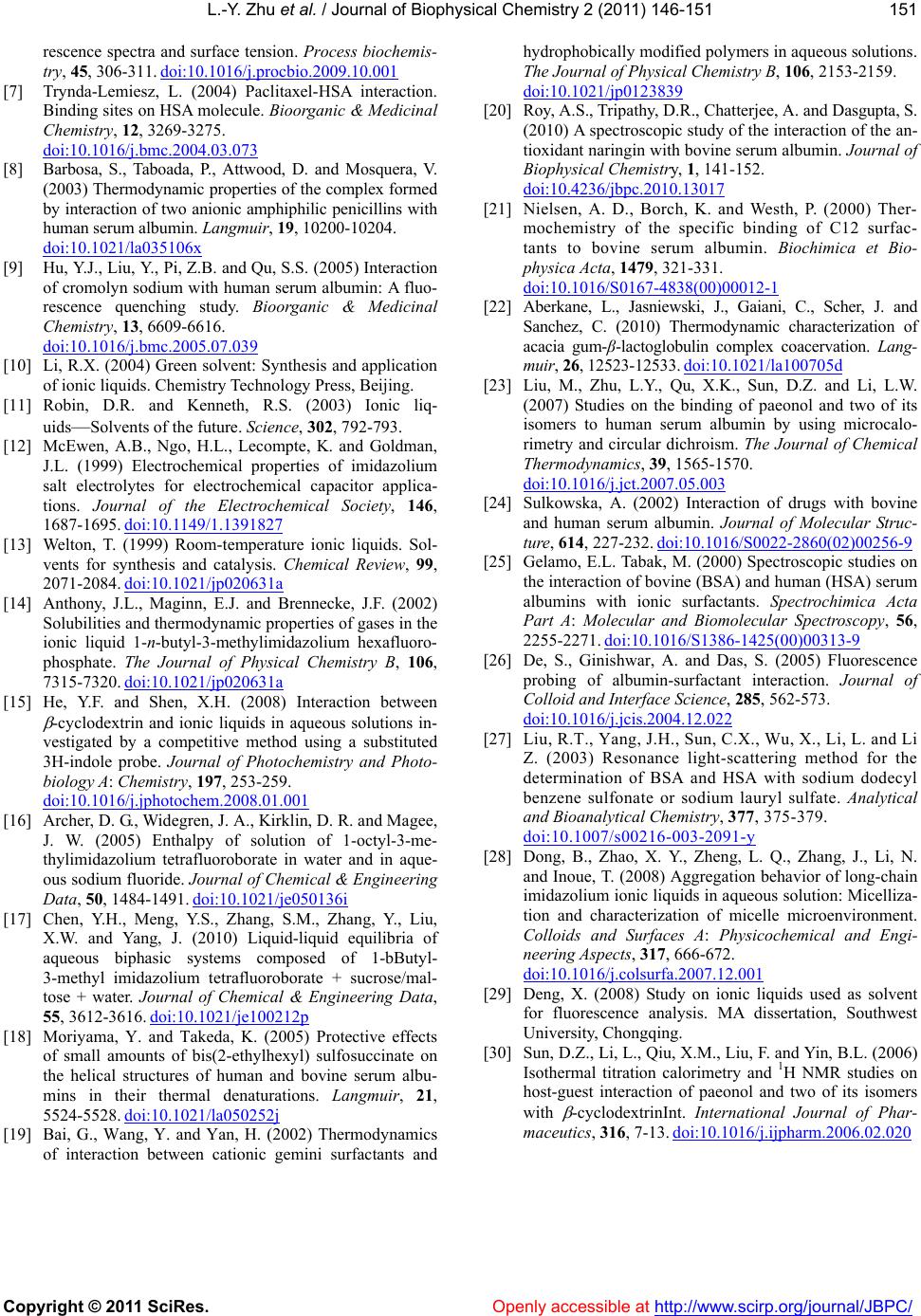
L.-Y. Zhu et al. / Journal of Biophysical Chemistry 2 (2011) 146-151
Copyright © 2011 SciRes. http://www.scirp.org/journal/JBPC/Openly accessible at
151
rescence spectra and surface tension. Process biochemis-
try, 45, 306-311. doi:10.1016/j.procbio.2009.10.001
[7] Trynda-Lemiesz, L. (2004) Paclitaxel-HSA interaction.
Binding sites on HSA molecule. Bioorganic & Medicinal
Chemistry, 12, 3269-3275.
doi:10.1016/j.bmc.2004.03.073
[8] Barbosa, S., Taboada, P., Attwood, D. and Mosquera, V.
(2003) Thermodynamic properties of the complex formed
by interaction of two anionic amphiphilic penicillins with
human serum albumin. Langmuir, 19, 10200-10204.
doi:10.1021/la035106x
[9] Hu, Y.J., Liu, Y., Pi, Z.B. and Qu, S.S. (2005) Interaction
of cromolyn sodium with human serum albumin: A fluo-
rescence quenching study. Bioorganic & Medicinal
Chemistry, 13, 6609-6616.
doi:10.1016/j.bmc.2005.07.039
[10] Li, R.X. (2004) Green solvent: Synthesis and application
of ionic liquids. Chemistry Technology Press, Beijing.
[11] Robin, D.R. and Kenneth, R.S. (2003) Ionic liq-
uids—Solvents of the future. Science, 302, 792-793.
[12] McEwen, A.B., Ngo, H.L., Lecompte, K. and Goldman,
J.L. (1999) Electrochemical properties of imidazolium
salt electrolytes for electrochemical capacitor applica-
tions. Journal of the Electrochemical Society, 146,
1687-1695. doi:10.1149/1.1391827
[13] Welton, T. (1999) Room-temperature ionic liquids. Sol-
vents for synthesis and catalysis. Chemical Review, 99,
2071-2084. doi:10.1021/jp020631a
[14] Anthony, J.L., Maginn, E.J. and Brennecke, J.F. (2002)
Solubilities and thermodynamic properties of gases in the
ionic liquid 1-n-butyl-3-methylimidazolium hexafluoro-
phosphate. The Journal of Physical Chemistry B, 106,
7315-7320. doi:10.1021/jp020631a
[15] He, Y.F. and Shen, X.H. (2008) Interaction between
-cyclodextrin and ionic liquids in aqueous solutions in-
vestigated by a competitive method using a substituted
3H-indole probe. Journal of Photochemistry and Photo-
biology A: Chemistry, 197, 253-259.
doi:10.1016/j.jphotochem.2008.01.001
[16] Archer, D. G., Widegren, J. A., Kirklin, D. R. and Magee,
J. W. (2005) Enthalpy of solution of 1-octyl-3-me-
thylimidazolium tetrafluoroborate in water and in aque-
ous sodium fluoride. Journal of Chemical & Engineering
Data, 50, 1484-1491. doi:10.1021/je050136i
[17] Chen, Y.H., Meng, Y.S., Zhang, S.M., Zhang, Y., Liu,
X.W. and Yang, J. (2010) Liquid-liquid equilibria of
aqueous biphasic systems composed of 1-bButyl-
3-methyl imidazolium tetrafluoroborate + sucrose/mal-
tose + water. Journal of Chemical & Engineering Data,
55, 3612-3616. doi:10.1021/je100212p
[18] Moriyama, Y. and Takeda, K. (2005) Protective effects
of small amounts of bis(2-ethylhexyl) sulfosuccinate on
the helical structures of human and bovine serum albu-
mins in their thermal denaturations. Langmuir, 21,
5524-5528. doi:10.1021/la050252j
[19] Bai, G., Wang, Y. and Yan, H. (2002) Thermodynamics
of interaction between cationic gemini surfactants and
hydrophobically modified polymers in aqueous solutions.
The Journal of Physical Chemistry B, 106, 2153-2159.
doi:10.1021/jp0123839
[20] Roy, A.S., Tripathy, D.R., Chatterjee, A. and Dasgupta, S.
(2010) A spectroscopic study of the interaction of the an-
tioxidant naringin with bovine serum albumin. Journal of
Biophysical Chemistry, 1, 141-152.
doi:10.4236/jbpc.2010.13017
[21] Nielsen, A. D., Borch, K. and Westh, P. (2000) Ther-
mochemistry of the specific binding of C12 surfac-
tants to bovine serum albumin. Biochimica et Bio-
physica Acta, 1479, 321-331.
doi:10.1016/S0167-4838(00)00012-1
[22] Aberkane, L., Jasniewski, J., Gaiani, C., Scher, J. and
Sanchez, C. (2010) Thermodynamic characterization of
acacia gum-β-lactoglobulin complex coacervation. Lang-
muir, 26, 12523-12533. doi:10.1021/la100705d
[23] Liu, M., Zhu, L.Y., Qu, X.K., Sun, D.Z. and Li, L.W.
(2007) Studies on the binding of paeonol and two of its
isomers to human serum albumin by using microcalo-
rimetry and circular dichroism. The Journal of Chemical
Thermodynamics, 39, 1565-1570.
doi:10.1016/j.jct.2007.05.003
[24] Sulkowska, A. (2002) Interaction of drugs with bovine
and human serum albumin. Journal of Molecular Struc-
ture, 614, 227-232. doi:10.1016/S0022-2860(02)00256-9
[25] Gelamo, E.L. Tabak, M. (2000) Spectroscopic studies on
the interaction of bovine (BSA) and human (HSA) serum
albumins with ionic surfactants. Spectrochimica Acta
Part A: Molecular and Biomolecular Spectroscopy, 56,
2255-2271. doi:10.1016/S1386-1425(00)00313-9
[26] De, S., Ginishwar, A. and Das, S. (2005) Fluorescence
probing of albumin-surfactant interaction. Journal of
Colloid and Interface Science, 285, 562-573.
doi:10.1016/j.jcis.2004.12.022
[27] Liu, R.T., Yang, J.H., Sun, C.X., Wu, X., Li, L. and Li
Z. (2003) Resonance light-scattering method for the
determination of BSA and HSA with sodium dodecyl
benzene sulfonate or sodium lauryl sulfate. Analytical
and Bioanalytical Chemistry, 377, 375-379.
doi:10.1007/s00216-003-2091-y
[28] Dong, B., Zhao, X. Y., Zheng, L. Q., Zhang, J., Li, N.
and Inoue, T. (2008) Aggregation behavior of long-chain
imidazolium ionic liquids in aqueous solution: Micelliza-
tion and characterization of micelle microenvironment.
Colloids and Surfaces A: Physicochemical and Engi-
neering Aspects, 317, 666-672.
doi:10.1016/j.colsurfa.2007.12.001
[29] Deng, X. (2008) Study on ionic liquids used as solvent
for fluorescence analysis. MA dissertation, Southwest
University, Chongqing.
[30] Sun, D.Z., Li, L., Qiu, X.M., Liu, F. and Yin, B.L. (2006)
Isothermal titration calorimetry and 1H NMR studies on
host-guest interaction of paeonol and two of its isomers
with
-cyclodextrinInt. International Journal of Phar-
maceutics, 316, 7-13. doi:10.1016/j.ijpharm.2006.02.020