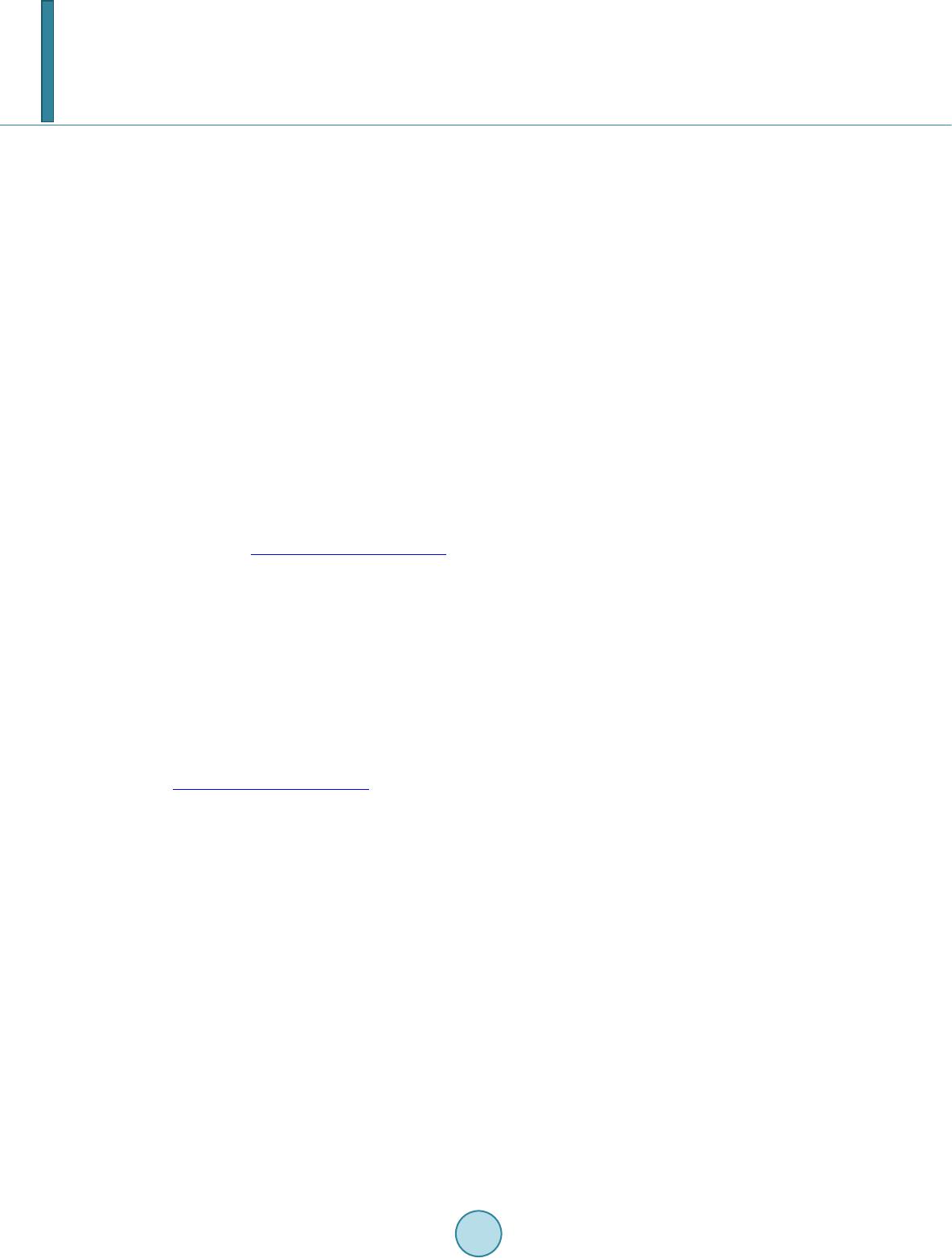
J. Gong et al.
As shown in Figure 9, in the range 3 - 5 mm downstream from throat, peak values of downstream pressure
gradients are reached for all the nozzles with different maximum expansion angles. The absolute value of peak
pressure gradient decreases with maximum expansion angles, indicating that the change of wall pressure be-
comes mitigated. Because it is convex wall for nozzle flow near the throat, the low favorable pressure drives
flow moving downstream steady rather than rapid expansion which would lead to oscillation in flow and nozzle
wall.
4. Conclusion
It can be concluded that a lower maximum expansion angle is required for quiet nozzle. However, a too low
value is also unfeasible because it may lead to extremely long nozzle consisting of more sections. Specifically, if
the maximum expansion angle is set as 3˚, a 4 m long expansion section which must be divided into more than
10 sections would be required (the 4˚ quiet nozzle at Purdue University is 2.56 m long and consists of 8 sec-
tions). Difficulties would be encountered in machining to meet the requirement of low roughness and smooth
joint. in another words, maximum expansion angle should be neither too large nor too small, and a optimized
value is to be determined. According to the discussion in the previous part, a optimized range of maximum ex-
pansion angle is 4˚ - 6˚. The fact that the maximum expansion angle of the Mach 6 quiet nozzle (the longest
nozzle all over the world) designed by Schneider is 4˚, agrees with our analysis.
References
[1] Beckwith, I.E. (1975) Development of a High Reynolds Number Quiet Tunnel for Transition Research. AIAA Journal,
13, 300-306. http://dx.doi.org/10.2514/3.49695
[2] Anders, J.B., Stainback, P.C. and Beckwith, I.E. (1978) A New Technique for Reducing Test Section Noise in Super-
sonic Wind Tunnels. AIAA Paper: 1978-0817.
[3] Beckwith, I.E. and Moore, W.O. (1982) Mean Flow and Noise Measurements in a Mach 3.5 Pilot Quiet Tunnel. AIAA
Paper: 1982-0569.
[4] Ch en, F.J., Wilkinson, S.P. and Beckwith, I.E. (1991) Görtler Instability and Hypersonic Quiet Nozzle Design. AIAA
Paper: 1991-1648.
[5] Schneider, S.P. (1998) Design and Fabrication of a 9.5-inch Mach-6 Quiet-Flow Ludwieg Tube. AIAA Paper:
1998-2511.
[6] Zhou, Y.W., Chang, X.Y., Yi, S.H. and Zhang, Y. (2002) The Aerodynamic Design of Supersonic Quiet Wind Tunnel.
Experiments and Measurements in Fluid Mechanics, 16, 61-66.
[7] Schneider, S.P. (2008) Development of Hypersonic Quiet Tunnels. Journal of Spacecraft and Rockets, 45, 641-664.
http://dx.doi.org/10.2514/1.34489
[8] Zhou, Y.W. and Yi, S.H. (2010) Summarization of the Characteristics and Development of Hypersonic Quiet Wind
Tunnel. Journal of Experimental Mechanics, 25, 167-172.
[9] Beckwith, I.E. and Holley, B.B. (1981) Görtler Vortices and Transition in Wall Boundary Layers of Two Mach-5 Noz-
zles. NASA -TP-1869.
[10] Schneider, S.P. (1998) Design of a Mach-6 Quiet-Flow Wind-Tunnel Nozzle Using the eN Method for Transition Es-
timation. AIAA Paper: 1998-0547.