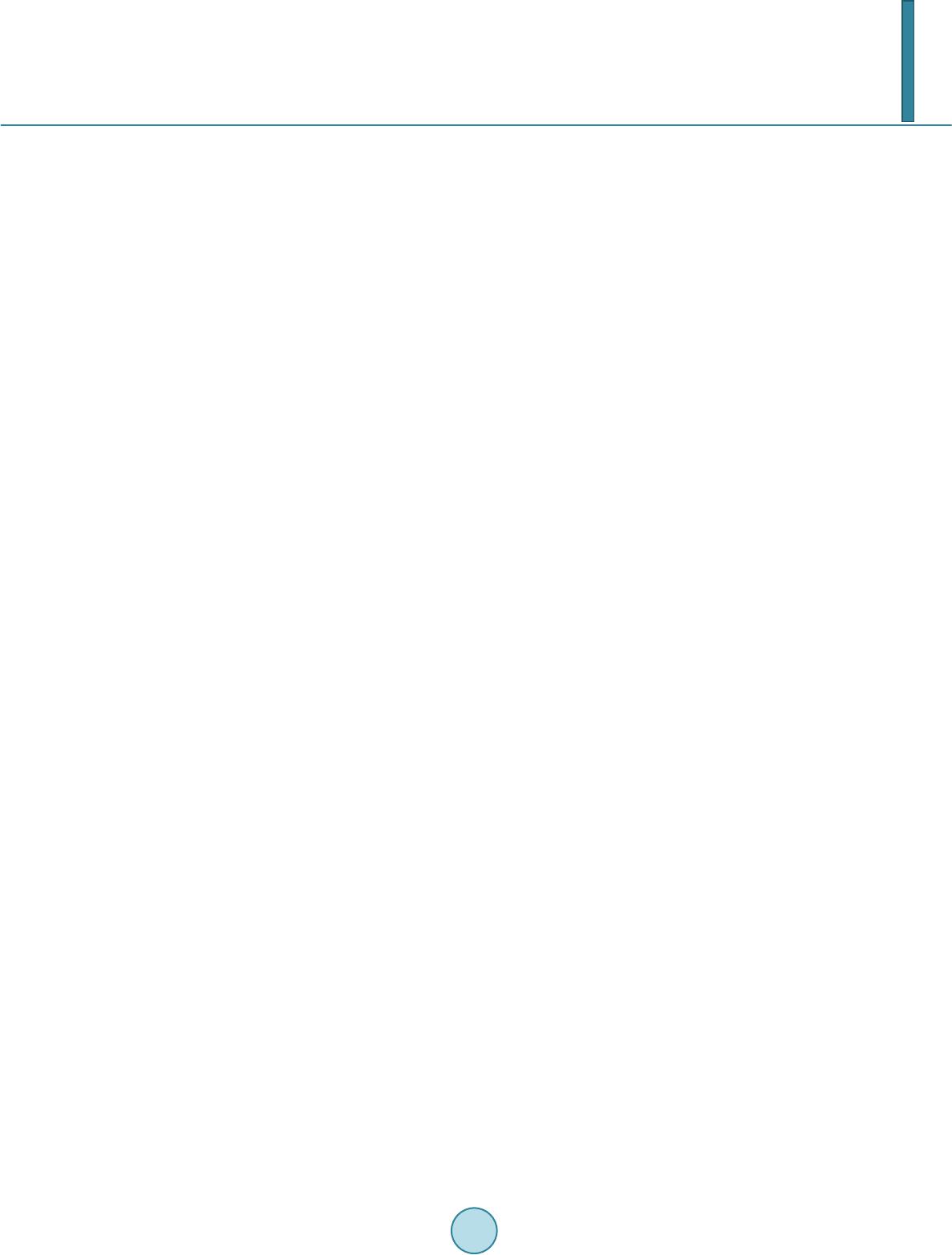
W. Thipprasert, P. Sritakaew
sorbing surge energy, the phenomenon of thermal runaway occurs as a result of increased heat generation caused
by subsequent ac voltage application. SA must not only be thermally stable at normal operating stress, but must
also discharge various transient overvoltage energies and return to the former thermally stable condition from
the temporary high temperature state. The latter is termed transient thermal stability. In the following text, tran-
sient thermal stability is abbreviated to thermal stability. When the operating stress becomes higher, the ab-
sorbed energy for transient overvoltages becomes larger because the current created by transient overvoltages is
larger. Therefore, the discharge capability requirement and the transient thermal stability are more severe in the
case of high operating stress [1-3].
ZnO SAs are fundamental elements of protecting systems of electric devices against overvoltages. They con-
tain varistores, which have ZnO as the main constituent as well as small amounts of other metal oxides. SAs are
exposed to transient effect of overvoltages generated as a result of lightning discharges, switching and damage
states in electric power systems.
This thermal runaway phenomenon can be studied as a problem of balance between heat generation and heat
dissipation. Conventionally, this problem has been discussed simply as static phenomenon on the basis that heat
generation of ZnO elements was uniform along the ZnO columns, while their heat dissipation was equally uni-
form [1,3]. Actual measurements were also conducted on single or several series-connected ZnO elements that
possessed different heat dissipating conditions.
The electrical energy is then converted and stored in the metal oxide valve elements as thermal energy, thus
resulting in a temperature rise above normal operating levels. An increase of valve element temperature can up-
set the thermal balance in the arrester assembly, resulting in a thermal runaway. Since, the thermal capabilities
of gapless metal oxide SAa depend on ambient temperature and solar radiation gain, both factors must be consi-
dered in application studies. Such studies can now be carried out using a relatively simple and yet representative
model of the thermal properties at the arrester valve element and housing [1].
In this research, in order to understand the thermal and electrical properties of a ZnO SAs 50 Hz AC voltage
changes in leakage current were measured. The temperature distribution appearing on the ZnO SA was observed
using a forward looking infrared camera. In particular, the correlation between the thermal and electrical proper-
ties of a ZnO SA was analyzed experimentally. From this analysis, the thermal phenomena resulting from the
heat generation and dissipation of the ZnO SA wer e interpr eted.
2. Thermal Performance of ZnO Surge Arrester [1]
The thermal stability of ZnO SA is affected by ambient temperature and heat dissipation capability, impulse de-
gradation and ageing. To obtain thermal stability, the electrical power dissipation in the element must be ba-
lanced against heat output to the environment. Near the thermal equilibrium, it is possible to express the thermal
dissipation capacity Q of a SA as:
(1)
where T is the temperature of ZnO valve elements, Ta is the ambient temperature and CT the thermal dissipation
factor. The heat generation, P, which is voltage and material composition dependent, may be approximated by
(2)
where Wc is the activation energy, k = 0.86 × 10 eV/K (Boltzmann constant), T is the temperature of the material
and A depends on the applied voltage level and the physical dimensions of the valve elements. The above curves
are shown schematically in Figures 1 and 2. For an ambient temperature Ta and an applied voltage V, the two
curves intersect at two points X and Y. The lower point X is at a stable operating temperature Tx and is referred
to as the lower limit stability point. The upper point Y is also at a stable operating temperature Ty and is called
the upper limit stability point. At these temperatures, the power input equals the power output.
3. Thermal Runaway Phenomenon of ZnO Surge Arrester [1,3]
When a ZnO SA is connected to a distribution system, resistive leakage current is less than 1 mA flows through
the arrester, as shown in Figure 3. However, when large surge energy of power system is absorbed, the temper-
ature of ZnO elements increases, causing the resistance value to decrease. As a result, the leakage current be-
comes greater and the heat generation increases. Even if the heat generation increases, the temperature rise by
surge energy absorption T1 shown in Figure 4 may be below the certain temperature limit intrinsic to the SA.