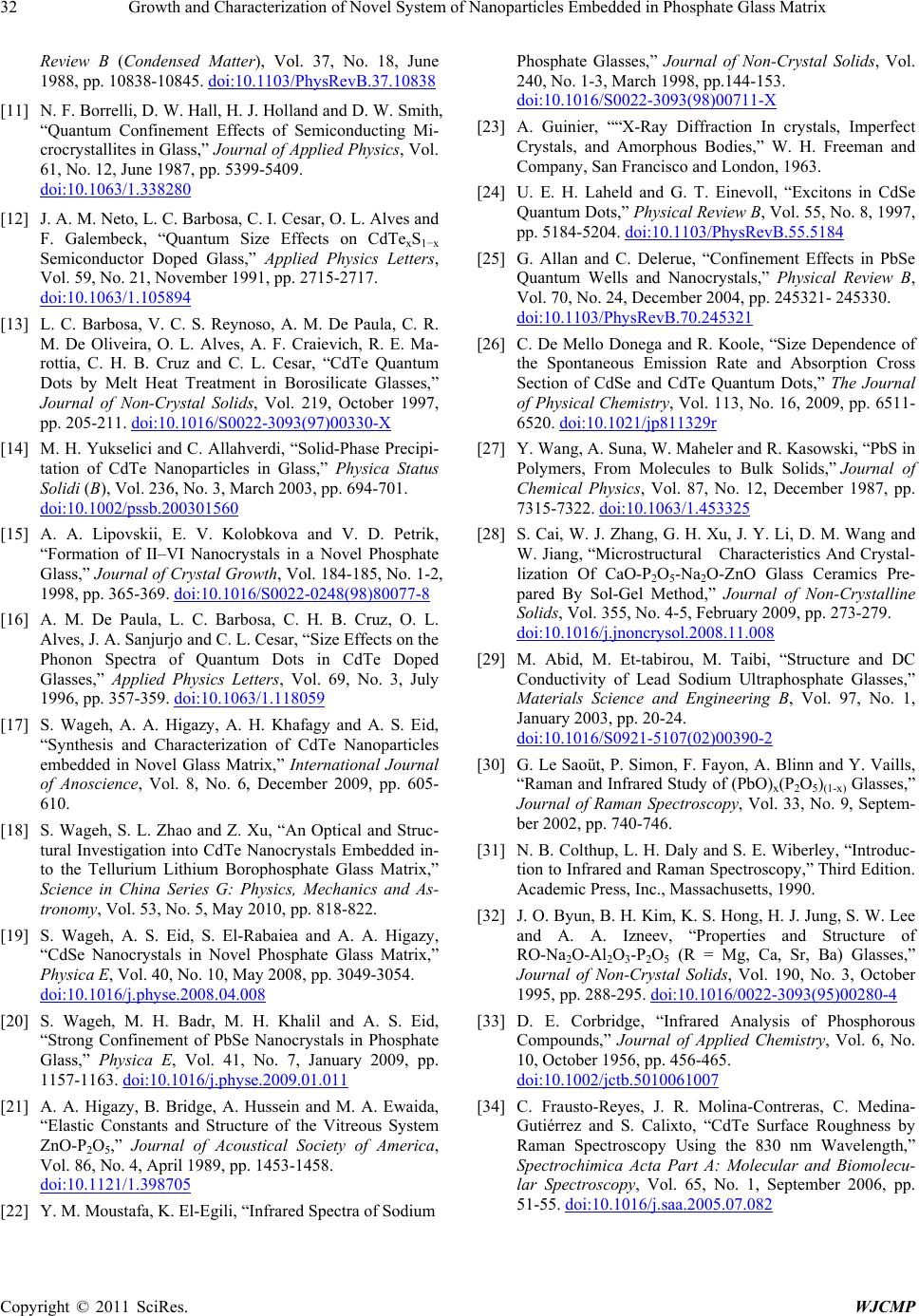
32 Growth and Characterization of Novel System of Nanoparticles Embedded in Phosphate Glass Matrix
Copyright © 2011 SciRes. WJCMP
Review B (Condensed Matter), Vol. 37, No. 18, June
1988, pp. 10838-10845. doi:10.1103/PhysRevB.37.10838
[11] N. F. Borrelli, D. W. Hall, H. J. Holland and D. W. Smith,
“Quantum Confinement Effects of Semiconducting Mi-
crocrystallites in Glass,” Journal of Applied Physics, Vol.
61, No. 12, June 1987, pp. 5399-5409.
doi:10.1063/1.338280
[12] J. A. M. N eto, L. C. Barbosa, C. I. Cesar, O. L. Alves and
F. Galembeck, “Quantum Size Effects on CdTexS1−x
Semiconductor Doped Glass,” Applied Physics Letters,
Vol. 59, No. 21, November 1991, pp. 2715-2717.
doi:10.1063/1.105894
[13] L. C. Barbosa, V. C. S. Reynoso, A. M. De Paula, C. R.
M. De Oliveira, O. L. Alves, A. F. Craievich, R. E. Ma-
rottia, C. H. B. Cruz and C. L. Cesar, “CdTe Quantum
Dots by Melt Heat Treatment in Borosilicate Glasses,”
Journal of Non-Crystal Solids, Vol. 219, October 1997,
pp. 205-211. doi:10.1016/S0022-3093(97)00330-X
[14] M. H. Yukselici and C. Allahverdi, “Solid-Phase Precipi-
tation of CdTe Nanoparticles in Glass,” Physica Status
Solidi (B), Vol. 236, No. 3, March 2003, pp. 694-701.
doi:10.1002/pssb.200301560
[15] A. A. Lipovskii, E. V. Kolobkova and V. D. Petrik,
“Formation of II–VI Nanocrystals in a Novel Phosphate
Glass,” Journal of Crystal Growth, Vol. 184-185, No. 1-2,
1998, pp. 365-369. doi:10.1016/S0022-0248(98)80077-8
[16] A. M. De Paula, L. C. Barbosa, C. H. B. Cruz, O. L.
Alves, J. A. Sanjurjo and C. L. Cesar, “Size Effects on the
Phonon Spectra of Quantum Dots in CdTe Doped
Glasses,” Applied Physics Lette rs, Vol. 69, No. 3, July
1996, pp. 357-359. doi:10.1063/1.118059
[17] S. Wageh, A. A. Higazy, A. H. Khafagy and A. S. Eid,
“Synthesis and Characterization of CdTe Nanoparticles
embedded in Novel Glass Matrix,” International Journal
of Anoscience, Vol. 8, No. 6, December 2009, pp. 605-
610.
[18] S. Wageh, S. L. Zhao and Z. Xu, “An Optical and Struc-
tural Investigation into CdTe Nanocrystals Embedded in-
to the Tellurium Lithium Borophosphate Glass Matrix,”
Science in China Series G: Physics, Mechanics and As-
tronomy, Vol. 53, No. 5, May 2010, pp. 818-822.
[19] S. Wageh, A. S. Eid, S. El-Rabaiea and A. A. Higazy,
“CdSe Nanocrystals in Novel Phosphate Glass Matrix,”
Physica E, Vol . 40, No. 10, Ma y 2008, pp. 3049-3054.
doi:10.1016/j.physe.2008.04.008
[20] S. Wageh, M. H. Badr, M. H. Khalil and A. S. Eid,
“Strong Confinement of PbSe Nanocrystals in Phosphate
Glass,” Physica E, Vol. 41, No. 7, January 2009, pp.
1157-1163. doi:10.1016/j.physe.2009.01.011
[21] A. A. Higazy, B. Bridge, A. Hussein and M. A. Ewaida,
“Elastic Constants and Structure of the Vitreous System
ZnO-P2O5,” Journal of Acoustical Society of America,
Vol. 86, No. 4, April 1989, pp. 1453-1458.
doi:10.1121/1.398705
[22] Y. M. Moustafa, K. El-Egili, “Infrared Spectra of Sodium
Phosphate Glasses,” Journal of Non-Crystal Solids, Vol.
240, No. 1-3, March 1998, pp.144-153.
doi:10.1016/S0022-3093(98)00711-X
[23] A. Guinier, ““X-Ray Diffraction In crystals, Imperfect
Crystals, and Amorphous Bodies,” W. H. Freeman and
Company, San Francisco and London, 1963.
[24] U. E. H. Laheld and G. T. Einevoll, “Excitons in CdSe
Quantum Dots,” Physical Review B, Vol. 55, No. 8, 1997,
pp. 5184-5204. doi:10.1103/PhysRevB.55.5184
[25] G. Allan and C. Delerue, “Confinement Effects in PbSe
Quantum Wells and Nanocrystals,” Physical Revie w B,
Vol. 70, No. 24, December 2004, pp. 245321- 245330.
doi:10.1103/PhysRevB.70.245321
[26] C. De Mello Donega and R. Koole, “Size Dependence of
the Spontaneous Emission Rate and Absorption Cross
Section of CdSe and CdTe Quantum Dots,” The Journal
of Physical Chemist ry, Vol. 113, No. 16, 2009, pp. 6511-
6520. doi:10.1021/jp811329r
[27] Y. Wang, A. Suna, W. Maheler and R. Kasowski, “PbS in
Polymers, From Molecules to Bulk Solids,” Journal of
Chemical Physics, Vol. 87, No. 12, December 1987, pp.
7315-7322. doi:10.1063/1.453325
[28] S. Cai, W. J. Zhang, G. H. Xu, J. Y. Li, D. M. Wang and
W. Jiang, “Microstructural Characteristics And Crystal-
lization Of CaO-P2O5-Na2O-ZnO Glass Ceramics Pre-
pared By Sol-Gel Method,” Journal of Non-Crystalline
Solids, Vol. 355, No. 4-5, February 2009, pp. 273-279.
doi:10.1016/j.jnoncrysol.2008.11.008
[29] M. Abid, M. Et-tabirou, M. Taibi, “Structure and DC
Conductivity of Lead Sodium Ultraphosphate Glasses,”
Materials Science and Engineering B, Vol. 97, No. 1,
January 2003, pp. 20-24.
doi:10.1016/S0921-5107(02)00390-2
[30] G. Le Saoüt, P. Simon, F. Fayon, A. Blinn and Y. Vaills,
“Raman and Infrared Study of (PbO)x(P2O5)(1-x) Glasses,”
Journal of Raman Spectroscopy, Vol. 33, No. 9, Septem-
ber 2002, pp. 740-746.
[31] N. B. Colthup, L. H. Daly and S. E. Wiber ley, “Introduc-
tion to Infrared and Raman Spectroscopy,” Third Edition.
Academic Press, Inc., Massachusetts, 1990.
[32] J. O. Byun, B. H. Kim, K. S. Hong, H. J. Jung, S. W. Lee
and A. A. Izneev, “Properties and Structure of
RO-Na2O-Al2O3-P2O5 (R = Mg, Ca, Sr, Ba) Glasses,”
Journal of Non-Crystal Solids, Vol. 190, No. 3, October
1995, pp. 288-295. doi:10.1016/0022-3093(95)00280-4
[33] D. E. Corbridge, “Infrared Analysis of Phosphorous
Compounds,” Journal of Applied Chemistry, Vol. 6, No.
10, October 1956, pp. 456-465.
doi:10.1002/jctb.5010061007
[34] C. Frausto-Reyes, J. R. Molina-Contreras, C. Medina-
Gutiérrez and S. Calixto, “CdTe Surface Roughness by
Raman Spectroscopy Using the 830 nm Wavelength,”
Spectrochimica Acta Part A: Molecular and Biomolecu-
lar Spectroscopy, Vol. 65, No. 1, September 2006, pp.
51-55. doi:10.1016/j.saa.2005.07.082