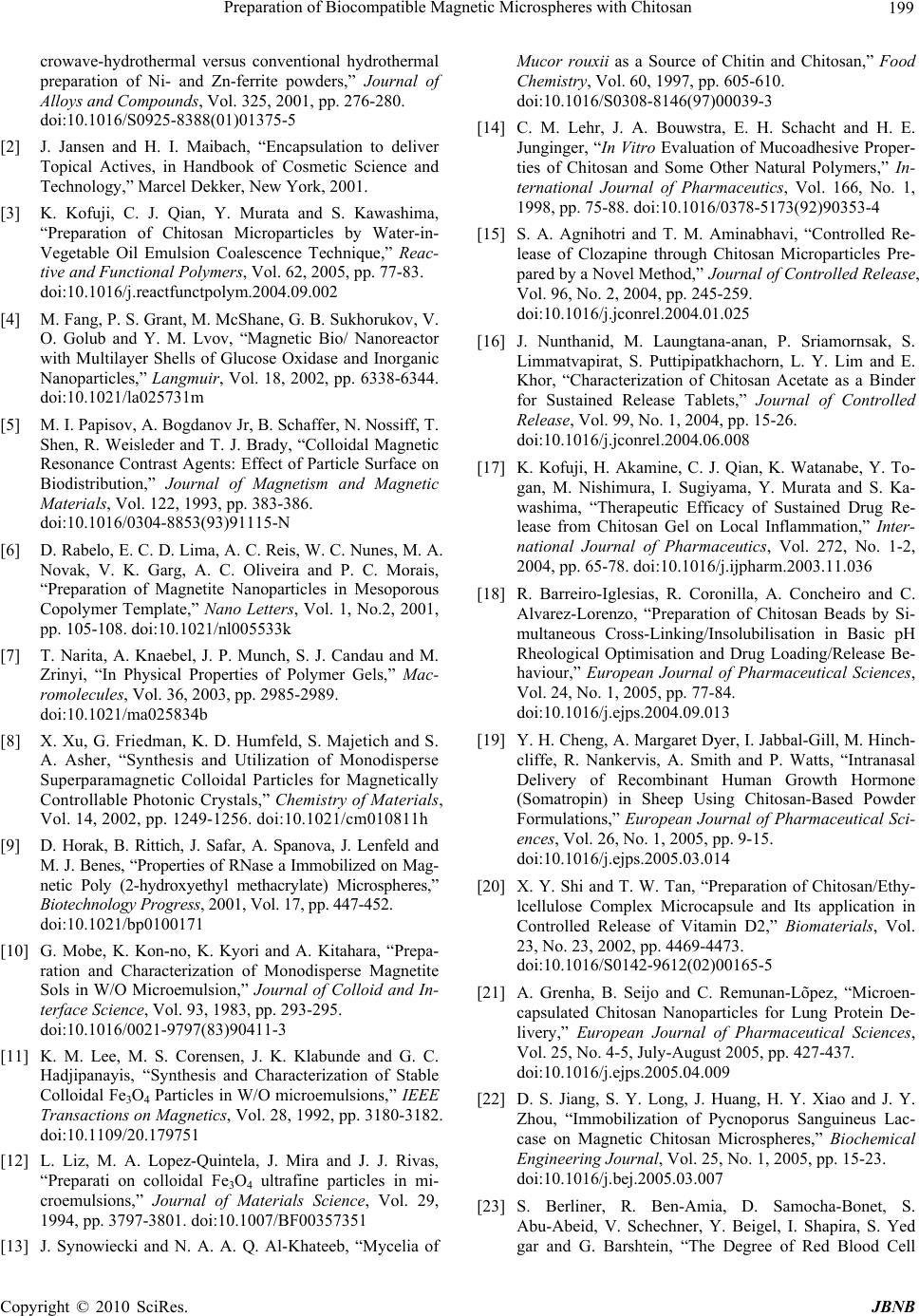
Preparation of Biocompatible Magnetic Microspheres with Chitosan 199
crowave-hydrothermal versus conventional hydrothermal
preparation of Ni- and Zn-ferrite powders,” Journal of
Alloys and Compounds, Vol. 325, 2001, pp. 276-280.
doi:10.1016/S0925-8388(01)01375-5
[2] J. Jansen and H. I. Maibach, “Encapsulation to deliver
Topical Actives, in Handbook of Cosmetic Science and
Technology,” Marcel Dekker, New York, 2001.
[3] K. Kofuji, C. J. Qian, Y. Murata and S. Kawashima,
“Preparation of Chitosan Microparticles by Water-in-
Vegetable Oil Emulsion Coalescence Technique,” Reac-
tive and Functional Polymers, Vol. 62, 2005, pp. 77-83.
doi:10.1016/j.reactfunctpolym.2004.09.002
[4] M. Fang, P. S. Grant, M. McShane, G. B. Sukhorukov, V.
O. Golub and Y. M. Lvov, “Magnetic Bio/ Nanoreactor
with Multilayer Shells of Glucose Oxidase and Inorganic
Nanoparticles,” Langmuir, Vol. 18, 2002, pp. 6338-6344.
doi:10.1021/la025731m
[5] M. I. Papisov, A. Bogdanov Jr, B. Schaffer, N. Nossiff, T.
Shen, R. Weisleder and T. J. Brady, “Colloidal Magnetic
Resonance Contrast Agents: Effect of Particle Surface on
Biodistribution,” Journal of Magnetism and Magnetic
Materials, Vol. 122, 1993, pp. 383-386.
doi:10.1016/0304-8853(93)91115-N
[6] D. Rabelo, E. C. D. Lima, A. C. Reis, W. C. Nunes, M. A.
Novak, V. K. Garg, A. C. Oliveira and P. C. Morais,
“Preparation of Magnetite Nanoparticles in Mesoporous
Copolymer Template,” Nano Letters, Vol. 1, No.2, 2001,
pp. 105-108. doi:10.1021/nl005533k
[7] T. Narita, A. Knaebel, J. P. Munch, S. J. Candau and M.
Zrinyi, “In Physical Properties of Polymer Gels,” Mac-
romolecules, Vol. 36, 2003, pp. 2985-2989.
doi:10.1021/ma025834b
[8] X. Xu, G. Friedman, K. D. Humfeld, S. Majetich and S.
A. Asher, “Synthesis and Utilization of Monodisperse
Superparamagnetic Colloidal Particles for Magnetically
Controllable Photonic Crystals,” Chemistry of Materials,
Vol. 14, 2002, pp. 1249-1256. doi:10.1021/cm010811h
[9] D. Horak, B. Rittich, J. Safar, A. Spanova, J. Lenfeld and
M. J. Benes, “Properties of RNase a Immobilized on Mag-
netic Poly (2-hydroxyethyl methacrylate) Microspheres,”
Biotechnology Progress, 2001, Vol. 17, pp. 447-452.
doi:10.1021/bp0100171
[10] G. Mobe, K. Kon-no, K. Kyori and A. Kitahara, “Prepa-
ration and Characterization of Monodisperse Magnetite
Sols in W/O Microemulsion,” Journal of Colloid and In-
terface Science, Vol. 93, 1983, pp. 293-295.
doi:10.1016/0021-9797(83)90411-3
[11] K. M. Lee, M. S. Corensen, J. K. Klabunde and G. C.
Hadjipanayis, “Synthesis and Characterization of Stable
Colloidal Fe3O4 Particles in W/O microemulsions,” IEEE
Transactions on Magnetics, Vol. 28, 1992, pp. 3180-3182.
doi:10.1109/20.179751
[12] L. Liz, M. A. Lopez-Quintela, J. Mira and J. J. Rivas,
“Preparati on colloidal Fe3O4 ultrafine particles in mi-
croemulsions,” Journal of Materials Science, Vol. 29,
1994, pp. 3797-3801. doi:10.1007/BF00357351
[13] J. Synowiecki and N. A. A. Q. Al-Khateeb, “Mycelia of
Mucor rouxii as a Source of Chitin and Chitosan,” Food
Chemistry, Vol. 60, 1997, pp. 605-610.
doi:10.1016/S0308-8146(97)00039-3
[14] C. M. Lehr, J. A. Bouwstra, E. H. Schacht and H. E.
Junginger, “In Vitro Evaluation of Mucoadhesive Proper-
ties of Chitosan and Some Other Natural Polymers,” In-
ternational Journal of Pharmaceutics, Vol. 166, No. 1,
1998, pp. 75-88. doi:10.1016/0378-5173(92)90353-4
[15] S. A. Agnihotri and T. M. Aminabhavi, “Controlled Re-
lease of Clozapine through Chitosan Microparticles Pre-
pared by a Novel Method,” Journal of Controlled Release,
Vol. 96, No. 2, 2004, pp. 245-259.
doi:10.1016/j.jconrel.2004.01.025
[16] J. Nunthanid, M. Laungtana-anan, P. Sriamornsak, S.
Limmatvapirat, S. Puttipipatkhachorn, L. Y. Lim and E.
Khor, “Characterization of Chitosan Acetate as a Binder
for Sustained Release Tablets,” Journal of Controlled
Release, Vol. 99, No. 1, 2004, pp. 15-26.
doi:10.1016/j.jconrel.2004.06.008
[17] K. Kofuji, H. Akamine, C. J. Qian, K. Watanabe, Y. To-
gan, M. Nishimura, I. Sugiyama, Y. Murata and S. Ka-
washima, “Therapeutic Efficacy of Sustained Drug Re-
lease from Chitosan Gel on Local Inflammation,” Inter-
national Journal of Pharmaceutics, Vol. 272, No. 1-2,
2004, pp. 65-78. doi:10.1016/j.ijpharm.2003.11.036
[18] R. Barreiro-Iglesias, R. Coronilla, A. Concheiro and C.
Alvarez-Lorenzo, “Preparation of Chitosan Beads by Si-
multaneous Cross-Linking/Insolubilisation in Basic pH
Rheological Optimisation and Drug Loading/Release Be-
haviour,” European Journal of Pharmaceutical Sciences,
Vol. 24, No. 1, 2005, pp. 77-84.
doi:10.1016/j.ejps.2004.09.013
[19] Y. H. Cheng, A. Margaret Dyer, I. Jabbal-Gill, M. Hinch-
cliffe, R. Nankervis, A. Smith and P. Watts, “Intranasal
Delivery of Recombinant Human Growth Hormone
(Somatropin) in Sheep Using Chitosan-Based Powder
Formulations,” European Journal of Pharmaceutical Sci-
ences, Vol. 26, No. 1, 2005, pp. 9-15.
doi:10.1016/j.ejps.2005.03.014
[20] X. Y. Shi and T. W. Tan, “Preparation of Chitosan/Ethy-
lcellulose Complex Microcapsule and Its application in
Controlled Release of Vitamin D2,” Biomaterials, Vol.
23, No. 23, 2002, pp. 4469-4473.
doi:10.1016/S0142-9612(02)00165-5
[21] A. Grenha, B. Seijo and C. Remunan-Lõpez, “Microen-
capsulated Chitosan Nanoparticles for Lung Protein De-
livery,” European Journal of Pharmaceutical Sciences,
Vol. 25, No. 4-5, July-August 2005, pp. 427-437.
doi:10.1016/j.ejps.2005.04.009
[22] D. S. Jiang, S. Y. Long, J. Huang, H. Y. Xiao and J. Y.
Zhou, “Immobilization of Pycnoporus Sanguineus Lac-
case on Magnetic Chitosan Microspheres,” Biochemical
Engineering Journal, Vol. 25, No. 1, 2005, pp. 15-23.
doi:10.1016/j.bej.2005.03.007
[23] S. Berliner, R. Ben-Amia, D. Samocha-Bonet, S.
Abu-Abeid, V. Schechner, Y. Beigel, I. Shapira, S. Yed
gar and G. Barshtein, “The Degree of Red Blood Cell
C
opyright © 2010 SciRes. JBNB