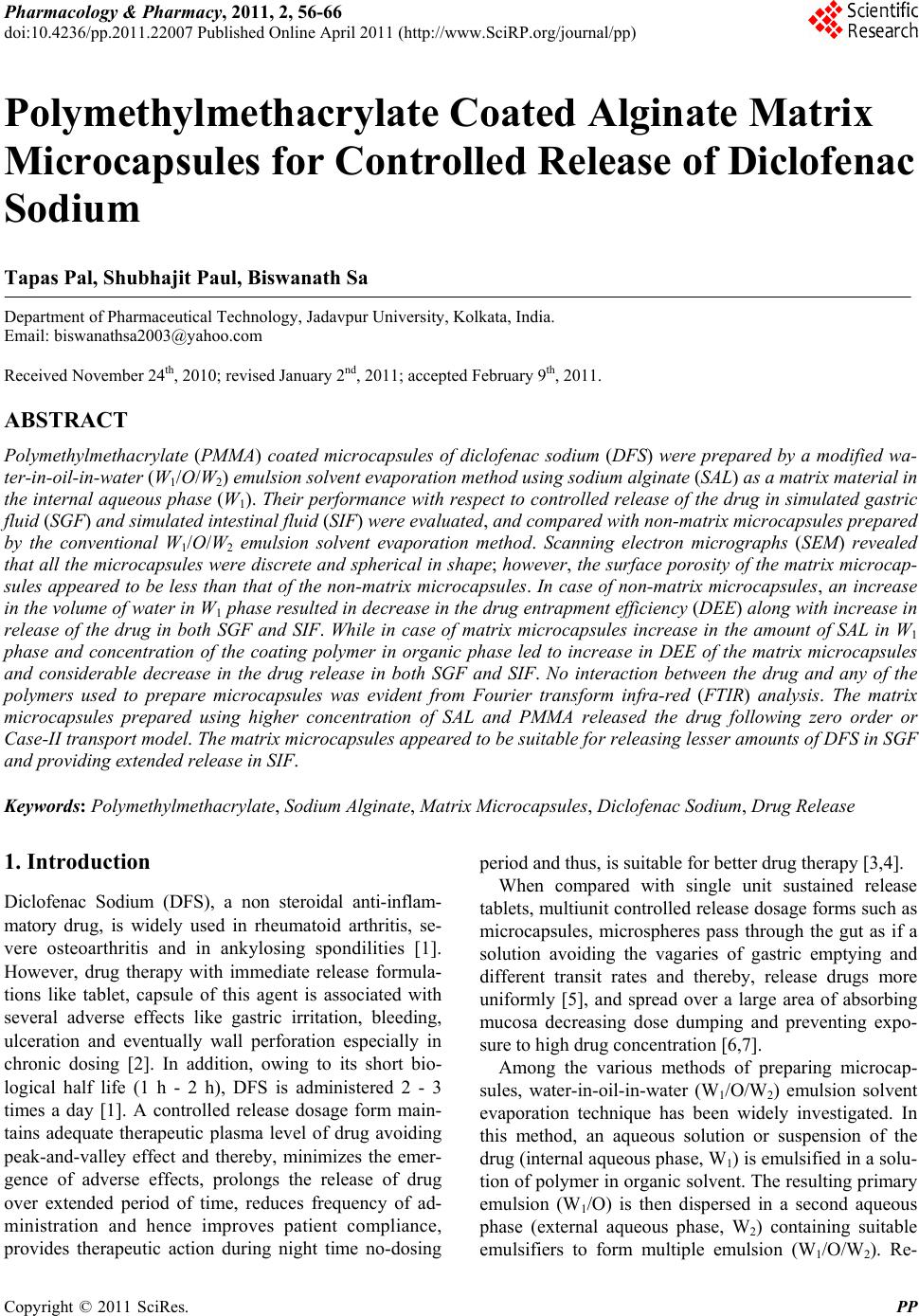 Pharmacology & Pharmacy, 2011, 2, 56-66 doi:10.4236/pp.2011.22007 Published Online April 2011 (http://www.SciRP.org/journal/pp) Copyright © 2011 SciRes. PP Polymethylmethacrylate Coated Alginate Matrix Microcapsules for Controlled Release of Diclofenac Sodium Tapas Pal, Shubhajit Paul, Biswanath Sa Department of Pharmaceutical Technology, Jadavpur University, Kolkata, India. Email: biswanathsa2003@yahoo.com Received November 24th, 2010; revised January 2nd, 2011; accepted February 9th, 2011. ABSTRACT Polymethylmethacrylate (PMMA) coated microcapsules of diclofenac sodium (DFS) were prepared by a modified wa- ter-in-oil-in-water (W1/O/W2) emulsion solvent evapora tion method u sing so d ium alginate (SAL) as a matrix material in the interna l aqueous phase (W1). Their performance with respect to controlled release of the drug in simulated gastric fluid (SGF) and simula ted in testin al flu id (SIF ) were eva lua ted, and compared with non-matrix microcapsules prepared by the conventional W1/O/W2 emulsion solvent evaporation method. Scanning electron micrographs (SEM) revealed that all the microcapsules were discrete and spherical in shape; however, the surface porosity of the matrix microcap- sules appeared to be less than that of the non-matrix microcapsules. In case of no n-matrix microcapsules, an increase in the volume of water in W1 phas e resulted in decrease in the drug entrapment efficien cy (DEE) along with increa se in release of the drug in both SGF and SIF. While in case of matrix microcapsules increase in the amount of SAL in W1 phase and concentration of the coating polymer in organic phase led to increase in DEE of the matrix microcapsules and considerable decrease in the drug release in both SGF and SIF. No interaction between the drug and any of the polymers used to prepare microcapsules was evident from Fourier transform infra-red (FTIR) analysis. The matrix microcapsules prepared using higher concentration of SAL and PMMA released the drug following zero order or Case-II transport model. The matrix microcapsules appeared to be suitable for releasing lesser amounts of DFS in SGF and providi ng ext ende d release in SIF. Keywords: Polymethylmethacrylate, Sodiu m Al gi n at e , Matrix Microcapsules, Diclofenac Sodium, Drug Release 1. Introduction Diclofenac Sodium (DFS), a non steroidal anti-inflam- matory drug, is widely used in rheumatoid arthritis, se- vere osteoarthritis and in ankylosing spondilities [1]. However, drug therapy with immediate release formula- tions like tablet, capsule of this agent is associated with several adverse effects like gastric irritation, bleeding, ulceration and eventually wall perforation especially in chronic dosing [2]. In addition, owing to its short bio- logical half life (1 h - 2 h), DFS is administered 2 - 3 times a day [1]. A controlled release dosage form main- tains adequate therapeutic plasma level of drug avoiding peak-and-valley effect and thereby, minimizes the emer- gence of adverse effects, prolongs the release of drug over extended period of time, reduces frequency of ad- ministration and hence improves patient compliance, provides therapeutic action during night time no-dosing period and thus, is suitable for better drug therapy [3,4]. When compared with single unit sustained release tablets, multiunit controlled release dosage forms such as microcapsules, microspheres pass through the gut as if a solution avoiding the vagaries of gastric emptying and different transit rates and thereby, release drugs more uniformly [5], and spread over a large area of absorbing mucosa decreasing dose dumping and preventing expo- sure to high drug concentration [6,7]. Among the various methods of preparing microcap- sules, water-in-oil-in-water (W1/O/W2) emulsion solvent evaporation technique has been widely investigated. In this method, an aqueous solution or suspension of the drug (internal aqueous phase, W1) is emulsified in a solu- tion of polymer in organic solvent. The resulting primary emulsion (W1/O) is then dispersed in a second aqueous phase (external aqueous phase, W2) containing suitable emulsifiers to form multiple emulsion (W1/O/W2). Re-
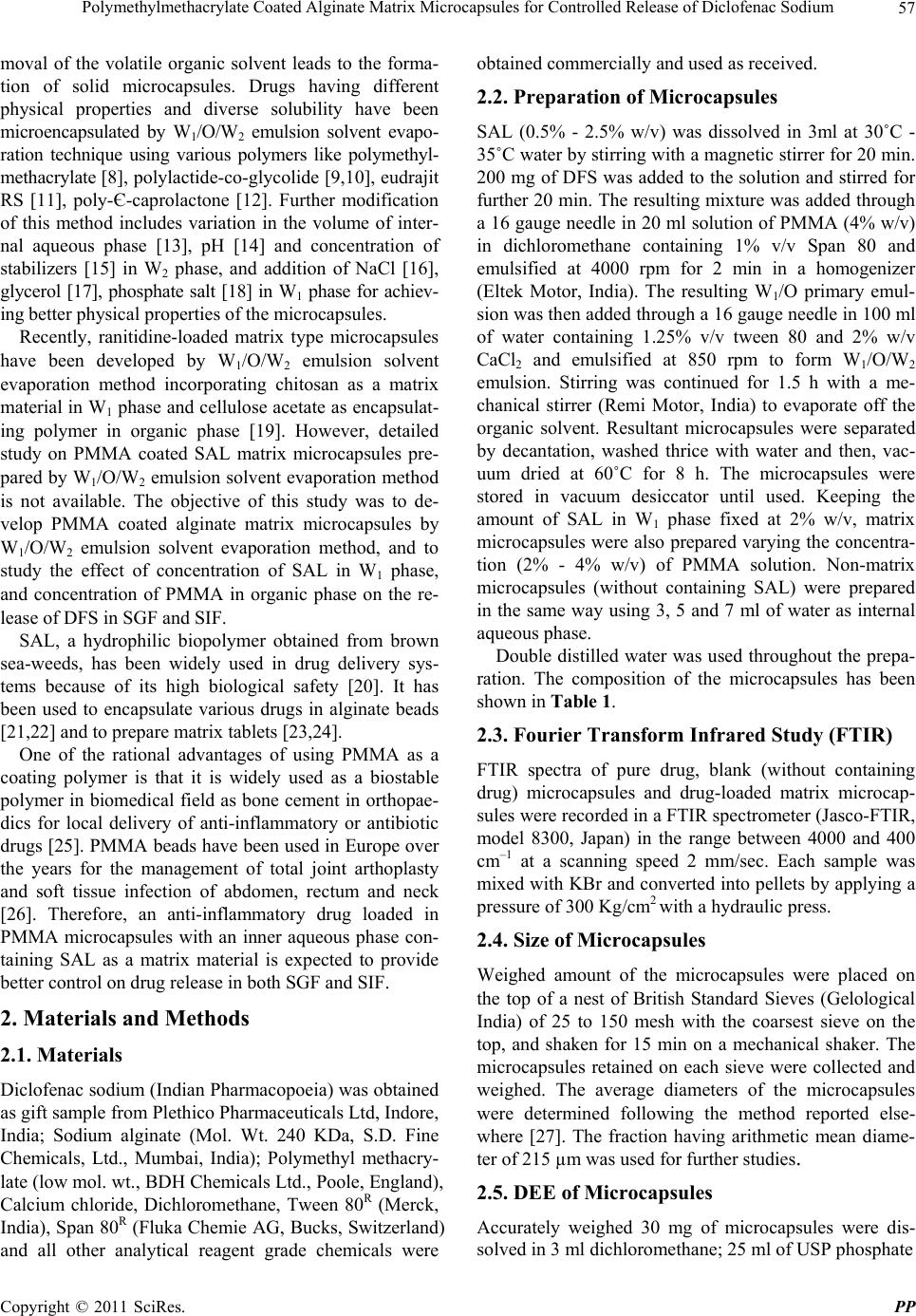 Polymethylmethacrylate Coated Alginate Matrix Microcapsules for Controlled Release of Diclofenac Sodium57 moval of the volatile organic solvent leads to the forma- tion of solid microcapsules. Drugs having different physical properties and diverse solubility have been microencapsulated by W1/O/W2 emulsion solvent evapo- ration technique using various polymers like polymethyl- methacrylate [8], polylactide-co-glycolide [9,10], eudrajit RS [11], poly-Є-caprolactone [12]. Further modification of this method includes variation in the volume of inter- nal aqueous phase [13], pH [14] and concentration of stabilizers [15] in W2 phase, and addition of NaCl [16], glycerol [17], phosphate salt [18] in W1 phase for achiev- ing better physical properties of the microcapsules. Recently, ranitidine-loaded matrix type microcapsules have been developed by W1/O/W2 emulsion solvent evaporation method incorporating chitosan as a matrix material in W1 phase and cellulose acetate as encapsulat- ing polymer in organic phase [19]. However, detailed study on PMMA coated SAL matrix microcapsules pre- pared by W1/O/W2 emulsion solvent evaporation method is not available. The objective of this study was to de- velop PMMA coated alginate matrix microcapsules by W1/O/W2 emulsion solvent evaporation method, and to study the effect of concentration of SAL in W1 phase, and concentration of PMMA in organic phase on the re- lease of DFS in SGF and SIF. SAL, a hydrophilic biopolymer obtained from brown sea-weeds, has been widely used in drug delivery sys- tems because of its high biological safety [20]. It has been used to encapsulate various drugs in alginate beads [21,22] and to prepare matrix tablets [23,24]. One of the rational advantages of using PMMA as a coating polymer is that it is widely used as a biostable polymer in biomedical field as bone cement in orthopae- dics for local delivery of anti-inflammatory or antibiotic drugs [25]. PMMA beads have been used in Europe over the years for the management of total joint arthoplasty and soft tissue infection of abdomen, rectum and neck [26]. Therefore, an anti-inflammatory drug loaded in PMMA microcapsules with an inner aqueous phase con- taining SAL as a matrix material is expected to provide better control on drug release in both SGF and SIF. 2. Materials and Methods 2.1. Materials Diclofenac sodium (Indian Pharmacopoeia) was obtained as gift sample from Plethico Pharmaceuticals Ltd, Indore, India; Sodium alginate (Mol. Wt. 240 KDa, S.D. Fine Chemicals, Ltd., Mumbai, India); Polymethyl methacry- late (low mol. wt., BDH Chemicals Ltd., Poole, England), Calcium chloride, Dichloromethane, Tween 80R (Merck, India), Span 80R (Fluka Chemie AG, Bucks, Switzerland) and all other analytical reagent grade chemicals were obtained commercially and used as received. 2.2. Preparation of Microcapsules SAL (0.5% - 2.5% w/v) was dissolved in 3ml at 30˚C - 35˚C water by stirring with a magnetic stirrer for 20 min. 200 mg of DFS was added to the solution and stirred for further 20 min. The resulting mixture was added through a 16 gauge needle in 20 ml solution of PMMA (4% w/v) in dichloromethane containing 1% v/v Span 80 and emulsified at 4000 rpm for 2 min in a homogenizer (Eltek Motor, India). The resulting W1/O primary emul- sion was then added through a 16 gauge needle in 100 ml of water containing 1.25% v/v tween 80 and 2% w/v CaCl2 and emulsified at 850 rpm to form W1/O/W2 emulsion. Stirring was continued for 1.5 h with a me- chanical stirrer (Remi Motor, India) to evaporate off the organic solvent. Resultant microcapsules were separated by decantation, washed thrice with water and then, vac- uum dried at 60˚C for 8 h. The microcapsules were stored in vacuum desiccator until used. Keeping the amount of SAL in W1 phase fixed at 2% w/v, matrix microcapsules were also prepared varying the concentra- tion (2% - 4% w/v) of PMMA solution. Non-matrix microcapsules (without containing SAL) were prepared in the same way using 3, 5 and 7 ml of water as internal aqueous phase. Double distilled water was used throughout the prepa- ration. The composition of the microcapsules has been shown in Table 1. 2.3. Fourier Transform Infrared Study (FTIR) FTIR spectra of pure drug, blank (without containing drug) microcapsules and drug-loaded matrix microcap- sules were recorded in a FTIR spectrometer (Jasco-FTIR, model 8300, Japan) in the range between 4000 and 400 cm–1 at a scanning speed 2 mm/sec. Each sample was mixed with KBr and converted into pellets by applying a pressure of 300 Kg/cm2 with a hydraulic press. 2.4. Size of Microcapsules Weighed amount of the microcapsules were placed on the top of a nest of British Standard Sieves (Gelological India) of 25 to 150 mesh with the coarsest sieve on the top, and shaken for 15 min on a mechanical shaker. The microcapsules retained on each sieve were collected and weighed. The average diameters of the microcapsules were determined following the method reported else- where [27]. The fraction having arithmetic mean diame- ter of 215 µm was used for further studies. 2.5. DEE of Microcapsules Accurately weighed 30 mg of microcapsules were dis- solved in 3 ml dichloromethane; 25 ml of USP phosphate Copyright © 2011 SciRes. PP
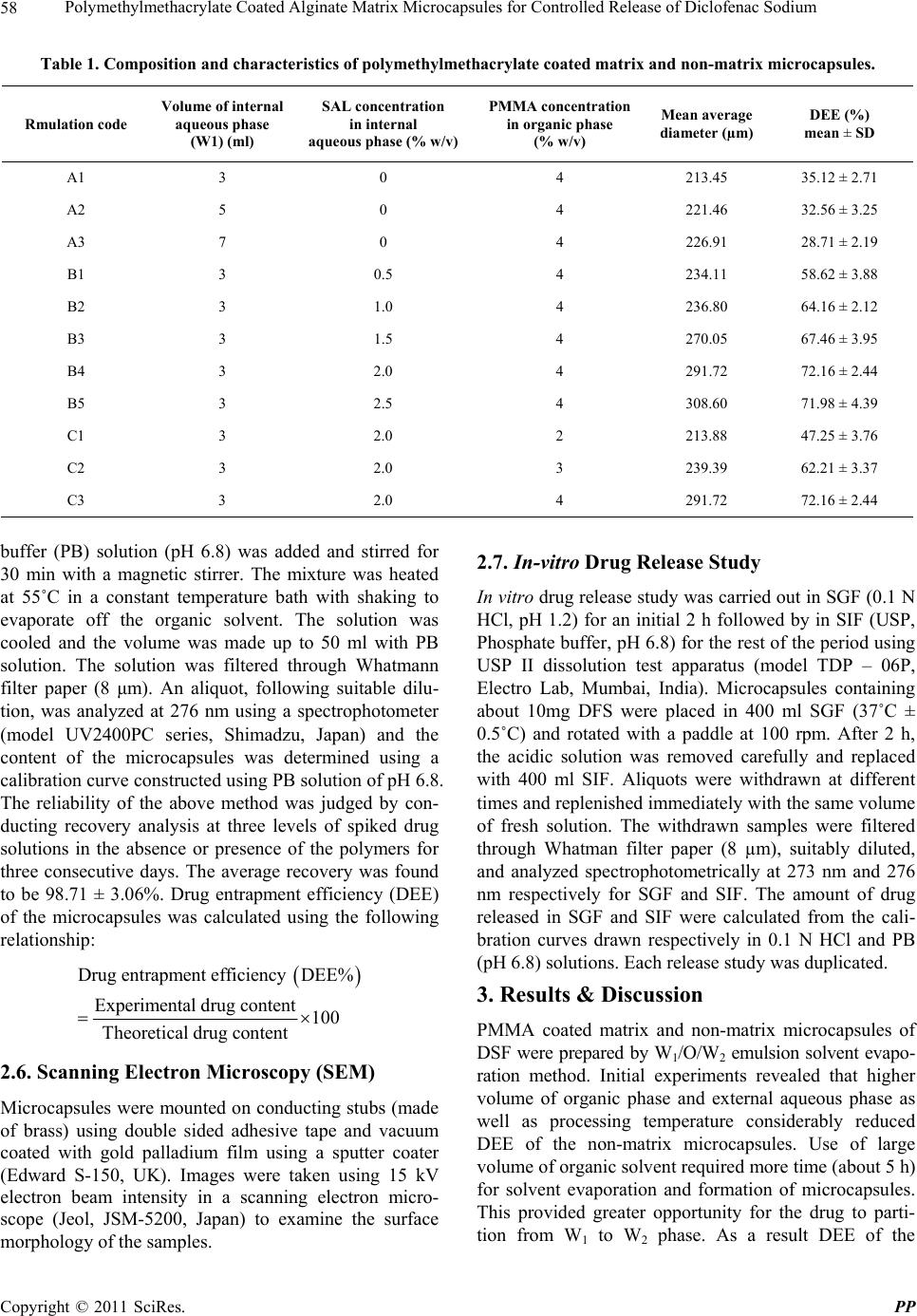 Polymethylmethacrylate Coated Alginate Matrix Microcapsules for Controlled Release of Diclofenac Sodium 58 Table 1. Composition and characteristics of polymethylmethacrylate coated matrix and non-matrix microcapsules. Rmulation code Volume of internal aqueous phase (W1) (ml) SAL concentration in internal aqueous phase (% w/v) PMMA concentration in organic phase (% w/v) Mean average diameter (µm) DEE (%) mean ± SD A1 3 0 4 213.45 35.12 ± 2.71 A2 5 0 4 221.46 32.56 ± 3.25 A3 7 0 4 226.91 28.71 ± 2.19 B1 3 0.5 4 234.11 58.62 ± 3.88 B2 3 1.0 4 236.80 64.16 ± 2.12 B3 3 1.5 4 270.05 67.46 ± 3.95 B4 3 2.0 4 291.72 72.16 ± 2.44 B5 3 2.5 4 308.60 71.98 ± 4.39 C1 3 2.0 2 213.88 47.25 ± 3.76 C2 3 2.0 3 239.39 62.21 ± 3.37 C3 3 2.0 4 291.72 72.16 ± 2.44 buffer (PB) solution (pH 6.8) was added and stirred for 30 min with a magnetic stirrer. The mixture was heated at 55˚C in a constant temperature bath with shaking to evaporate off the organic solvent. The solution was cooled and the volume was made up to 50 ml with PB solution. The solution was filtered through Whatmann filter paper (8 μm). An aliquot, following suitable dilu- tion, was analyzed at 276 nm using a spectrophotometer (model UV2400PC series, Shimadzu, Japan) and the content of the microcapsules was determined using a calibration curve constructed using PB solution of pH 6.8. The reliability of the above method was judged by con- ducting recovery analysis at three levels of spiked drug solutions in the absence or presence of the polymers for three consecutive days. The average recovery was found to be 98.71 ± 3.06%. Drug entrapment efficiency (DEE) of the microcapsules was calculated using the following relationship: Drug entrapment efficiency DEE% Experimental drug content100 Theoretical drug content 2.6. Scanning Electron Microscopy (SEM) Microcapsules were mounted on conducting stubs (made of brass) using double sided adhesive tape and vacuum coated with gold palladium film using a sputter coater (Edward S-150, UK). Images were taken using 15 kV electron beam intensity in a scanning electron micro- scope (Jeol, JSM-5200, Japan) to examine the surface morphology of the samples. 2.7. In-vitro Drug Release Study In vitro drug release study was carried out in SGF (0.1 N HCl, pH 1.2) for an initial 2 h followed by in SIF (USP, Phosphate buffer, pH 6.8) for the rest of the period using USP II dissolution test apparatus (model TDP – 06P, Electro Lab, Mumbai, India). Microcapsules containing about 10mg DFS were placed in 400 ml SGF (37˚C ± 0.5˚C) and rotated with a paddle at 100 rpm. After 2 h, the acidic solution was removed carefully and replaced with 400 ml SIF. Aliquots were withdrawn at different times and replenished immediately with the same volume of fresh solution. The withdrawn samples were filtered through Whatman filter paper (8 µm), suitably diluted, and analyzed spectrophotometrically at 273 nm and 276 nm respectively for SGF and SIF. The amount of drug released in SGF and SIF were calculated from the cali- bration curves drawn respectively in 0.1 N HCl and PB (pH 6.8) solutions. Each release study was duplicated. 3. Results & Discussion PMMA coated matrix and non-matrix microcapsules of DSF were prepared by W1/O/W2 emulsion solvent evapo- ration method. Initial experiments revealed that higher volume of organic phase and external aqueous phase as well as processing temperature considerably reduced DEE of the non-matrix microcapsules. Use of large volume of organic solvent required more time (about 5 h) for solvent evaporation and formation of microcapsules. This provided greater opportunity for the drug to parti- tion from W1 to W2 phase. As a result DEE of the Copyright © 2011 SciRes. PP
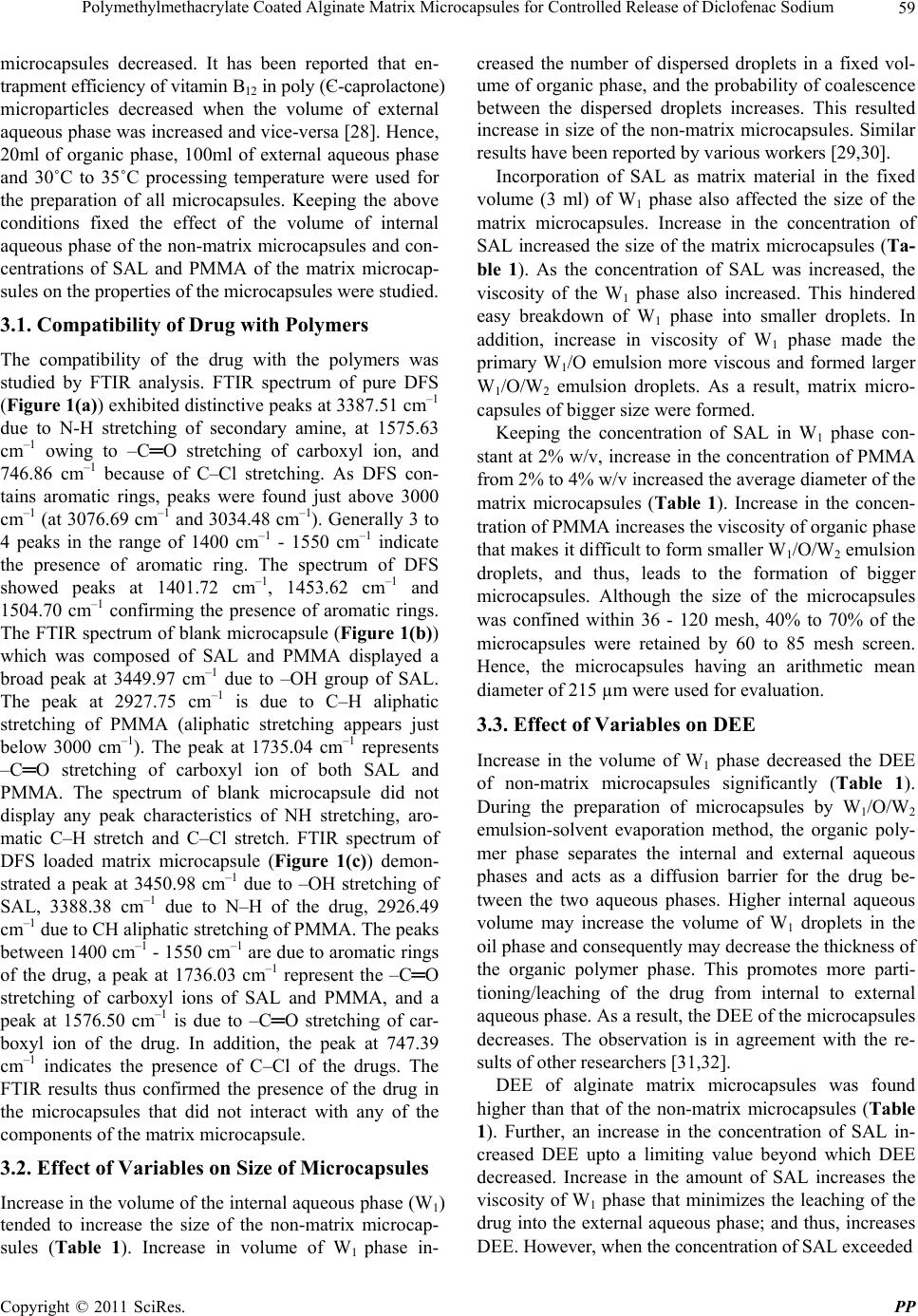 Polymethylmethacrylate Coated Alginate Matrix Microcapsules for Controlled Release of Diclofenac Sodium59 microcapsules decreased. It has been reported that en- trapment efficiency of vitamin B12 in poly (Є-caprolactone) microparticles decreased when the volume of external aqueous phase was increased and vice-versa [28]. Hence, 20ml of organic phase, 100ml of external aqueous phase and 30˚C to 35˚C processing temperature were used for the preparation of all microcapsules. Keeping the above conditions fixed the effect of the volume of internal aqueous phase of the non-matrix microcapsules and con- centrations of SAL and PMMA of the matrix microcap- sules on the properties of the microcapsules were studied. 3.1. Compatibility of Drug with Polymers The compatibility of the drug with the polymers was studied by FTIR analysis. FTIR spectrum of pure DFS (Figure 1(a)) exhibited distinctive peaks at 3387.51 cm–1 due to N-H stretching of secondary amine, at 1575.63 cm–1 owing to –C═O stretching of carboxyl ion, and 746.86 cm–1 because of C–Cl stretching. As DFS con- tains aromatic rings, peaks were found just above 3000 cm–1 (at 3076.69 cm–1 and 3034.48 cm–1). Generally 3 to 4 peaks in the range of 1400 cm–1 - 1550 cm–1 indicate the presence of aromatic ring. The spectrum of DFS showed peaks at 1401.72 cm–1, 1453.62 cm–1 and 1504.70 cm–1 confirming the presence of aromatic rings. The FTIR spectrum of blank microcapsule (Figure 1(b)) which was composed of SAL and PMMA displayed a broad peak at 3449.97 cm–1 due to –OH group of SAL. The peak at 2927.75 cm–1 is due to C–H aliphatic stretching of PMMA (aliphatic stretching appears just below 3000 cm–1). The peak at 1735.04 cm–1 represents –C═O stretching of carboxyl ion of both SAL and PMMA. The spectrum of blank microcapsule did not display any peak characteristics of NH stretching, aro- matic C–H stretch and C–Cl stretch. FTIR spectrum of DFS loaded matrix microcapsule (Figure 1(c)) demon- strated a peak at 3450.98 cm–1 due to –OH stretching of SAL, 3388.38 cm–1 due to N–H of the drug, 2926.49 cm–1 due to CH aliphatic stretching of PMMA. The peaks between 1400 cm–1 - 1550 cm–1 are due to aromatic rings of the drug, a peak at 1736.03 cm–1 represent the –C═O stretching of carboxyl ions of SAL and PMMA, and a peak at 1576.50 cm–1 is due to –C═O stretching of car- boxyl ion of the drug. In addition, the peak at 747.39 cm–1 indicates the presence of C–Cl of the drugs. The FTIR results thus confirmed the presence of the drug in the microcapsules that did not interact with any of the components of the matrix microcapsule. 3.2. Effect of Variables on Size of Microcapsules Increase in the volume of the internal aqueous phase (W1) tended to increase the size of the non-matrix microcap- sules (Table 1). Increase in volume of W1 phase in- creased the number of dispersed droplets in a fixed vol- ume of organic phase, and the probability of coalescence between the dispersed droplets increases. This resulted increase in size of the non-matrix microcapsules. Similar results have been reported by various workers [29,30]. Incorporation of SAL as matrix material in the fixed volume (3 ml) of W1 phase also affected the size of the matrix microcapsules. Increase in the concentration of SAL increased the size of the matrix microcapsules (Ta- ble 1). As the concentration of SAL was increased, the viscosity of the W1 phase also increased. This hindered easy breakdown of W1 phase into smaller droplets. In addition, increase in viscosity of W1 phase made the primary W1/O emulsion more viscous and formed larger W1/O/W2 emulsion droplets. As a result, matrix micro- capsules of bigger size were formed. Keeping the concentration of SAL in W1 phase con- stant at 2% w/v, increase in the concentration of PMMA from 2% to 4% w/v increased the average diameter of the matrix microcapsules (Table 1). Increase in the concen- tration of PMMA increases the viscosity of organic phase that makes it difficult to form smaller W1/O/W2 emulsion droplets, and thus, leads to the formation of bigger microcapsules. Although the size of the microcapsules was confined within 36 - 120 mesh, 40% to 70% of the microcapsules were retained by 60 to 85 mesh screen. Hence, the microcapsules having an arithmetic mean diameter of 215 µm were used for evaluation. 3.3. Effect of Variables on DEE Increase in the volume of W1 phase decreased the DEE of non-matrix microcapsules significantly (Table 1). During the preparation of microcapsules by W1/O/W2 emulsion-solvent evaporation method, the organic poly- mer phase separates the internal and external aqueous phases and acts as a diffusion barrier for the drug be- tween the two aqueous phases. Higher internal aqueous volume may increase the volume of W1 droplets in the oil phase and consequently may decrease the thickness of the organic polymer phase. This promotes more parti- tioning/leaching of the drug from internal to external aqueous phase. As a result, the DEE of the microcapsules decreases. The observation is in agreement with the re- sults of other researchers [31,32]. DEE of alginate matrix microcapsules was found higher than that of the non-matrix microcapsules (Table 1). Further, an increase in the concentration of SAL in- creased DEE upto a limiting value beyond which DEE decreased. Increase in the amount of SAL increases the viscosity of W1 phase that minimizes the leaching of the drug into the external aqueous phase; and thus, increases DEE. However, when the concentration of SAL exceeded Copyright © 2011 SciRes. PP
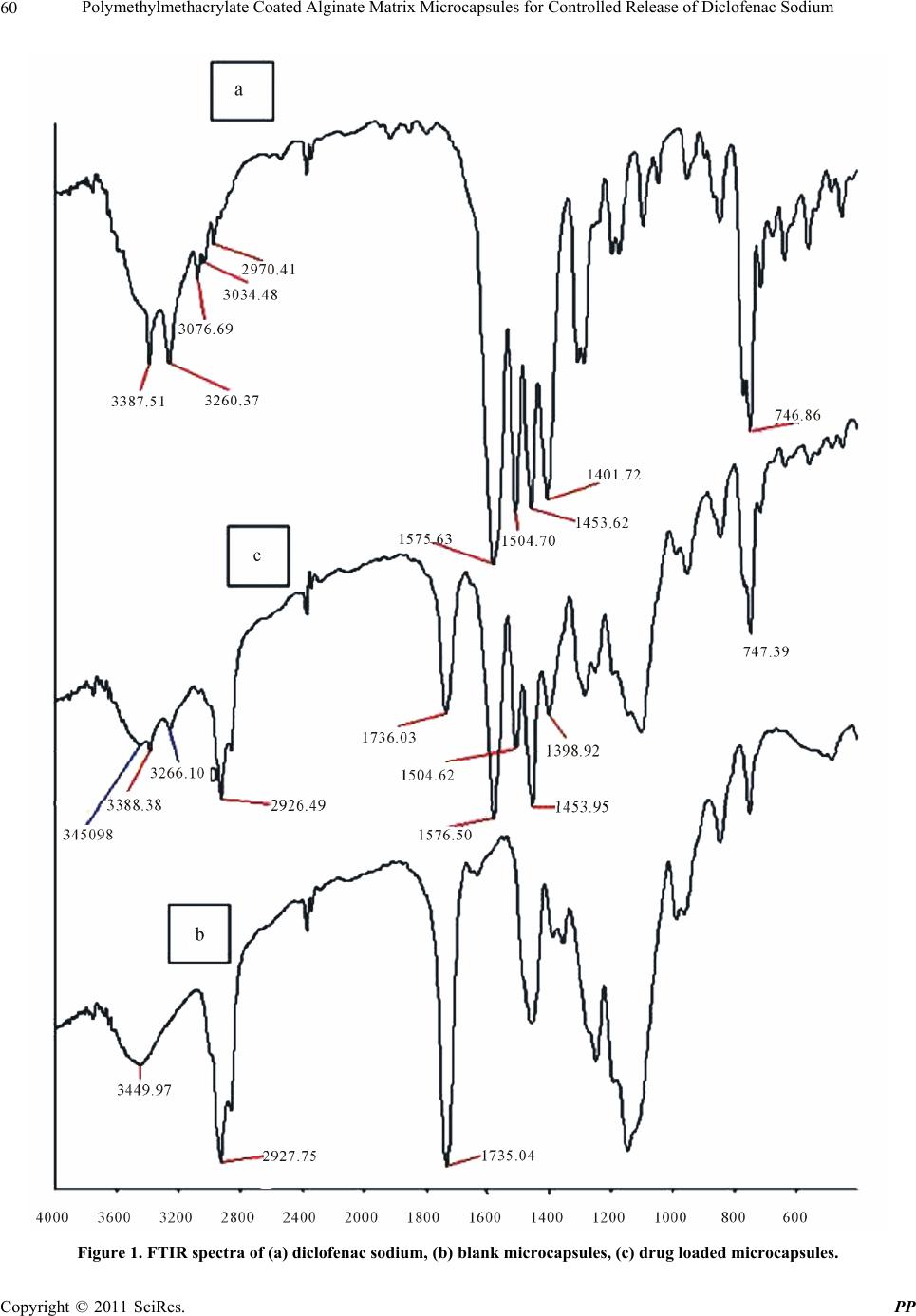 Polymethylmethacrylate Coated Alginate Matrix Microcapsules for Controlled Release of Diclofenac Sodium Copyright © 2011 SciRes. PP 60 Figure 1. FTIR spectra of (a) diclofenac sodium, (b) blank microcapsules, (c) drug loaded microcapsules.
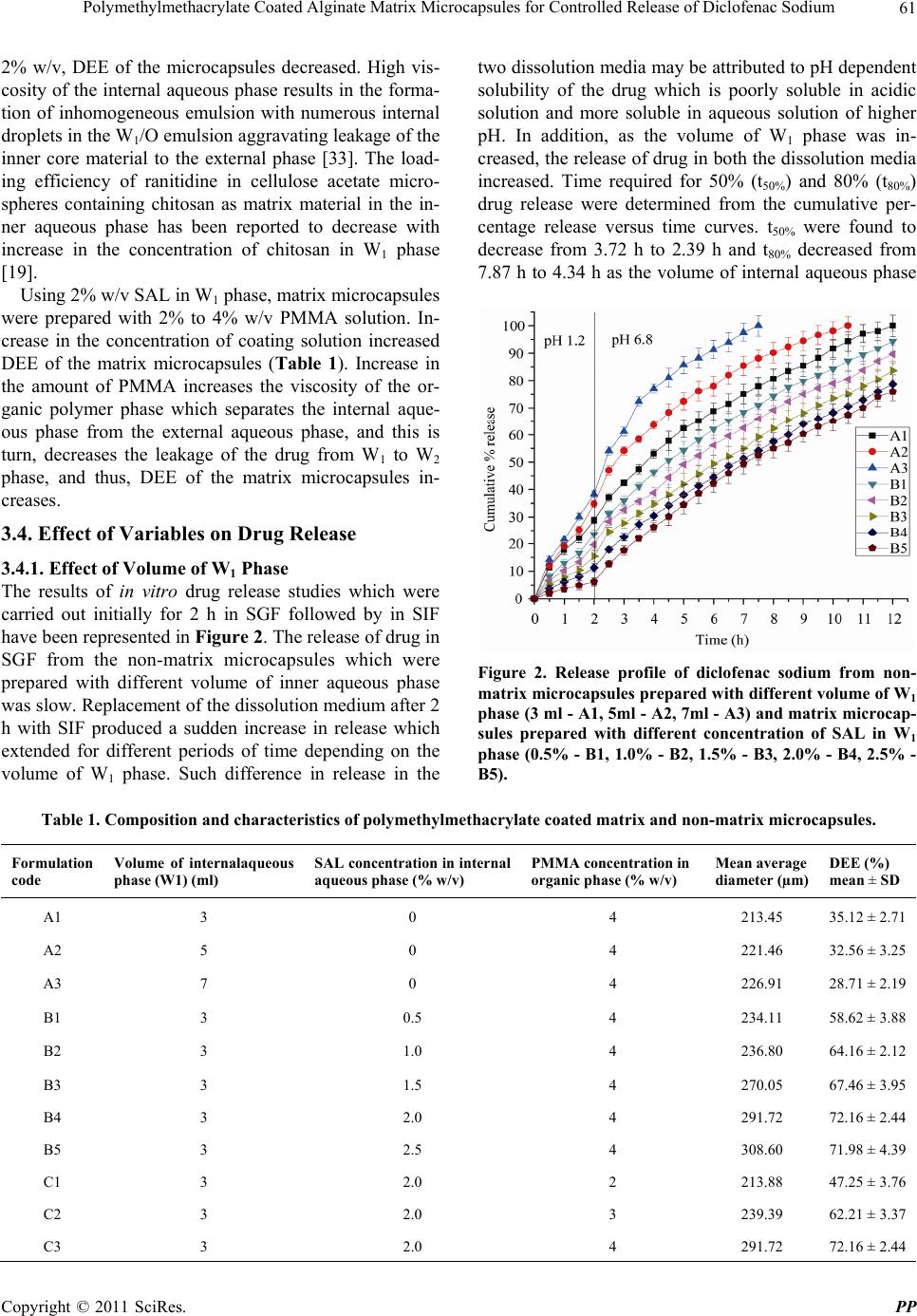 Polymethylmethacrylate Coated Alginate Matrix Microcapsules for Controlled Release of Diclofenac Sodium61 2% w/v, DEE of the microcapsules decreased. High vis- cosity of the internal aqueous phase results in the forma- tion of inhomogeneous emulsion with numerous internal droplets in the W1/O emulsion aggravating leakage of the inner core material to the external phase [33]. The load- ing efficiency of ranitidine in cellulose acetate micro- spheres containing chitosan as matrix material in the in- ner aqueous phase has been reported to decrease with increase in the concentration of chitosan in W1 phase [19]. Using 2% w/v SAL in W1 phase, matrix microcapsules were prepared with 2% to 4% w/v PMMA solution. In- crease in the concentration of coating solution increased DEE of the matrix microcapsules (Table 1). Increase in the amount of PMMA increases the viscosity of the or- ganic polymer phase which separates the internal aque- ous phase from the external aqueous phase, and this is turn, decreases the leakage of the drug from W1 to W2 phase, and thus, DEE of the matrix microcapsules in- creases. 3.4. Effect of Variables on Drug Release 3.4.1. Effect of Volume of W1 Phase The results of in vitro drug release studies which were carried out initially for 2 h in SGF followed by in SIF have been represented in Figure 2. The release of drug in SGF from the non-matrix microcapsules which were prepared with different volume of inner aqueous phase was slow. Replacement of the dissolution medium after 2 h with SIF produced a sudden increase in release which extended for different periods of time depending on the volume of W1 phase. Such difference in release in the two dissolution media may be attributed to pH dependent solubility of the drug which is poorly soluble in acidic solution and more soluble in aqueous solution of higher pH. In addition, as the volume of W1 phase was in- creased, the release of drug in both the dissolution media increased. Time required for 50% (t50%) and 80% (t80%) drug release were determined from the cumulative per- centage release versus time curves. t50% were found to decrease from 3.72 h to 2.39 h and t80% decreased from 7.87 h to 4.34 h as the volume of internal aqueous phase Figure 2. Release profile of diclofenac sodium from non- matrix microcapsules prepared with different volume of W1 phase (3 ml - A1, 5ml - A2, 7ml - A3) and matrix microcap- sules prepared with different concentration of SAL in W1 phase (0.5% - B1, 1.0% - B2, 1.5% - B3, 2.0% - B4, 2.5% - B5). Table 1. Composition and characteristics of polymethylmethacrylate coated matrix and non-matrix microcapsules. Formulation code Volume of internalaqueous phase (W1) (ml) SAL concentration in internal aqueous phase (% w/v) PMMA concentration in organic phase (% w/v) Mean average diameter (µm) DEE (%) mean ± SD A1 3 0 4 213.45 35.12 ± 2.71 A2 5 0 4 221.46 32.56 ± 3.25 A3 7 0 4 226.91 28.71 ± 2.19 B1 3 0.5 4 234.11 58.62 ± 3.88 B2 3 1.0 4 236.80 64.16 ± 2.12 B3 3 1.5 4 270.05 67.46 ± 3.95 B4 3 2.0 4 291.72 72.16 ± 2.44 B5 3 2.5 4 308.60 71.98 ± 4.39 C1 3 2.0 2 213.88 47.25 ± 3.76 C2 3 2.0 3 239.39 62.21 ± 3.37 C3 3 2.0 4 291.72 72.16 ± 2.44 Copyright © 2011 SciRes. PP
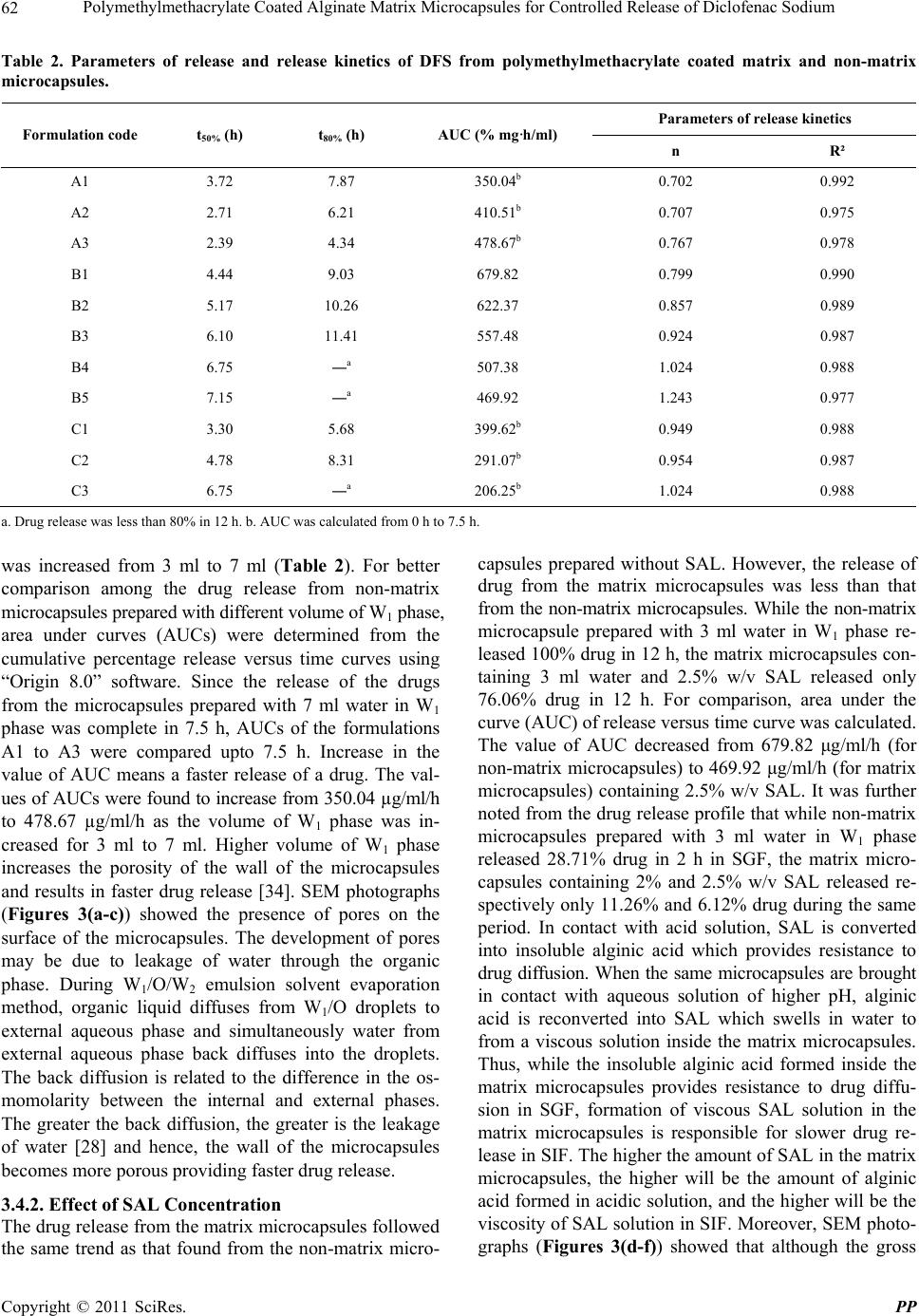 Polymethylmethacrylate Coated Alginate Matrix Microcapsules for Controlled Release of Diclofenac Sodium 62 Table 2. Parameters of release and release kinetics of DFS from polymethylmethacrylate coated matrix and non-matrix microcapsules. Parameters of release kinetics Formulation code t50% (h) t80% (h) AUC (% mg·h/ml) n R² A1 3.72 7.87 350.04b 0.702 0.992 A2 2.71 6.21 410.51b 0.707 0.975 A3 2.39 4.34 478.67b 0.767 0.978 B1 4.44 9.03 679.82 0.799 0.990 B2 5.17 10.26 622.37 0.857 0.989 B3 6.10 11.41 557.48 0.924 0.987 B4 6.75 ―a 507.38 1.024 0.988 B5 7.15 ―a 469.92 1.243 0.977 C1 3.30 5.68 399.62b 0.949 0.988 C2 4.78 8.31 291.07b 0.954 0.987 C3 6.75 ―a 206.25b 1.024 0.988 a. Drug release was less than 80% in 12 h. b. AUC was calculated from 0 h to 7.5 h. was increased from 3 ml to 7 ml (Table 2). For better comparison among the drug release from non-matrix microcapsules prepared with different volume of W1 phase, area under curves (AUCs) were determined from the cumulative percentage release versus time curves using “Origin 8.0” software. Since the release of the drugs from the microcapsules prepared with 7 ml water in W1 phase was complete in 7.5 h, AUCs of the formulations A1 to A3 were compared upto 7.5 h. Increase in the value of AUC means a faster release of a drug. The val- ues of AUCs were found to increase from 350.04 µg/ml/h to 478.67 µg/ml/h as the volume of W1 phase was in- creased for 3 ml to 7 ml. Higher volume of W1 phase increases the porosity of the wall of the microcapsules and results in faster drug release [34]. SEM photographs (Figures 3(a-c)) showed the presence of pores on the surface of the microcapsules. The development of pores may be due to leakage of water through the organic phase. During W1/O/W2 emulsion solvent evaporation method, organic liquid diffuses from W1/O droplets to external aqueous phase and simultaneously water from external aqueous phase back diffuses into the droplets. The back diffusion is related to the difference in the os- momolarity between the internal and external phases. The greater the back diffusion, the greater is the leakage of water [28] and hence, the wall of the microcapsules becomes more porous providing faster drug release. 3.4.2. Effect of SAL Concentration The drug release from the matrix microcapsules followed the same trend as that found from the non-matrix micro- capsules prepared without SAL. However, the release of drug from the matrix microcapsules was less than that from the non-matrix microcapsules. While the non-matrix microcapsule prepared with 3 ml water in W1 phase re- leased 100% drug in 12 h, the matrix microcapsules con- taining 3 ml water and 2.5% w/v SAL released only 76.06% drug in 12 h. For comparison, area under the curve (AUC) of release versus time curve was calculated. The value of AUC decreased from 679.82 μg/ml/h (for non-matrix microcapsules) to 469.92 μg/ml/h (for matrix microcapsules) containing 2.5% w/v SAL. It was further noted from the drug release profile that while non-matrix microcapsules prepared with 3 ml water in W1 phase released 28.71% drug in 2 h in SGF, the matrix micro- capsules containing 2% and 2.5% w/v SAL released re- spectively only 11.26% and 6.12% drug during the same period. In contact with acid solution, SAL is converted into insoluble alginic acid which provides resistance to drug diffusion. When the same microcapsules are brought in contact with aqueous solution of higher pH, alginic acid is reconverted into SAL which swells in water to from a viscous solution inside the matrix microcapsules. Thus, while the insoluble alginic acid formed inside the matrix microcapsules provides resistance to drug diffu- sion in SGF, formation of viscous SAL solution in the matrix microcapsules is responsible for slower drug re- lease in SIF. The higher the amount of SAL in the matrix microcapsules, the higher will be the amount of alginic acid formed in acidic solution, and the higher will be the viscosity of SAL solution in SIF. Moreover, SEM photo- graphs (Figures 3(d-f)) showed that although the gross Copyright © 2011 SciRes. PP
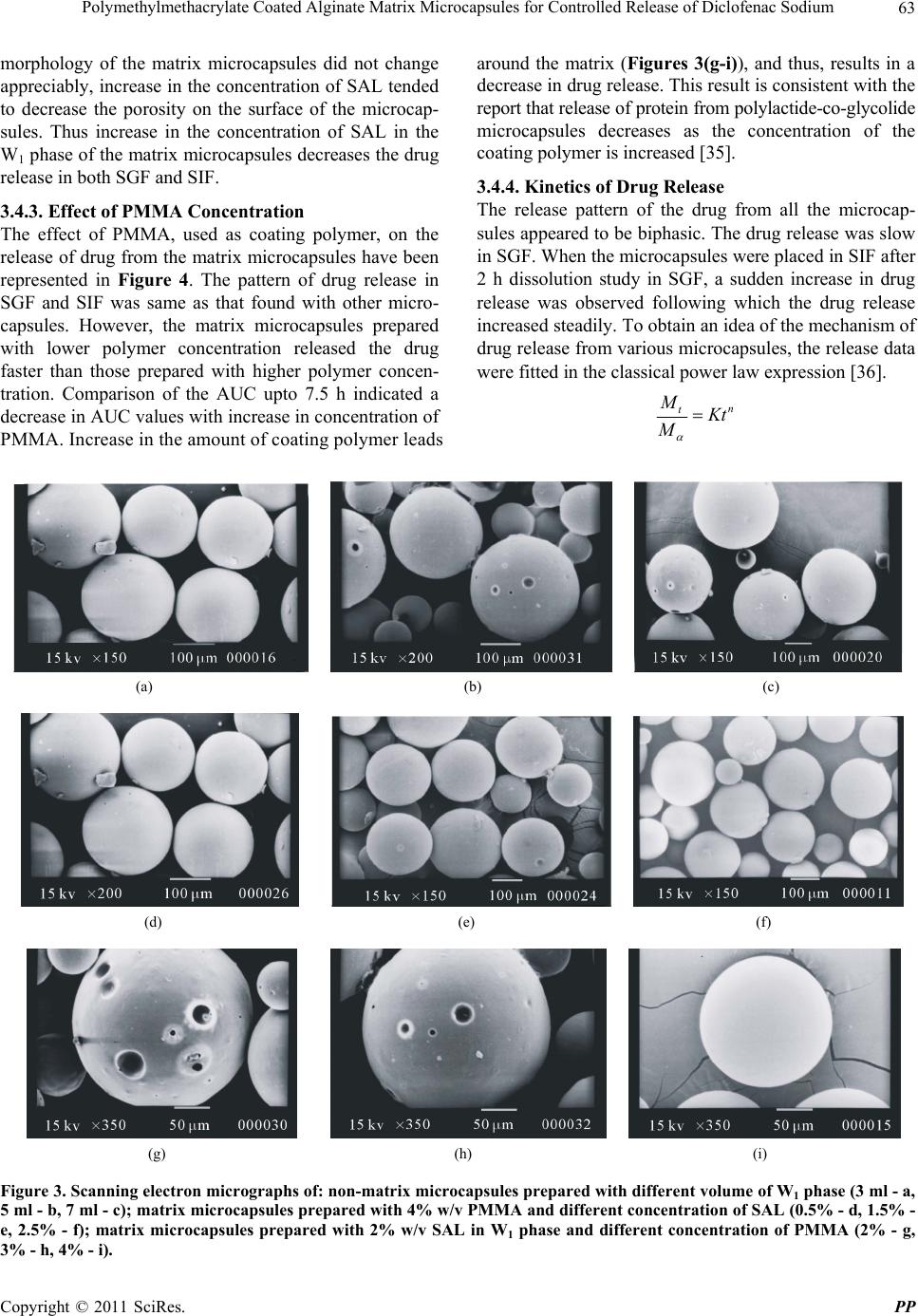 Polymethylmethacrylate Coated Alginate Matrix Microcapsules for Controlled Release of Diclofenac Sodium63 morphology of the matrix microcapsules did not change appreciably, increase in the concentration of SAL tended to decrease the porosity on the surface of the microcap- sules. Thus increase in the concentration of SAL in the W1 phase of the matrix microcapsules decreases the drug release in both SGF and SIF. 3.4.3. Effect of PMMA Concentration The effect of PMMA, used as coating polymer, on the release of drug from the matrix microcapsules have been represented in Figure 4. The pattern of drug release in SGF and SIF was same as that found with other micro- capsules. However, the matrix microcapsules prepared with lower polymer concentration released the drug faster than those prepared with higher polymer concen- tration. Comparison of the AUC upto 7.5 h indicated a decrease in AUC values with increase in concentration of PMMA. Increase in the amount of coating polymer leads around the matrix (Figures 3(g-i)), and thus, results in a decrease in drug release. This result is consistent with the report that release of protein from polylactide-co-glycolide microcapsules decreases as the concentration of the coating polymer is increased [35]. 3.4.4. Kinetics of Drug Release The release pattern of the drug from all the microcap- sules appeared to be biphasic. The drug release was slow in SGF. When the microcapsules were placed in SIF after 2 h dissolution study in SGF, a sudden increase in drug release was observed following which the drug release increased steadily. To obtain an idea of the mechanism of drug release from various microcapsules, the release data were fitted in the classical power law expression [36]. n t M t M (a) (b) (c) (d) (e) (f) (g) (h) (i) Figure 3. Scanning e lectron mic rographs of: non-matrix microcapsules prepared with different volume of W1 phase (3 ml - a, 5 ml - b, 7 ml - c); matrix microcapsules prepared with 4% w/v PMMA and different concentration of SAL (0.5% - d, 1.5% - e, 2.5% - f); matrix microcapsules prepared with 2% w/v SAL in W1 phase and different concentration of PMMA (2% - g, 3% - h, 4% - i). Copyright © 2011 SciRes. PP
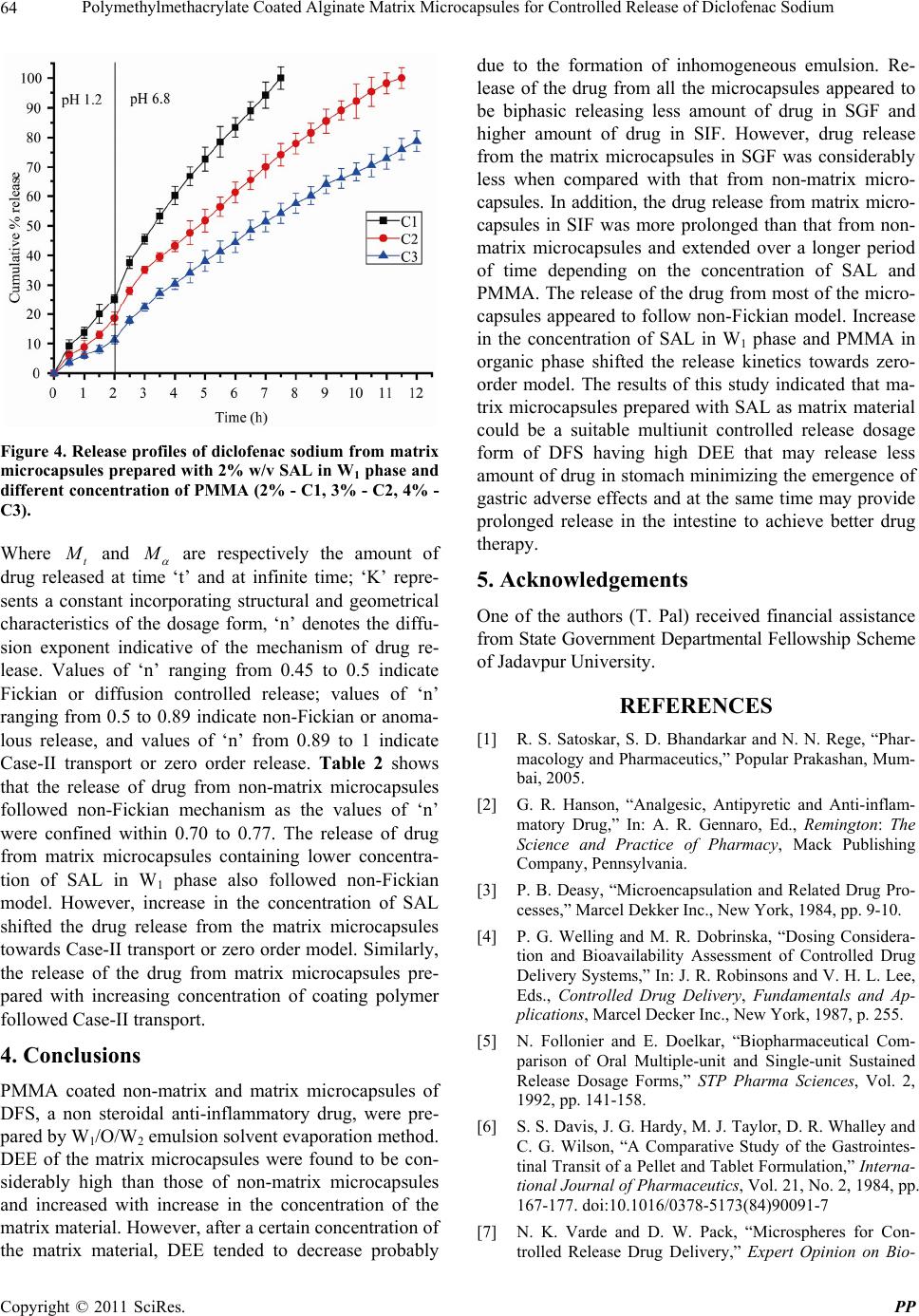 Polymethylmethacrylate Coated Alginate Matrix Microcapsules for Controlled Release of Diclofenac Sodium 64 Figure 4. Release profiles of diclofenac sodium from matrix microcapsules prepared with 2% w/v SAL in W1 phase and different concentration of PMMA (2% - C1, 3% - C2, 4% - C3). Where t and are respectively the amount of drug released at time ‘t’ and at infinite time; ‘K’ repre- sents a constant incorporating structural and geometrical characteristics of the dosage form, ‘n’ denotes the diffu- sion exponent indicative of the mechanism of drug re- lease. Values of ‘n’ ranging from 0.45 to 0.5 indicate Fickian or diffusion controlled release; values of ‘n’ ranging from 0.5 to 0.89 indicate non-Fickian or anoma- lous release, and values of ‘n’ from 0.89 to 1 indicate Case-II transport or zero order release. Table 2 shows that the release of drug from non-matrix microcapsules followed non-Fickian mechanism as the values of ‘n’ were confined within 0.70 to 0.77. The release of drug from matrix microcapsules containing lower concentra- tion of SAL in W1 phase also followed non-Fickian model. However, increase in the concentration of SAL shifted the drug release from the matrix microcapsules towards Case-II transport or zero order model. Similarly, the release of the drug from matrix microcapsules pre- pared with increasing concentration of coating polymer followed Case-II transport. 4. Conclusions PMMA coated non-matrix and matrix microcapsules of DFS, a non steroidal anti-inflammatory drug, were pre- pared by W1/O/W2 emulsion solvent evaporation method. DEE of the matrix microcapsules were found to be con- siderably high than those of non-matrix microcapsules and increased with increase in the concentration of the matrix material. However, after a certain concentration of the matrix material, DEE tended to decrease probably due to the formation of inhomogeneous emulsion. Re- lease of the drug from all the microcapsules appeared to be biphasic releasing less amount of drug in SGF and higher amount of drug in SIF. However, drug release from the matrix microcapsules in SGF was considerably less when compared with that from non-matrix micro- capsules. In addition, the drug release from matrix micro- capsules in SIF was more prolonged than that from non- matrix microcapsules and extended over a longer period of time depending on the concentration of SAL and PMMA. The release of the drug from most of the micro- capsules appeared to follow non-Fickian model. Increase in the concentration of SAL in W1 phase and PMMA in organic phase shifted the release kinetics towards zero- order model. The results of this study indicated that ma- trix microcapsules prepared with SAL as matrix material could be a suitable multiunit controlled release dosage form of DFS having high DEE that may release less amount of drug in stomach minimizing the emergence of gastric adverse effects and at the same time may provide prolonged release in the intestine to achieve better drug therapy. 5. Acknowledgements One of the authors (T. Pal) received financial assistance from State Government Departmental Fellowship Scheme of Jadavpur University. REFERENCES [1] R. S. Satoskar, S. D. Bhandarkar and N. N. Rege, “Phar- macology and Pharmaceutics,” Popular Prakashan, Mum- bai, 2005. [2] G. R. Hanson, “Analgesic, Antipyretic and Anti-inflam- matory Drug,” In: A. R. Gennaro, Ed., Remington: The Science and Practice of Pharmacy, Mack Publishing Company, Pennsylvania. [3] P. B. Deasy, “Microencapsulation and Related Drug Pro- cesses,” Marcel Dekker Inc., New York, 1984, pp. 9-10. [4] P. G. Welling and M. R. Dobrinska, “Dosing Considera- tion and Bioavailability Assessment of Controlled Drug Delivery Systems,” In: J. R. Robinsons and V. H. L. Lee, Eds., Controlled Drug Delivery, Fundamentals and Ap- plications, Marcel Decker Inc., New York, 1987, p. 255. [5] N. Follonier and E. Doelkar, “Biopharmaceutical Com- parison of Oral Multiple-unit and Single-unit Sustained Release Dosage Forms,” STP Pharma Sciences, Vol. 2, 1992, pp. 141-158. [6] S. S. Davis, J. G. Hardy, M. J. Taylor, D. R. Whalley and C. G. Wilson, “A Comparative Study of the Gastrointes- tinal Transit of a Pellet and Tablet Formulation,” Interna- tional Journal of Pharmaceutics, Vol. 21, No. 2, 1984, pp. 167-177. doi:10.1016/0378-5173(84)90091-7 [7] N. K. Varde and D. W. Pack, “Microspheres for Con- trolled Release Drug Delivery,” Expert Opinion on Bio- Copyright © 2011 SciRes. PP
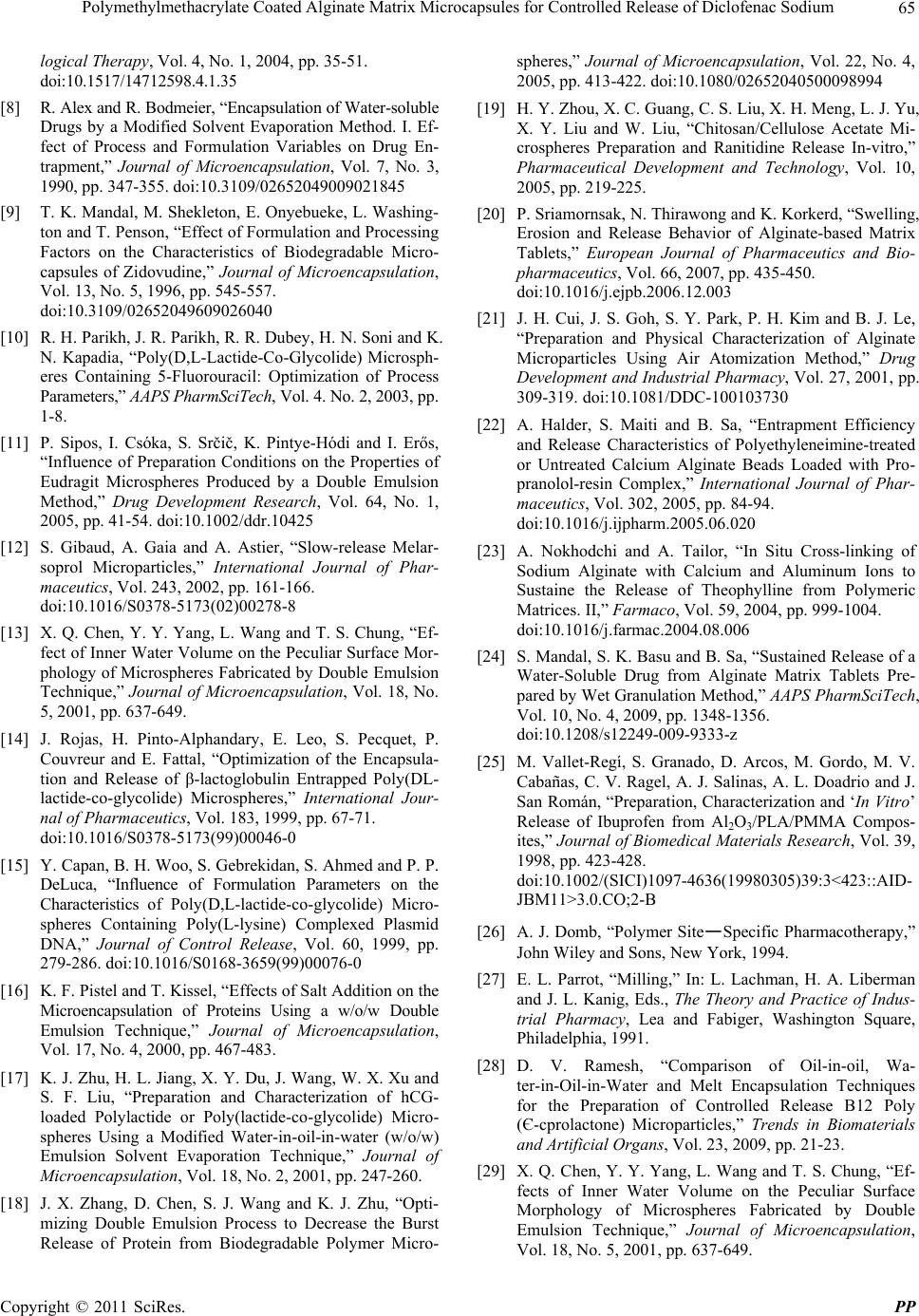 Polymethylmethacrylate Coated Alginate Matrix Microcapsules for Controlled Release of Diclofenac Sodium65 logical Therapy, Vol. 4, No. 1, 2004, pp. 35-51. doi:10.1517/14712598.4.1.35 [8] R. Alex and R. Bodmeier, “Encapsulation of Water-soluble Drugs by a Modified Solvent Evaporation Method. I. Ef- fect of Process and Formulation Variables on Drug En- trapment,” Journal of Microencapsulation, Vol. 7, No. 3, 1990, pp. 347-355. doi:10.3109/02652049009021845 [9] T. K. Mandal, M. Shekleton, E. Onyebueke, L. Washing- ton and T. Penson, “Effect of Formulation and Processing Factors on the Characteristics of Biodegradable Micro- capsules of Zidovudine,” Journal of Microencapsulation, Vol. 13, No. 5, 1996, pp. 545-557. doi:10.3109/02652049609026040 [10] R. H. Parikh, J. R. Parikh, R. R. Dubey, H. N. Soni and K. N. Kapadia, “Poly(D,L-Lactide-Co-Glycolide) Microsph- eres Containing 5-Fluorouracil: Optimization of Process Parameters,” AAPS PharmSciTech, Vol. 4. No. 2, 2003, pp. 1-8. [11] P. Sipos, I. Csóka, S. Srčič, K. Pintye-Hódi and I. Erős, “Influence of Preparation Conditions on the Properties of Eudragit Microspheres Produced by a Double Emulsion Method,” Drug Development Research, Vol. 64, No. 1, 2005, pp. 41-54. doi:10.1002/ddr.10425 [12] S. Gibaud, A. Gaia and A. Astier, “Slow-release Melar- soprol Microparticles,” International Journal of Phar- maceutics, Vol. 243, 2002, pp. 161-166. doi:10.1016/S0378-5173(02)00278-8 [13] X. Q. Chen, Y. Y. Yang, L. Wang and T. S. Chung, “Ef- fect of Inner Water Volume on the Peculiar Surface Mor- phology of Microspheres Fabricated by Double Emulsion Technique,” Journal of Microencapsulation, Vol. 18, No. 5, 2001, pp. 637-649. [14] J. Rojas, H. Pinto-Alphandary, E. Leo, S. Pecquet, P. Couvreur and E. Fattal, “Optimization of the Encapsula- tion and Release of β-lactoglobulin Entrapped Poly(DL- lactide-co-glycolide) Microspheres,” International Jour- nal of Pharmaceutics, Vol. 183, 1999, pp. 67-71. doi:10.1016/S0378-5173(99)00046-0 [15] Y. Capan, B. H. Woo, S. Gebrekidan, S. Ahmed and P. P. DeLuca, “Influence of Formulation Parameters on the Characteristics of Poly(D,L-lactide-co-glycolide) Micro- spheres Containing Poly(L-lysine) Complexed Plasmid DNA,” Journal of Control Release, Vol. 60, 1999, pp. 279-286. doi:10.1016/S0168-3659(99)00076-0 [16] K. F. Pistel and T. Kissel, “Effects of Salt Addition on the Microencapsulation of Proteins Using a w/o/w Double Emulsion Technique,” Journal of Microencapsulation, Vol. 17, No. 4, 2000, pp. 467-483. [17] K. J. Zhu, H. L. Jiang, X. Y. Du, J. Wang, W. X. Xu and S. F. Liu, “Preparation and Characterization of hCG- loaded Polylactide or Poly(lactide-co-glycolide) Micro- spheres Using a Modified Water-in-oil-in-water (w/o/w) Emulsion Solvent Evaporation Technique,” Journal of Microencapsulation, Vol. 18, No. 2, 2001, pp. 247-260. [18] J. X. Zhang, D. Chen, S. J. Wang and K. J. Zhu, “Opti- mizing Double Emulsion Process to Decrease the Burst Release of Protein from Biodegradable Polymer Micro- spheres,” Journal of Microencapsulation, Vol. 22, No. 4, 2005, pp. 413-422. doi:10.1080/02652040500098994 [19] H. Y. Zhou, X. C. Guang, C. S. Liu, X. H. Meng, L. J. Yu, X. Y. Liu and W. Liu, “Chitosan/Cellulose Acetate Mi- crospheres Preparation and Ranitidine Release In-vitro,” Pharmaceutical Development and Technology, Vol. 10, 2005, pp. 219-225. [20] P. Sriamornsak, N. Thirawong and K. Korkerd, “Swelling, Erosion and Release Behavior of Alginate-based Matrix Tablets,” European Journal of Pharmaceutics and Bio- pharmaceutics, Vol. 66, 2007, pp. 435-450. doi:10.1016/j.ejpb.2006.12.003 [21] J. H. Cui, J. S. Goh, S. Y. Park, P. H. Kim and B. J. Le, “Preparation and Physical Characterization of Alginate Microparticles Using Air Atomization Method,” Drug Development and Industrial Pharmacy, Vol. 27, 2001, pp. 309-319.doi:10.1081/DDC-100103730 [22] A. Halder, S. Maiti and B. Sa, “Entrapment Efficiency and Release Characteristics of Polyethyleneimine-treated or Untreated Calcium Alginate Beads Loaded with Pro- pranolol-resin Complex,” International Journal of Phar- maceutics, Vol. 302, 2005, pp. 84-94. doi:10.1016/j.ijpharm.2005.06.020 [23] A. Nokhodchi and A. Tailor, “In Situ Cross-linking of Sodium Alginate with Calcium and Aluminum Ions to Sustaine the Release of Theophylline from Polymeric Matrices. II,” Farmaco, Vol. 59, 2004, pp. 999-1004. doi:10.1016/j.farmac.2004.08.006 [24] S. Mandal, S. K. Basu and B. Sa, “Sustained Release of a Water-Soluble Drug from Alginate Matrix Tablets Pre- pared by Wet Granulation Method,” AAPS PharmSciTech, Vol. 10, No. 4, 2009, pp. 1348-1356. doi:10.1208/s12249-009-9333-z [25] M. Vallet-Regí, S. Granado, D. Arcos, M. Gordo, M. V. Cabañas, C. V. Ragel, A. J. Salinas, A. L. Doadrio and J. San Román, “Preparation, Characterization and ‘In Vitro’ Release of Ibuprofen from Al2O3/PLA/PMMA Compos- ites,” Journal of Biomedical Materials Research, Vol. 39, 1998, pp. 423-428. doi:10.1002/(SICI)1097-4636(19980305)39:3<423::AID- JBM11>3.0.CO;2-B [26] A. J. Domb, “Polymer Site—Specific Pharmacotherapy,” John Wiley and Sons, New York, 1994. [27] E. L. Parrot, “Milling,” In: L. Lachman, H. A. Liberman and J. L. Kanig, Eds., The Theory and Practice of Indus- trial Pharmacy, Lea and Fabiger, Washington Square, Philadelphia, 1991. [28] D. V. Ramesh, “Comparison of Oil-in-oil, Wa- ter-in-Oil-in-Water and Melt Encapsulation Techniques for the Preparation of Controlled Release B12 Poly (Є-cprolactone) Microparticles,” Trends in Biomaterials and Artificial Organs, Vol. 23, 2009, pp. 21-23. [29] X. Q. Chen, Y. Y. Yang, L. Wang and T. S. Chung, “Ef- fects of Inner Water Volume on the Peculiar Surface Morphology of Microspheres Fabricated by Double Emulsion Technique,” Journal of Microencapsulation, Vol. 18, No. 5, 2001, pp. 637-649. Copyright © 2011 SciRes. PP
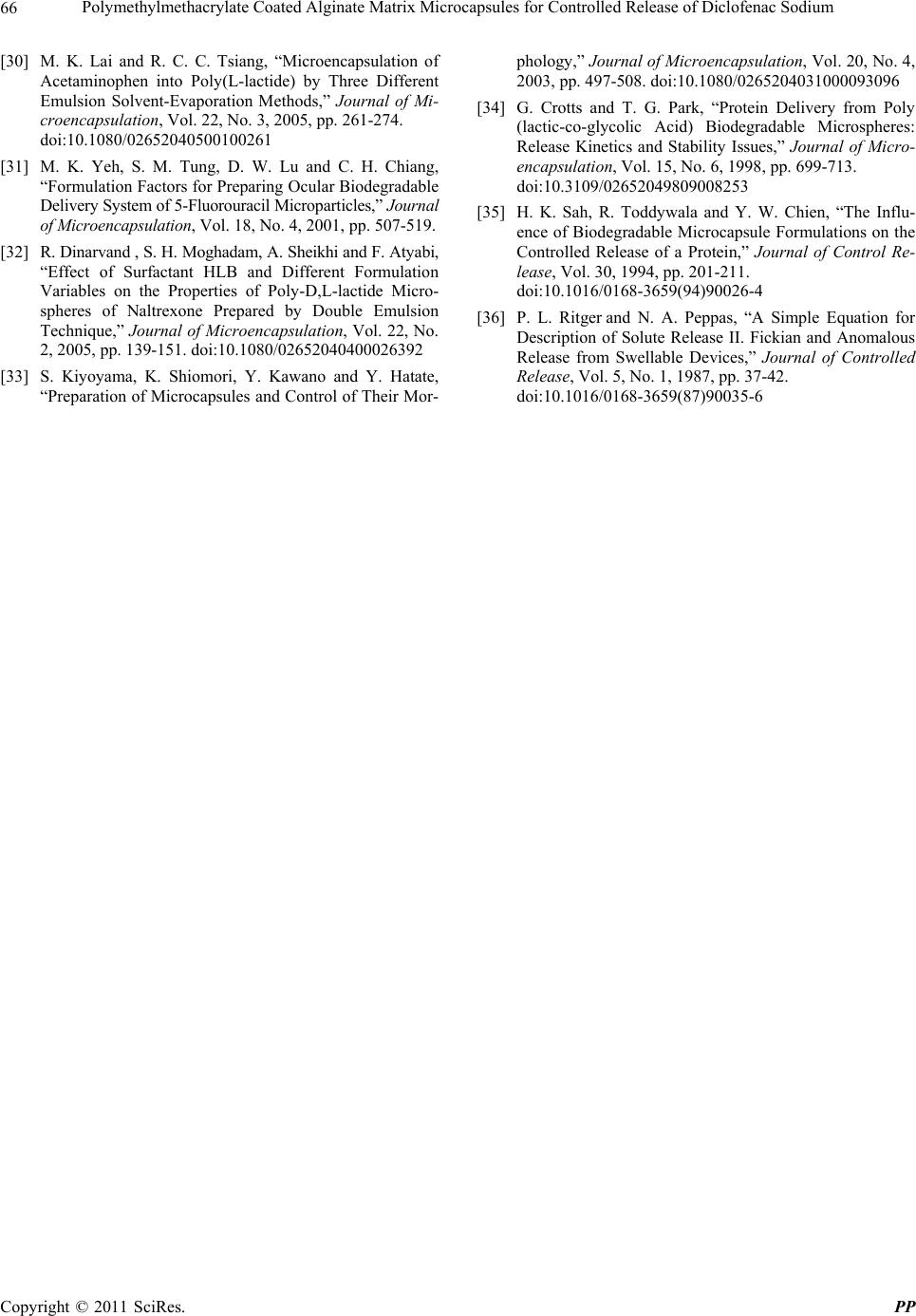 Polymethylmethacrylate Coated Alginate Matrix Microcapsules for Controlled Release of Diclofenac Sodium Copyright © 2011 SciRes. PP 66 [30] M. K. Lai and R. C. C. Tsiang, “Microencapsulation of Acetaminophen into Poly(L-lactide) by Three Different Emulsion Solvent-Evaporation Methods,” Journal of Mi- croencapsulation, Vol. 22, No. 3, 2005, pp. 261-274. doi:10.1080/02652040500100261 [31] M. K. Yeh, S. M. Tung, D. W. Lu and C. H. Chiang, “Formulation Factors for Preparing Ocular Biodegradable Delivery System of 5-Fluorouracil Microparticles,” Journal of Microencapsulation, Vol. 18, No. 4, 2001, pp. 507-519. [32] R. Dinarvand , S. H. Moghadam, A. Sheikhi and F. Atyabi, “Effect of Surfactant HLB and Different Formulation Variables on the Properties of Poly-D,L-lactide Micro- spheres of Naltrexone Prepared by Double Emulsion Technique,” Journal of Microencapsulation, Vol. 22, No. 2, 2005, pp. 139-151. doi:10.1080/02652040400026392 [33] S. Kiyoyama, K. Shiomori, Y. Kawano and Y. Hatate, “Preparation of Microcapsules and Control of Their Mor- phology,” Journal of Microencapsulation, Vol. 20, No. 4, 2003, pp. 497-508. doi:10.1080/0265204031000093096 [34] G. Crotts and T. G. Park, “Protein Delivery from Poly (lactic-co-glycolic Acid) Biodegradable Microspheres: Release Kinetics and Stability Issues,” Journal of Micro- encapsulation, Vol. 15, No. 6, 1998, pp. 699-713. doi:10.3109/02652049809008253 [35] H. K. Sah, R. Toddywala and Y. W. Chien, “The Influ- ence of Biodegradable Microcapsule Formulations on the Controlled Release of a Protein,” Journal of Control Re- lease, Vol. 30, 1994, pp. 201-211. doi:10.1016/0168-3659(94)90026-4 [36] P. L. Ritger and N. A. Peppas, “A Simple Equation for Description of Solute Release II. Fickian and Anomalous Release from Swellable Devices,” Journal of Controlled Release, Vol. 5, No. 1, 1987, pp. 37-42. doi:10.1016/0168-3659(87)90035-6
|