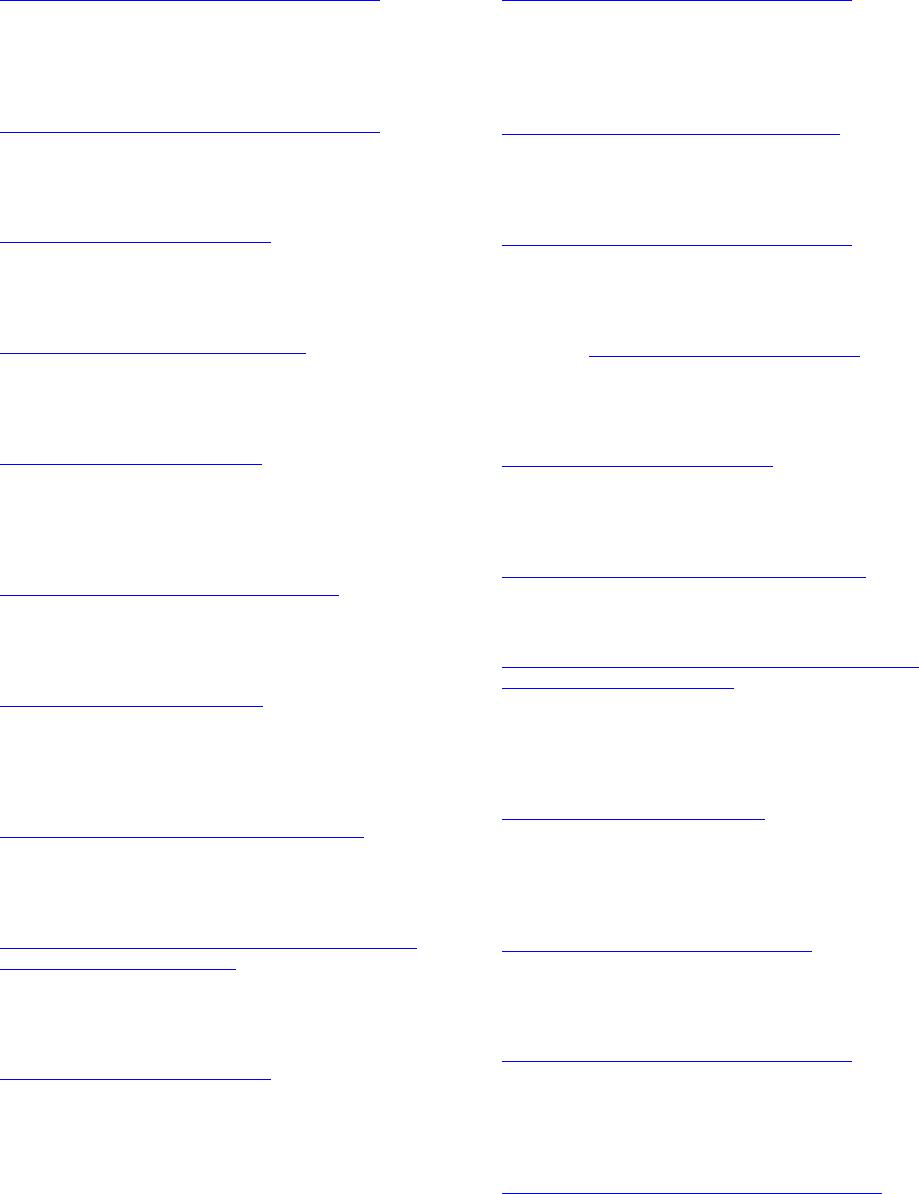
Q. ZHANG ET AL.
OPEN ACCESS MSCE
http://dx.doi.org/10.1016/j.compscitech.2008.05.021
[10] R. D. Farahani, H. Dalir, V. Le Borgne, L. A. Gautier, M.
A. El Khakani, M. Lévesque, et al., “Reinforcing Epoxy
Nanocomposites with Functionalized Carbon Nanotubes
via Biotin-Streptavidin Interactions,” Composites Science
and Technology, Vol. 72, No. 12, 2012, pp. 1387-1395.
http://dx.doi.org/10.1016/j.compscitech.2012.05.010
[11] X. Y. Gong, J. Liu, S. Baskaran, R. D. Voise and J. S.
Young, “Surfactant-Assisted Processing of Carbon Nano-
tube/Polymer Composites,” Chemistry of Materials, Vol.
12, No. 4, 2000, pp. 1049-1052.
http://dx.doi.org/10.1021/cm9906396
[12] C. Richard, F. Balavoine, P. Schultz, T. W. Ebbesen and
C. Mioskowski, “Supramolecular Self-Assembly of Lipid
Derivatives on Carbon Nanotubes,” Science, Vol. 300, No.
5620, 2003, pp. 775-778.
http://dx.doi.org/10.1126/science.1080848
[13] Q. Wang, Y. Han, Y. Wang, Y. Qin and Z.-X. Guo, “Ef-
fect of Surfactant Structure on the Stability of Carbon
Nanotubes in Aqueous Solution,” The Journal of Physical
Chemistry B, Vol. 112, No. 24, 2008, pp. 7227-7233.
http://dx.doi.org/10.1021/jp711816c
[14] M. N. Zhang, L. Su and L. Q. Mao, “Surfactant Function-
alization of Carbon Nanotubes (CNTs) for Laye r -by-
Layer Assembling of CNT Multi-Layer Films and Fabri-
cation of Gold Nanoparticle/CNT Nanohybrid,” Carbon,
Vol. 44, No. 2, 2006, pp. 276-283.
http://dx.doi.org/10.1016/j.carbon.2005.07.021
[15] H. J. Barraza, F. Pompeo, E. A. O’Rea and D. E. Resasco,
“SWN T-Filled Thermoplastic and Elastomeric Compos-
ites Prepared by Miniemulsion Polymerization,” Nano
Letters, Vol. 2, No. 8, 2002, pp. 797-802.
http://dx.doi.org/10.1021/nl0256208
[16] M. J. O'Connell, P. Boul, L. M. Ericson, C. Huffman, Y.
Wang, E. Haroz, et al., “Reversible Water-Solubilization
of Single-Walled Carbon Nanotubes by Polymer Wrap-
ping,” Chemical Physics Letters, Vol. 342, No. 3-4, 2001,
pp. 265-271.
http://dx.doi.org/10.1016/S0009-2614(01)00490-0
[17] H. J. Li, X. B. Wang, Y. L. Song, Y. Q. Liu, Q. S. Li, L.
Jiang, et al., “Super-Amphiphobic Aligned Carbon
Nanotube Films,” Angewandte Chemie International Edi-
tion, Vol. 40, No. 9, 2001, pp. 1743-1746.
http://dx.doi.org/10.1002/1521-3773(20010504)40:9<174
3::AID-ANIE17430>3.0.CO;2-#
[18] A. Kowalski, A. Duda and S. Penczek, “Kinetics and
Mechanism of Cyclic Esters Polymerization Initiated with
Tin(II) Octoate. 3.† Polymerization of l,l-Dilactide,”
Macromolecules, Vol. 33, No. 20, 2000, pp. 7359-7370.
http://dx.doi.org/10.1021/ma000125o
[19] V. Ambrogi, G. Gentile, C. Ducati, M. C. Oliva and C.
Carfagna, “Multiwalled Carbon Nanotubes Functional-
ized with Maleated Poly(propylene) by a Dry Mech-
ano-Chemical Process,” Polymer, Vol. 53, No. 2, 2012,
pp. 291-299.
http://dx.doi.org/10.1016/j.polymer.2011.11.048
[20] A. N. Chakoli, J. Wan, J. T. Feng, M. Amirian, J. H. Sui
and W. Cai, “Functionalization of Multiwalled Carbon
Nanotubes for Reinforcing of Poly(l-lactide-co-ɛ-capro-
lactone) Biodegradable Copolymers,” Applied Surface
Science, Vol. 256, No. 1, 2009, pp. 170-177.
http://dx.doi.org/10.1016/j.apsusc.2009.07.103
[21] J. Feng, W. Cai, J. Sui, Z. Li, J. Wan and A. N. Chakoli,
“P ol y(l-lactide) Brushes on Magnetic Multiwalled Carbon
Nanotubes by In-Situ Ring-Opening Polymerizatio n ,”
Polymer, Vol. 49, No. 23, 2008, pp. 4989-4994.
http://dx.doi.org/10.1016/j.polymer.2008.09.022
[22] H. X. Xu, X. B. Wang, Y. F. Zhang and S. Y. Liu, “Sin-
gle-Step in Situ Preparation of Polymer-Grafted Multi-
Walled Carbon Nanotube Composites under 60Co ç-Ray
Irradiation,” Chem Mater Vol. 18, No. 13, 2006, pp.
2929-2934. http://dx.doi.org/10.1021/cm052840o
[23] S. M. Chen, G. Z. Wu, Y. D. Liu and D. W. Long, “Pre-
paration of Poly(acrylic acid) Grafted Multiwalled Car-
bon Nanotubes by a Two-Step Irradiation Technique,”
Macromolecules, Vol. 39, No. 1, 2006, pp. 330-334.
http://dx.doi.org/10.1021/ma0520500
[24] J. Lunt, “Large -Scale Production, Properties and Com-
mercial Applications of Polylactic Acid Polymers,” Po ly-
mer Degradation and Stability, Vol. 59, No. 1-3, 1998, pp.
145-152.
http://dx.doi.org/10.1016/S0141-3910(97)00148-1
[25] R. E. Drumright, P. R. Gruber, D. E. Henton, “Polylactic
Acid Technology,” Advanced Materials, Vol. 12, No. 23,
2000, pp. 1841-1846.
http://dx.doi.org/10.1002/1521-4095(200012)12:23<1841
::AID-ADMA1841>3.0.CO;2-E
[26] G.-X. Chen, H.-S. Kim, B. H. Park and J.-S. Yoon, “Con-
trolled Functionalization of Multiwalled Carbon Nano-
tubes with Various Molecular-Weight Poly(l-lactic acid),”
The Journal of Physical Chemistry B, Vol. 109, No. 47,
2005, pp. 22237-22243.
http://dx.doi.org/10.1021/jp054768n
[27] G.-X. Chen, H.-S. Kim, B. H. Park and J.-S. Yoon,
“Synthesis of Poly(L-lactide)-Functionalized Multiwalled
Carbon Nanotubes by Ring-Opening Polymerization,”
Macromolecular Chemistry and Physics, Vol. 208, No. 4,
2007, pp. 389-398.
http://dx.doi.org/10.1002/macp.200600411
[28] W. Song, Z. Zheng, W. Tang and X. Wang, “A Facile
Approach to Covalently Functionalized Carbon Nano-
tubes with Biocompatible Polymer,” Polymer, Vol. 48,
No. 13, 2007, pp. 3658-3663.
http://dx.doi.org/10.1016/j.polymer.2007.04.071
[29] J. T. Yoon, S. C. Lee and Y. G. Jeong, “Effects of Grafted
Chain Length on Mechanical and Electrical Properties of
Nanocomposites Containing Polylactide-Grafted Carbon
Nanotubes,” Composites Science and Technology, Vol.
70, No. 5, 2010, pp. 776-782.
http://dx.doi.org/10.1016/j.compscitech.2010.01.011