multiple deforma- tion and metamorphic processes. Some of scholar considered that BIF produced in mid-oceanic ridge and others thought that it produced in an island are or back-arc basin (Dai, Zhang, Wang, Liu, Cui, Zhu, & Xiang, 2012; Feng, Zhou, & Xiao, 2009; Liu, & Jin, 2010; Cui, Sun, Zhang, Gu, Zhou, & Sun, 2012; Zhang, Yang, Zhou, & Shi, 2013; Sun, Zhu, Tang, Zhang, & Han, 2014; Wang, Xia, Zhao, Fu, & Hou et al., 2013) [1]-[7]. In addition, the known reports on the Nanfen BIF is small while the detailed study on the Gongchangling and Waitoushan
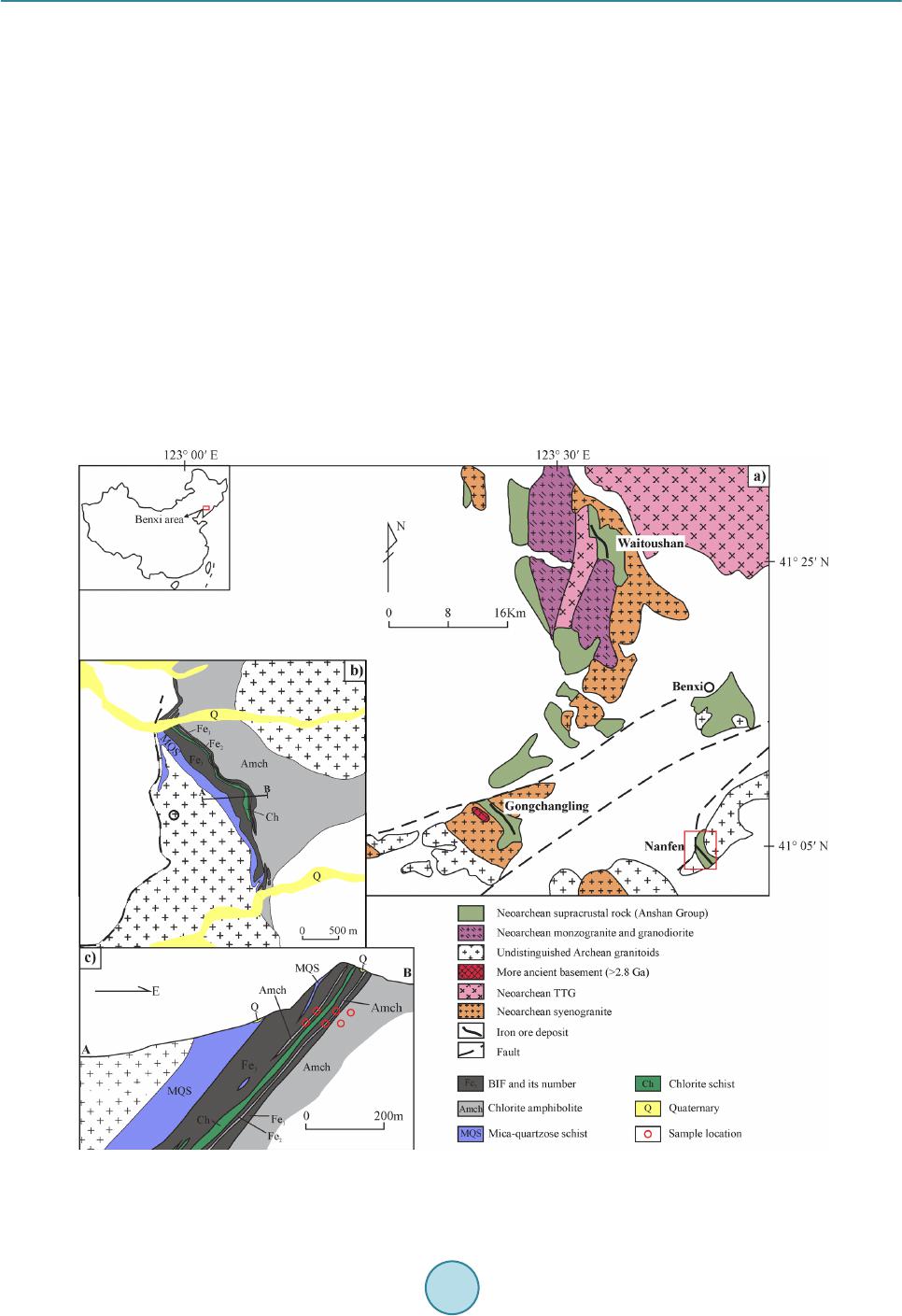 E. D. Wang et al. BIF-hosted iron deposits is carried out by many geologists. In fact, despite extensive research on BIF around the world in the past few decades, many aspects of their ori- gin including tectonic setting remain unresolved (Bekker, Slack, Planavsky, Krapez, Hofmann, Konhauser, & Rouxel, 2010) [8]. Alogma-type BIF are closely associated with volcanic activity (Gross, 1980) [9], and so geochemical features of their volcanic wall rocks are widely used to constrain the tectonic setting of BIF. Chlo- rite amphibolites are important metamorphic volcanic rock of the middle Anshan Group stratigraphy and are in contact with BIF in the Nanfen iron deposit. In view of the close links between Algoma-type BIF and subjacent volcanism, this study has focused on es- tablishing the nature and distribution of metamorphic volcanic rock within the middle Anshan Group of Nanfen iron deposit. We report and discuss in this paper the results of a geochemical study on chlorite amphibolites from the Nanfen iron deposit in detail, and also reveal its tectonic significance for the formation environment of the BIF in the study area. 2. Regional Geologic Background The Benxi iron producing area is located in Archean greenstone belts of the northeastern margin of China. The strata in this area included Archean Anshan Group, Proterozoic Liaohe Group, Neoproterozoic, Paleozoic, Me- sozoic and Neozoic strata. Archean geological bodies are exposed in Gongchangling, Nanfen and Waitoushan area etc., and are mainly composed of about 30% iron-bearing rock series and 70% granitic rocks (Figure 1). Figure 1. (a) Simplified geologic map of Benxi area (modified after Dai et al., 2012 [1]); (b) geologic map of Nanfen iron deposit and (c) stratigraphic section with sample location (modified after Zhou, 1994 [11]).
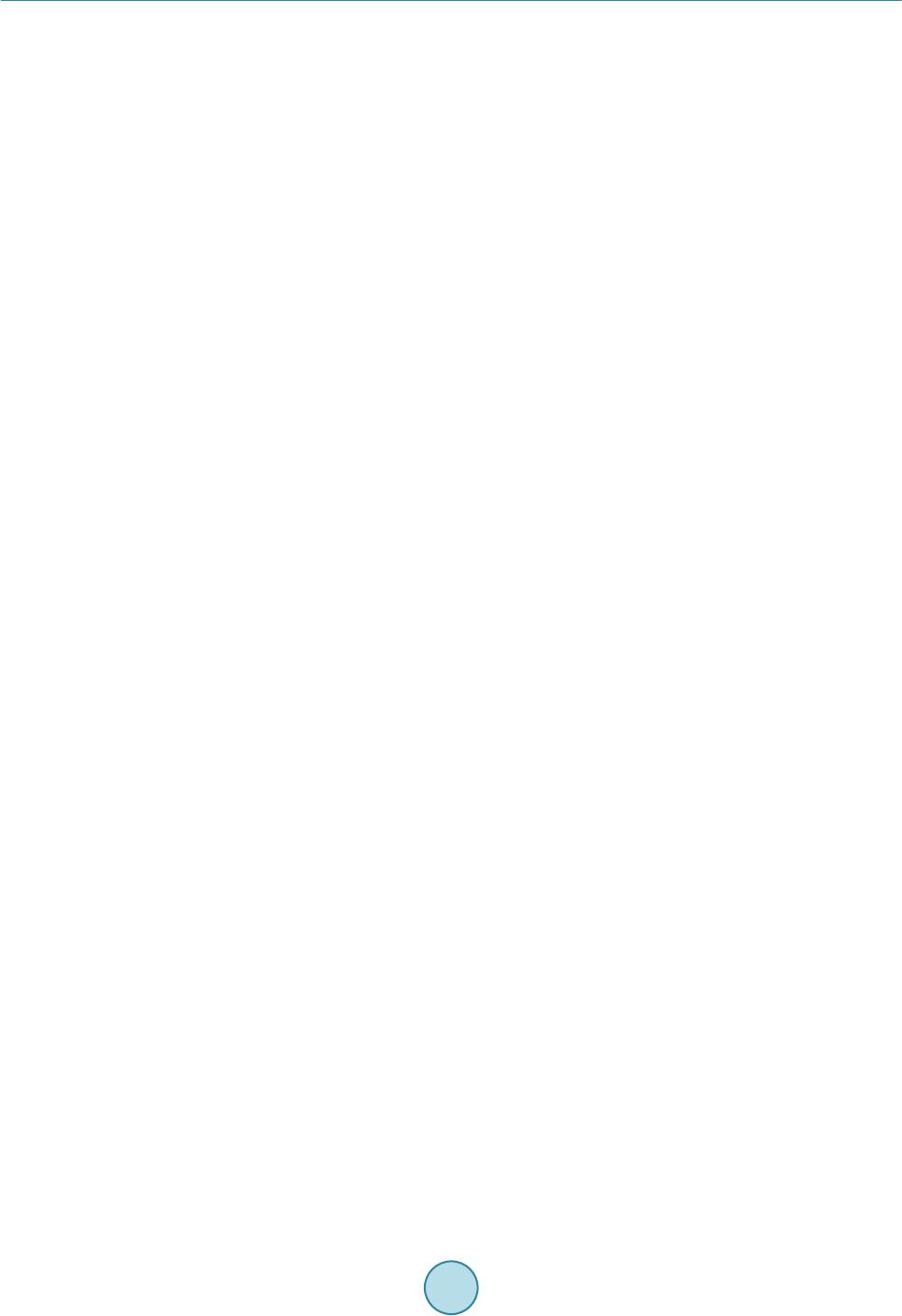 E. D. Wang et al. The Anshan Group strata are hosted between two phases of Archean granite, which have ages of about 3.0 Ga and about 2.45 Ga respectively, as “islands” (Z ha i & Sa nt o sh, 2011; Zhou, 1994) [10] [11]. The iron-bearing rock series is restricted between these two phases of granite emplacement. BIFs in the Benxi area occur in the Archean middle Anshan Group which is mostly distributed from Benxi to Liaoyang area, and their formation age mainly concentrate between 2.50 - 2.55 Ga (Dai, Zhang, Wang, Liu, Cui, Zhu, & Xiang, 2012; Wan, Dong, Xie, Wang, Song, & Xu et al., 2012) [1] [12]. Lithologically the middle Anshan Group is made up mainly of amphibolites, amphibole-bearing gneiss and biotite leptynite, also interbedded with muscovite quartz schist, and chlorite quartz schist (Zhou, 1994) [11]. This iron-bearing rock series is characterized by multi-layers of iron ore, iron minerals dominated by oxide with minor silicate and a general close association with meta-basalt owing to greenschist-lower amphibolite facies metamorphism, which includes the Gongchangling, Nanfen and Waitou- shan iron deposits, etc. (Zhou, 1994) [11]. At the Gongchangling iron ore deposit, the iron-bearing rock series can be divided into six units from bottom to top, which include the bottom amphibolite, bottom schist, lower iron ore (composed of the first layer of iron ore, the schist and the second layer of iron ore), biotite leptynite zone (interlayer with the third layer of iron ore), upper iron ore (composed of fourth layer of iron ore, lower plagioclase amphibolite, the fifth layer of iron ore, upper plagioclase amphibolites and the sixth layer of iron ore) and siliceous rocks (Wang, Xia, Zhao, Fu, & Hou, 2013; Sun, Zhu, Tang, Zhang, Luo, & Han, 2014; Zhou, 1994) [6] [7] [11]. Among them, the main ore types in- clude the low-grade magnetite quartzite, high-grade magnetite quartzite and some of low-grade martite ore. At the Waitoushan iron deposit, the iron-bearing rock series is mainly composed of a suite of plagioclase amphibo- lites, magnetite quartzite, actinolite quartz schist, actinolite quartzite, and garnet biotite actinolite schist. The iron ore body can be divided into three layers of magnetitie quartzite, which are mainly hosted by plagioclase amphibolitic rocks (Dai, Zhang, Wang, Liu, Cui, Zhu, & Xiang, 2012) [1]. Nanfen iron deposit is in super large scale with simple ore body shape and evenly distributed useful compo- nents. This consist of three roughly paralleled ore bodies trending to NW-SE and dipping to the SW at 40˚ - 50˚, which occurs as bedded or stratoid structure interbedded with the chlorite schists and chlorite amphibolites (Figure 1). Their ore-preserving roof wall rock is mica quartz schist and their ore-preserving floor wall rock is chlorite amphibolite and others. The mainly ore mineral is magnetite quartz (Zhou, 1994) [11]. It is clear from the above-mentioned geological analysis that the metamorphic volcanic rocks are hosted by many petrographic varieties of amphibolites (i.e. mainly the plagioclase amphibolites in Gongchangling and Waitoushan, the chlorite amphibolites in Nanfen), which share the same tectonic and metamorphic history. So it can be argued convincingly that amphibolites and iron ore are integral parts of the middle Anshan Group and were therefore formed during the same geological event. Clearly the thinking has changed regarding the prove- nance of the amphibolites in the middle Anshan Group and further work was regarded as being of utmost im- portance to better characterize the Benxi iron ore deposits. 3. Sample and Analytical Methods Six samples of chlorite amphibolite were collected from the Nanfen iron deposit, covering the interval from the lower part of Fe1 ore layer to Fe3 ore layer. Two came from the lower part of the succession associated with the Fe1 ore layer and other two between Fe1 ore layer and Fe2 ore layer, while the rest are from the interbeds be- tween F3 ore layer (Figure 1(c)). In outcrop the chlorite amphibolites of Nanfen iron deposit are generally cela- don or green (Fig ure 2(a)) and possess a fine grained granoblastic texture as well as a massive structure. Miner- al composition is dominated by hornblende and chlorite (Figure 2(b) & Figure 2(c)), minor minerals include epidote, quartz, plagioclase and biotite and others. All samples were processed and analysed by the Australian Laboratory Services Chemx (Guangzhou) Co. Ltd. For the whole-rock major element analyses, ME-XRF06 assay is used (lithium tetra-metaborate fusion, followed by X-Ray Fluorescence Spectroscopy; detection range of each oxide −0.01% - 100%). Trace element analyses were performed by four-acid digestion (perchloric, nitric, hydrofluoric and hydrochloric), with the dried residue taken up in dilute hydrochloric acid and analysed by ICP-AES. Rare earth element analyses were performed by ME-ICP161 assay (ICP-MS on lithium metaborate fusion beads). The accuracy for the trace element and rare earth element results were both better than 10%RSD. The analytical results are listed in Table 1. 4. Geochemical Characters The studied chlorite amphibolites display small variations in major oxide compositions, with SiO2 = 47.04 -
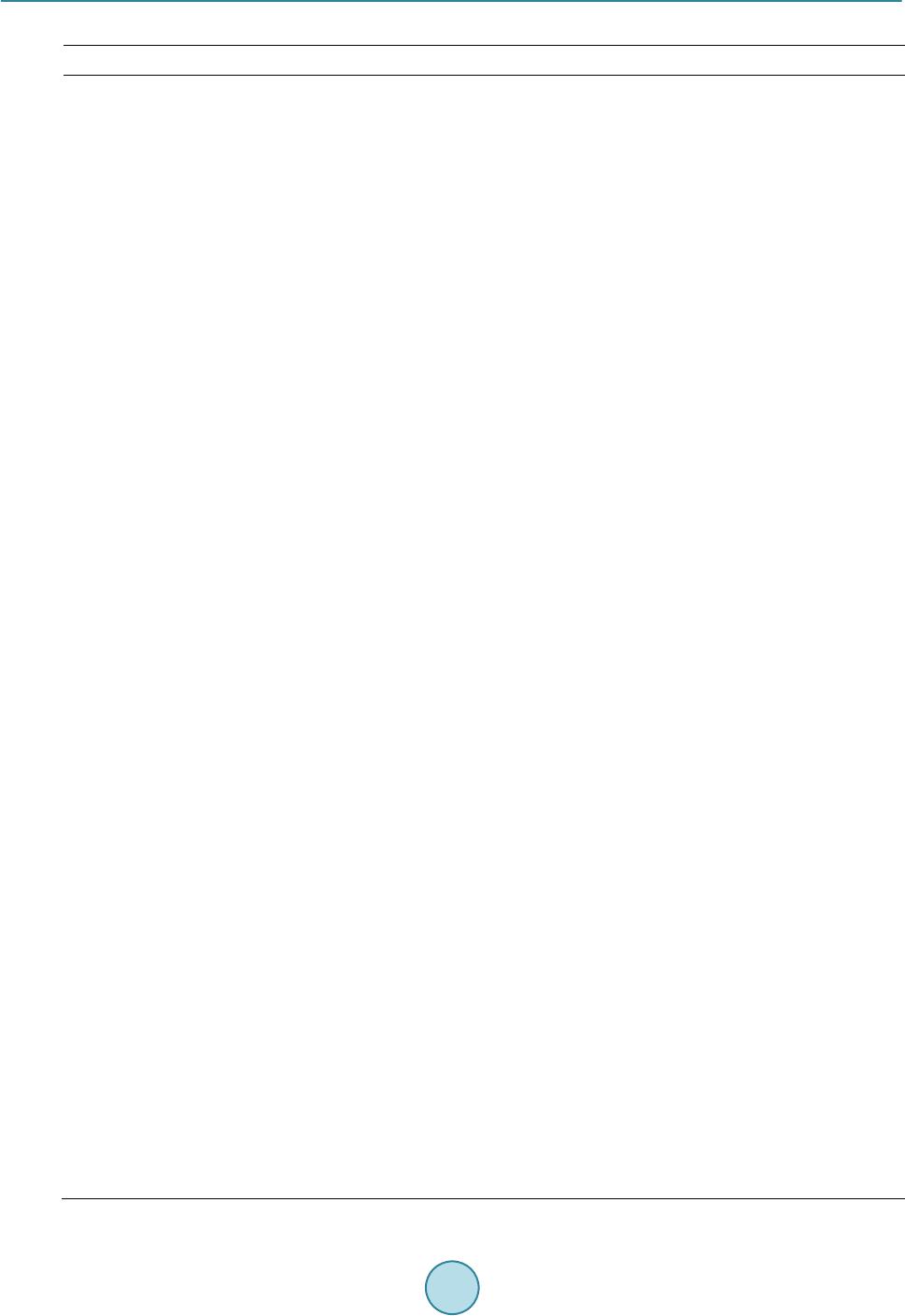 E. D. Wang et al. Table 1. Major (wt.%) and trace element (ppm) compositions of chlorite amphibolites from Nanfen iron deposit. Samples NF-1 NF-2 NF-3 NF-4 NF-5 NF-6 SiO2 49. 85 48.64 49.35 48.04 47.04 47.19 TiO2 0.85 0.77 0.80 0.79 0.86 0.90 Al2O3 14.73 14.29 14.37 15.02 15.44 15.97 FeOt 11.69 11.78 11.66 1 1.69 12.24 11.78 MnO 0.19 0.20 0.20 0.18 0.20 0.19 MgO 7.86 8.61 8.40 7.92 8.79 7.70 CaO 10.69 9.57 10.20 9.38 9.93 11.37 Na2O 0.62 1.18 1.03 1.37 1.10 0.64 K2O 0.04 0.13 0.05 0.73 0.17 0.15 P2O5 0.07 0.06 0.06 0.05 0.06 0.06 LOI 3.62 3.85 3.49 3.90 4.29 4.22 Total 100.22 99.08 99.62 99. 06 100.13 100.19 Cr 100 138 1 39 155 159 157 V 282 310 305 306 326 330 Sc 35. 60 38.60 39.60 38.0 4 0.80 41.50 Rb 4.00 4.40 4.20 34.40 5.80 4.90 Ba 21.00 71. 00 31.00 239.00 72.00 59.00 Th 0.56 0.50 0.50 0.52 0.52 0.46 U 0.19 0.17 0.18 0.36 0.20 0.15 Nb 2.60 2.30 2.30 2.30 2.30 2.00 Ta 0.17 0.13 0.15 0.13 0.14 0.14 Pb 5.40 13.00 7.00 9.30 5.60 8.60 Sr 109.00 88.20 95.70 77.80 95.70 134.00 Zr 57.00 48. 00 49.00 45. 00 54.00 49.00 Hf 1.50 1.40 1.40 1.20 1.50 1.40 La 3.70 2.80 3.00 2.80 2.90 2.90 Ce 9.52 7.61 8.39 7.71 7.95 7.91 Pr 1.39 1.28 1.36 1.24 1.42 1.20 Nd 7.10 6.50 6.60 6.00 6.80 5.80 Sm 2.39 2.19 2.21 2.01 2.43 2.00 Eu 0.78 0.72 0.68 0.84 0.73 0.78 Gd 2.88 2.87 2.77 2.65 3.16 2.40 Tb 0.53 0.50 0.50 0.46 0.54 0.50 Dy 3.58 3.31 3.41 3.25 3.46 3.32 Ho 0.73 0.75 0.71 0.71 0.76 0.72 Er 2.45 2.12 2.10 2.11 2.19 2.24 Tm 0.35 0.34 0.32 0.28 0.31 0.35 Yb 2.11 2.00 2.06 1.91 2.12 2.12 Lu 0.33 0.31 0.33 0.26 0.30 0.29
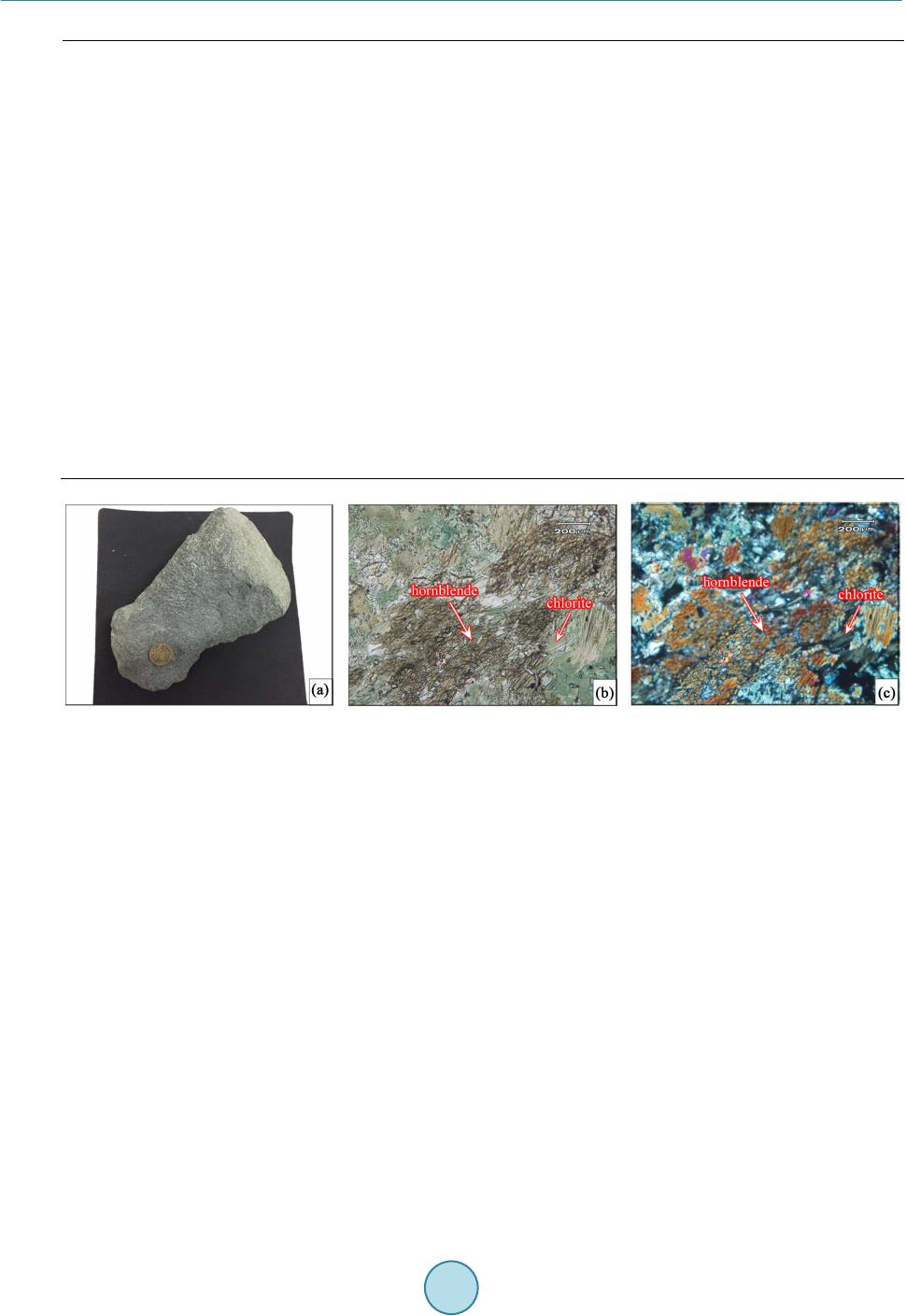 E. D. Wang et al. Conti n ue d Y 19.40 16.30 16. 80 16.00 16.40 1 7.90 ∑REE 37. 84 33.30 34. 44 32.03 35.07 32.53 ( La / Y b ) CN 1.26 1.00 1.04 1.05 0.98 0.98 (Gd/Yb)CN 1.13 1.19 1.11 1.15 1.23 0.94 Eu/Eu* 0.91 0.88 0.84 0.85 0.81 1.09 Ce/Ce* 1.03 0.98 1.02 1.01 0.96 1.04 (N b / La ) N 0.68 0.79 0.74 0.79 0.76 0.66 (T h /La ) N 1.22 1.44 1.35 1.50 1.45 1.28 (Hf/Sm)N 0.90 0.92 0.91 0.86 0.89 1.01 Nb /La 0.70 0.82 0.77 0.82 0.79 0.69 B a / La 5.68 25.36 1 0.33 85.36 24.83 20.34 Sm/Nd 0.34 0.34 0.33 0.34 0.36 0.34 Zr/Nb 2 1.92 20.87 2 1.30 19.57 23. 48 24.50 Zr/Y 2.94 2.94 2.92 2.81 3.29 2.74 Nb/Ta 15.29 17.69 1 5.33 17.69 16.43 14.29 Figure 2. Chlorite amphibolites: (a) hand specimen; (b) plane polarization; (c) cross polarization. 49.85 wt.%, MgO = 7.70 - 8.79 wt.%, Al2O3 = 14.29 - 15.97 wt.%, FeOt = 11.66 - 12.24 wt.%, CaO = 9.38 - 11.37 wt.%, Na2O + K2O = 0.66 - 2.10 wt.% and TiO2 = 0.77 - 0.90 wt.% (Table 1), and are plot into the basalt fields in the diagram of SiO2 versus K2O + Na2O (Figure 3(a)). The chondrite-normalized REE patterns for chlorite amphibolites are shown in Figure 3(b). As shown in Figure 3(b), the total REE contents are relatively low and samples exhibits nearly flat patterns with (La/Yb)CN = 0.98 - 1.26, (Gd/Yb)CN = 0.94 - 1.23 and insignificant Eu and Ce anomaly (Eu/Eu* = 0.81 - 1.09; Ce/Ce* = 0.96 - 1.04). On the primitive mantle-normalized spidergram (Figure 3(c)), the chlorite amphibolites are characterized by subparallel patterns with the weakly enrichment in LILE (e.g. Th, U and Pb) and depletion in HFSE (e.g. Nb-Ta, Zr-Hf, Ti and Y), where samples have (Nb/La)N = 0.66 - 0.79, (Th/La)N = 1.22 - 1.50 and (Hf/Sm)N = 0.86 - 1.01 (Table 1). 5. Protolith Reconstruction The Nanfen basaltic samples have relatively high LOI, suggesting that these rocks might have undergone some degree of alteration. It is essential to determine the effects of alteration in Archean metamorphic volcanic rocks . During alteration and metamorphism, the LILE such as Cs, Na, K, Rb, Ba, Ca and Sr are typically mobile. However, the some of major elements such as Al, Ti, Fe, the HFSE (Nb, Ta, Zr and Hf) and REE (except Eu) and others are poorly affected by alteration (Zhang, Wang, Fan, Zhang, & Yang, 2012) [13]. In fact, the REE and HFSE in Nanfen samples show coherent and subparallel concentration patterns, indicating that they still
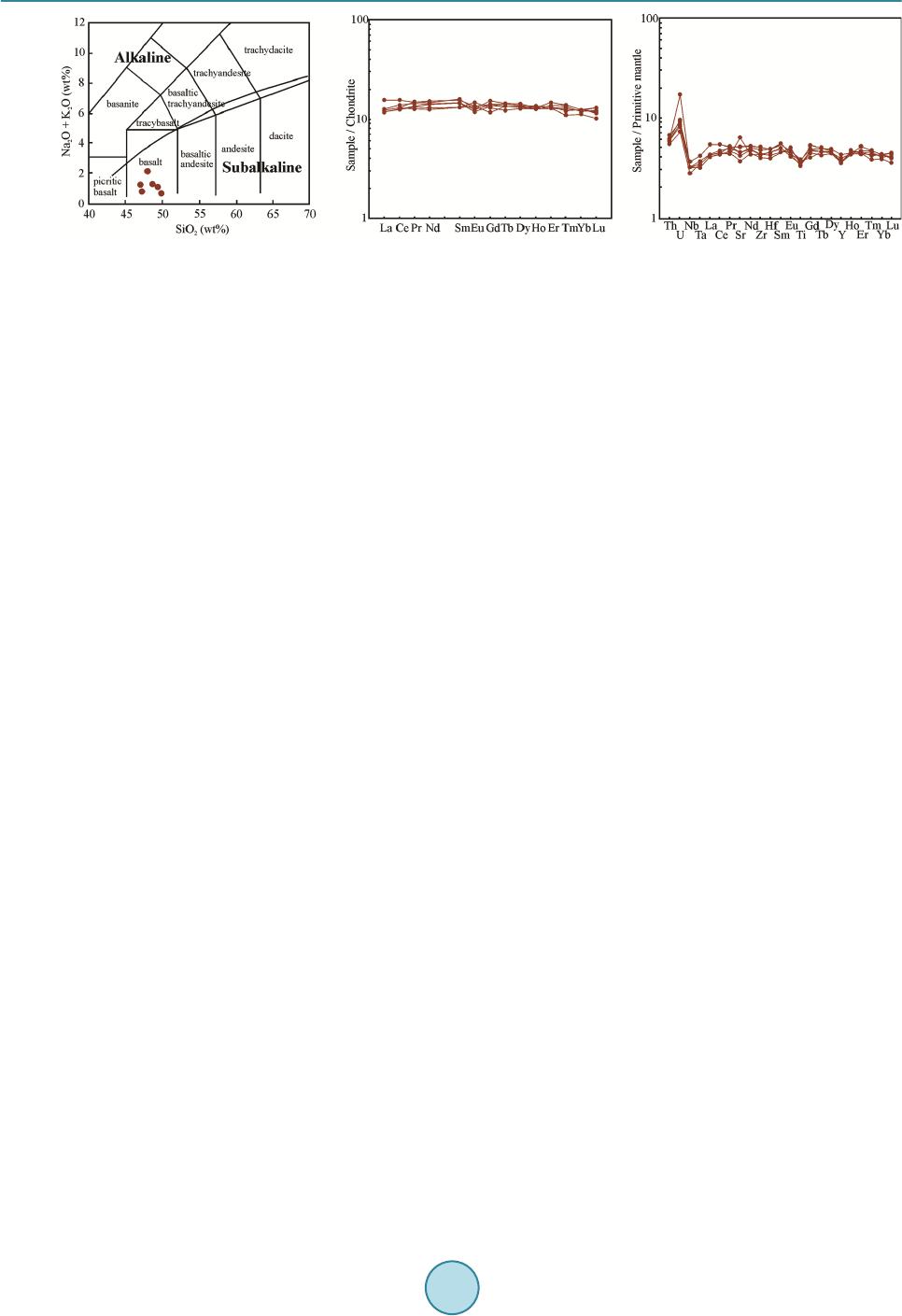 E. D. Wang et al. (a) (b) (c) Figure 3. (a) SiO2 versus K2O + Na2O diagram (after Middlemost, 1994) [14], (b) chondrite-normalized REE pattern and (c) primitive mantle normalized trace spider diagram for the Nanfen chlorite amphibolites (standard data from Sun, & McDo- nough, 1989) [15]. preserve the signature of original trace element composition (Figure 3(b) & Figure 3(c)). However, some mo- bile elements such as Rb, Ba and Sr show variable concentrations, which appear to indicate that some LILE have been significantly affected by alteration. So, we used immobile elements to discover the nature of basaltic rocks and selected appropriate discrimination diagrams for protolith reconstruction. In the Ti/100-Zr-3×Y diagram (Figure 4(a)), all of our samples plot into the overlapping area between MORB-like basalts and arc-like ones, indicating that Nanfen chlorite amphibolites not only have MORB-like compositional characteristics but also have island arc-like ones. Basaltic rocks that combine both the MORB- like characters and arc-like ones may be BABB. The FeOt/MgO-TiO2 diagram (Figure 4(b)) and Y/15-La/10 - Nb/8 diagram (Figure 4(c)) show that the protolith of Nanfen chlorite amphibolites are BABB. In addition, Nanfen samples have Nb/La = 0.69 - 0.82, Ba/La = 5.68 - 85.36, Sm/Nd = 0.33 - 0.36, Zr/Nb = 19.57 - 24.50 and Zr/Y = 2.74 - 3.29 (Table 1), similar to those observed in Okinawa Trough BABB (Nb/La > 0.6, Ba/La > 10, Sm/Nd < 0.3 and Zr/Nb ≈ 18, Zr/Y ≈ 2.9) (Zhang, Wang, Fan, Zhang, & Yang, 2012; Shinjo, 1998) [13] [16]. 6. Tectonic Significance Most Algoma-type BIFs are part of greenstone belts that have been deformed, metamorphosed, and dismem- bered. This makes reconstruction of the basinal setting of such BIFs very difficult (Bekker, Slack, Planavsky, Krapez, Hofmann, Konhauser, & Rouxel, 2010) [8]. Recovering of tectonic environment of BIF may be possible through the basaltic volcanic sequences interlayered with Algoma-type BIF. The spatial and temporal associa- tion between the BIF and basaltic volcanic sequences indicates a genetic relationship between volcanic activity and BIF. At present, the tectonic nature during Archean BIF formation period in Benxi area has been long disputed. Based on the geochemical charaters, Feng, Zhou, & Xiao (2009) and Liu, & Jin (2010) [2] [3] considered the protolith of the Gongchangling plagioclase amphibolites to be mid-ocean ridge tholeiite. Dai, Zhang, Wang, Liu, Cui, Zhu, & Xiang (2012) [1] proposed tectonic environment of its BIF as back-arc basin according to analysis results that protolith of the Waitoushan plagioclase amphibolites is BABB. Through the study on plagioclase amphibolites of middle Anshan Group, Cui, Sun, Zhang, Gu, Zhou, & Sun (2012) [4] and Zhang, Yang, Zhou, & Shi (2013) [5] considered that Gongchangling plagioclase amphibolites had typically the characters of Archean arc basalts and Waitoushan plagioclase amphibolites formed within back-arc spread- ing environment, and also presented that BIFs of Benxi area produced within the mantle plume-arc tectonic sys- tem. Based on the material source analysis about the plagioclase amphibolites combined with biotite leptynites in Gongchangling, Wang, Xia, Zhao, Fu, & Hou (2013) [7] proposed tectonic environment for Gongchangling BIF to be the back-arc basin. Sun, Zhu, Tang, Zhang, Luo, & Han (2014) [6] considered that the protolith of the Gongchangling plagioclase amphibolites to be basic volcanic rock and also proposed that Gongchangling BIF produced within subduction-related arc environment. As mentioned above, Nanfen chlorite amphibolites originated from back-arc basalts, which have the transi- tional characters from MORB to arc-like basalt because they are formed by the upwelling upper mantle beneath an ocean ridge system in a supra-subduction zone. At the initial stage of back-arc basin formation, the spreading
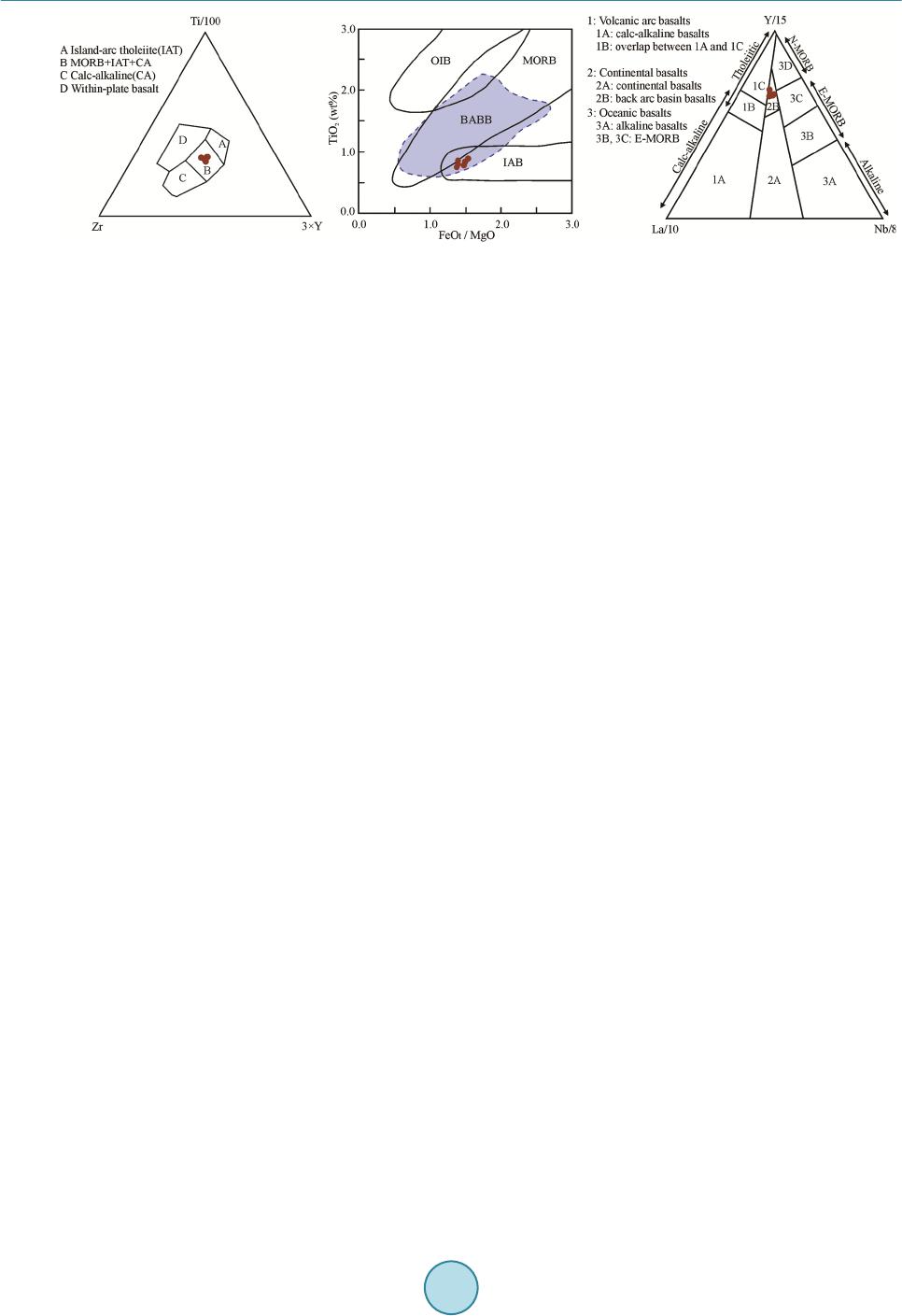 E. D. Wang et al. (a) (b) (c) Figure 4. (a) Ti/100-Zr-3×Y diagram (after Pearce, & Cann, 1973) [17], (b) FeOt/MgO-TiO2 diagram (after Shuto et al., 2006) [18] and (c) Y/15-La/1 0 -Nb/8 diagram (after Cabanis, & Lecolle, 1989) [19] for the Nanfen chlorite amphibolite. axis is located close to the island arc, and the contents of volcanic rocks are similar to the island arc ones. Dur- ing the development of the back-arc basin, the distance between the island arc and the through increase gradual- ly, and the content of volcanic rocks also become more similar to MORBs. Such a compositional feature is gen- erally acknowledged to be unique to BABB (Ghazi, Moazzen, Rahgoshay, & Moghadam, 2012; Xu, Castillo, Chen, Niu, Yu, & Zhen, 2003; Pearce, & Stern, 2006) [20]-[22]. It is generally believed that HFSE depletions were caused by the immobility of HFSE in fluids derived from the dehydration of the subducting oceanic crust in a convergent margin (Ghazi, Moazzen, Rahgoshay, & Moghadam, 2012; Xu, Castillo, Chen, Niu, Yu, & Zhen, 2003; Pearce, & Stern, 2006; Zhang, Wang, Fan, Zhang, & Yang, 2012) [13] [20]-[22]. In fact, the elemental geochemical signatures for Nanfen chlorite amphibolites, including weakly enrichment in LILE and a depletion in HFSE as well as significant negative Nb-Ta, Zr-Hf, Ti and Y anomalies (Figure 3(c)), could be explained by the introduction of metasomatized melt/fluid released from the subducted slab. Especially, the depletion and differentiation of Nb and Ta are considered to be a result of the initial partitioning and subsequent equilibration between rutile and fluids or melts in a subducted slab (Münker, 1998) [23]. The Nanfen chlo rite amphibolites have Nb/Ta ratios of 14.29 - 17.69, reflecting a mixing contribution to the matasomatized fluids and melts (Münker, 1998) [23]. In study area, the back-arc basins may have developed between the subduction zones and provided a favorable sedimentary environment for Algoma-type BIF. The hypothesis about the subduction-re- lated tectonic setting is consistent with the viewpoint of Sun, Zhu, Tang, Zhang, Luo, & Han (2014) [6] for for- mation environment of Benxi BIFs. In summary, we suggest that the Nanfen BIF were formed in a subduction-related back-arc basin. 7. Conclusions Protolith of chlorite amphibolites from Nanfen iron deposit is BABB. Tectonic environment for Nanfen BIF is the subduction-related back-arc basin. Acknowledgements We thank the financial support by Research of High-grade Iron Ore Formation Mechanism and Prediction of China (2012CB416800), Research on Archean Geologic bodies Evolution and Metallization of Large Ore De- posit in North Margin of North China Craton (41372098) and Fundamental Research Funds for the Graduate Innovation State Education Ministry (N110601002). References [1] Dai, Y.P., Zhang, L.C., Wang, C.L., Liu, L., Cui, M.L., Zhu, M.T. and Xiang, P. (201 2) Genetic Typ e, Formation Age and Tectonic Setting of Waitoushan Banded Iron Formation, Benxi, Liaoning Province. Acta Petrologica Sinica, 28, 3574-3594. [2] Fen g, J.R., Zhou, Z.H. and Xiao, R.G. (2009) The Protolith Restoration of the Second Diggings Wall Rock in Gong- changling Iron Ore, Liaoning Pr ovin ce. China Mining Magazine, 18, 106-110. [3] Li u , J. and Jin, S.Y. (2010) Geochemical Characteristics and Original Rock Restoration of Plagioclase Amphibolites in
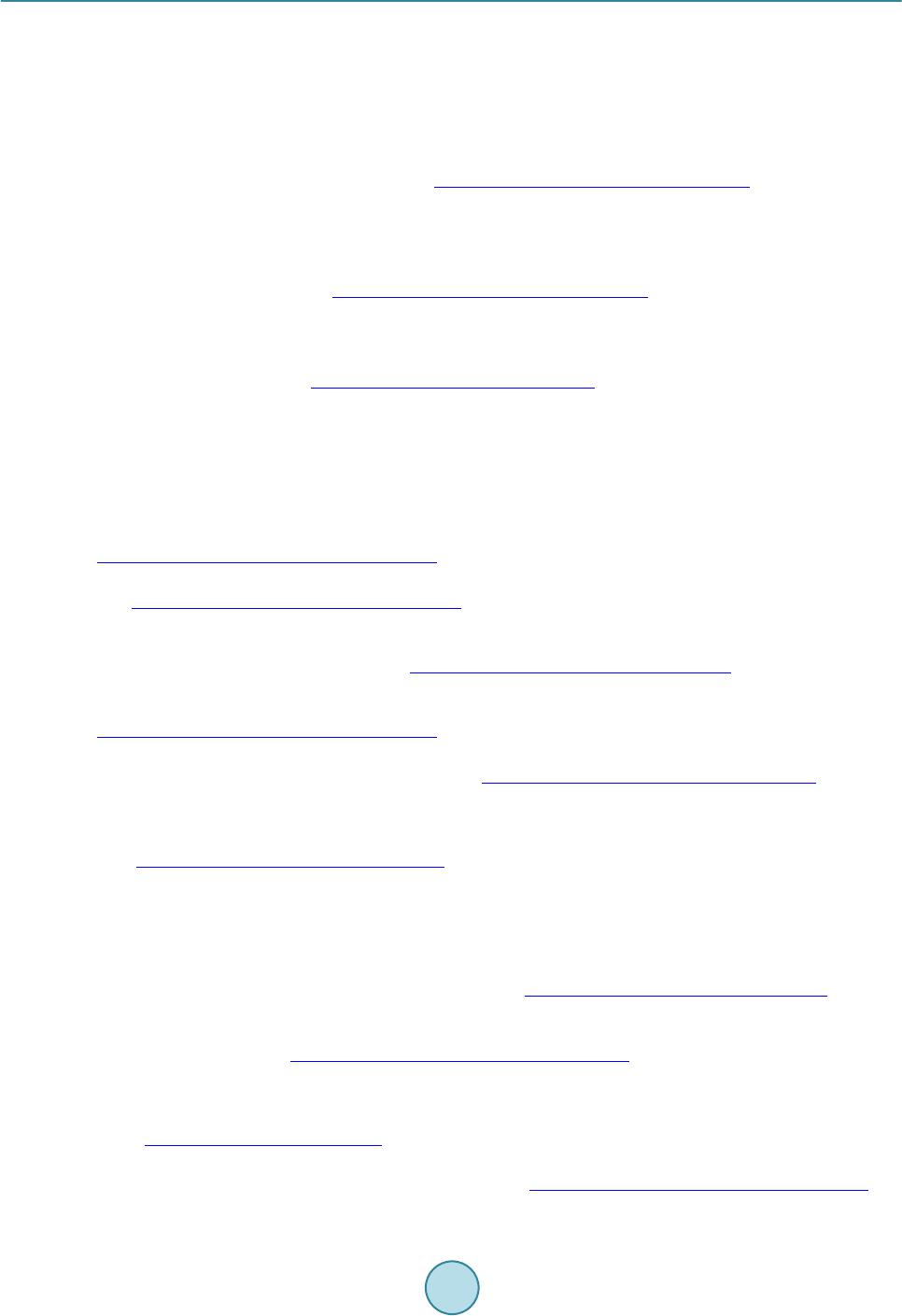 E. D. Wang et al. the Gongchangling Iron Deposit, Liaoning Province. Geology in China, 37, 324-333 . [4] Cui, P.L., Sun, J.G., Zhang, P., Gu, A.L., Zhou, K.Q. and Sun, Q.L. (2 012) Geochemical Study on BIF Wall Rock— Plagioclase Amphibolites of Cigou Formation in Anshan-Benxi Ar e a . Mineral Deposits, 31, 93-94. [5] Zhang, P., Yang, H.Z., Zhou, Y. and Shi, J.M. (20 13) Geochemical Characters and Geological Significance of Pla- gioclase Amphibolites from Anshan Group Cigou Formation, Anshan-Benxi Are a . Geological Review, 59, 1217-12 18 . [6] Sun, X.H., Zhu, X.Q., Tang, H.S., Zhang, Q., Luo, T.Y. and Han, T. (2014) Protolith Reconstruction and Geochemical Study on the Wall Rocks of Anshan BIFs, Northeast China: Implications for the Provenance and Tectonic Setting. Journal of Geochemical Exploration, 136, 65-75 . http://dx.doi.org/10.1016/j.gexplo.2013.10.009 [7] Wan g, E. D., Xia, J.M., Zhao, C.F., Fu, J.F. and Hou, G.Q. (2013) Material Sources and Sedimentary Environment of Gongchangling Iron Deposit. Mineral Deposits, 32, 380-396 . [8] Bekker, A., Slack, J.F., Planavsky, N., Krapez, B., Hofmann, A., Konhauser, K.O. and Rouxel, O.J. (2010) Iron For- mation : The Sedimentary Product of a Complex Interplay among Mantle, Tectonic, Oceanic and Biospheric Processes. Economic Geology, 105, 467-508. http://dx.doi.org/10.2113/gsecongeo.105.3.467 [9] Gr o s s , G.A. (1980) A Classification of Iron Formations Based on Depositional Environments. Canadian Mineralogist, 18, 215-222. [10] Zhai , M.G. an d Santosh, M. (2011) The Early P recambri an Odyssey of North China Craton: A Synoptic Overview. Gondwana Research, 20, 6-25. http://dx.doi.org/10.1016/j.gr.2011.02.005 [11] Zhou, S.T. (1994) The BIF Geology of Anshan—Benxi Ar e a . Geological Publishing House, Beijing. [12] Wan , Y. S., Dong, C.Y., Xie, H.Q., Wang, S.J., Song, M.C., Xu, Z.Y., Wang, S.Y., Zhou, H.Y., Ma, M.Z. and Liu, D.Y. (201 2) Formation Ages of Early Precambrian BIFs in the North China Craton: SHRIMP Zircon U-Pb Dating. Acta Geologica Sinica, 86, 1447-1478. [13] Zhang, A.M., Wang, Y.J., Fan, W.M., Zhang, Y.Z. and Yang, J. (201 2) Earliest Neoproterozoic (ca. 1.0 Ga) Arc-Back - Arc Basin Nature along the Northern Yunkai Domain of the Cathaysia Block: Geochronological and Geochemical Evidence from the Metab asite. Precambrian Research, 220-221, 217-23 3 . http://dx.doi.org/10.1016/j.precamres.2012.08.003 [14] Middlemost, E .A.K. (1994) Naming Materials in the M a g ma/Igneous Rock System. Earth Science Reviews, 37, 215- 224. http://dx.doi.org/10.1016/0012-8252(94)90029-9 [15] Sun, S.S. and McDon ou gh, W.F. (1989) Chemical and Isotopic Systematics of Oceanic Basalts: Implications for Man- tle Composition and Proces ses. In: Sau nders, A.D. and Norry, M.J., Eds., Magmatism in Ocean Basins: Special Publi- cation, Geological Society, London, 313-345. http://dx.doi.org/10.1144/gsl.sp.1989.042.01.19 [16] Shinjo, R. (1998 ) Petrochemistry and Tectonic Significance of the Emerged Late Cenozic Basalts behind the Okinawa Trough Ryukyu Arc System. Journal of Volcanology and Geothermal Research, 80, 39-53. http://dx.doi.org/10.1016/S0377-0273(97)00042-5 [17] P earce, J.A. and Cann, J.R. (1973 ) Tectonic Setting of Basic Volcanic Rocks Determined Using Trace Element Ana- lyses. Earth and Planetary Science Letters, 19, 290 -300. http://dx.doi.org/10.1016/0012-821X(73) 9012 9-5 [18] Shuto, K., Ishimoto, H., Hirahara, Y., Sato, M., Matsui, K., Fujibayanshi, N., Takazawa, E., Yabuki, K., Sekine, M., Kato, M. and Rezanov, A.I. (2006 ) Geochemical Secular Variation of Magma Source during Early to Middle Miocene Time in the Niigata Area , NE Japen: Asthenosphere Mantle Upwelling during Back-Arc Basin Opening. Lithos, 86, 1-33. http://dx.doi.org/10.1016/j.lithos.2005.06.001 [19] Caban is, B. and Lecolle, M. (19 89 ) Le diagramme La/10-Y/15-Nb/8: Un outil pour la discrimination des series volca- niques et lamise en evidence des processus demelange et/ou de contamination crustale. Compte Rendus de I’Académie des Sciences Series II, 309, 2023 -2029. [20] Ghazi, J.M., Moazzen, M., Rahgoshay, M. and Moghadam, H.S. (2012) Geochemical Characteristics of Basaltic Rocks from the Nain Ophiolite (Central Iran); Constraints on Mantle Wedge Source Evolution in an Oceanic Back-Arc Basin and a Geodynamical Model. Tectonoph ysics, 574-57 5, 92-10 4. http://dx.doi.org/10.1016/j.tecto.2011.10.001 [21] Xu, J.F., Castillo, P.R., Chen, F.R., Niu, H.C., Yu, X.Y. and Zhen, Z.P. (2003 ) Geochemistry of Late Paleozoic Mafic Igneous Rocks from the Kuerti Area, Xinjiang, Northwest China: Implications for Back-Arc Mantle Evolut ion . Chem- ical Geology, 193, 137 -154 . http://dx.doi.org/10.1016/S0009-2541(02)00265-6 [22] P earce, J.A. an d Stern, R.J. (2006) Origin of Back-Arc Basin Magmas: Trace Element and Isotope Perspectives. In: Christie, D.M., Fisher, C.R. , Lee, S.M. and Givens, S., Eds., Back-Arc Spreading Systems; Geological, Biological, Chemica l, and Physical Interactions. Geophysical Monograph Series 166, American Geophysical Union, Washington, 63-86. http://dx.doi.org/10.1029/166gm06 [23] Mün ker, C. (1998) Nb/Ta Fraction in a Cambrian Arc-Back System, New Zealand: Source Constraints and Application of Refined ICPMS Techniques. Chemical Geology, 144, 23-45. http://dx.doi.org/10.1016/S0009-2541(97 )00 105 -8
|