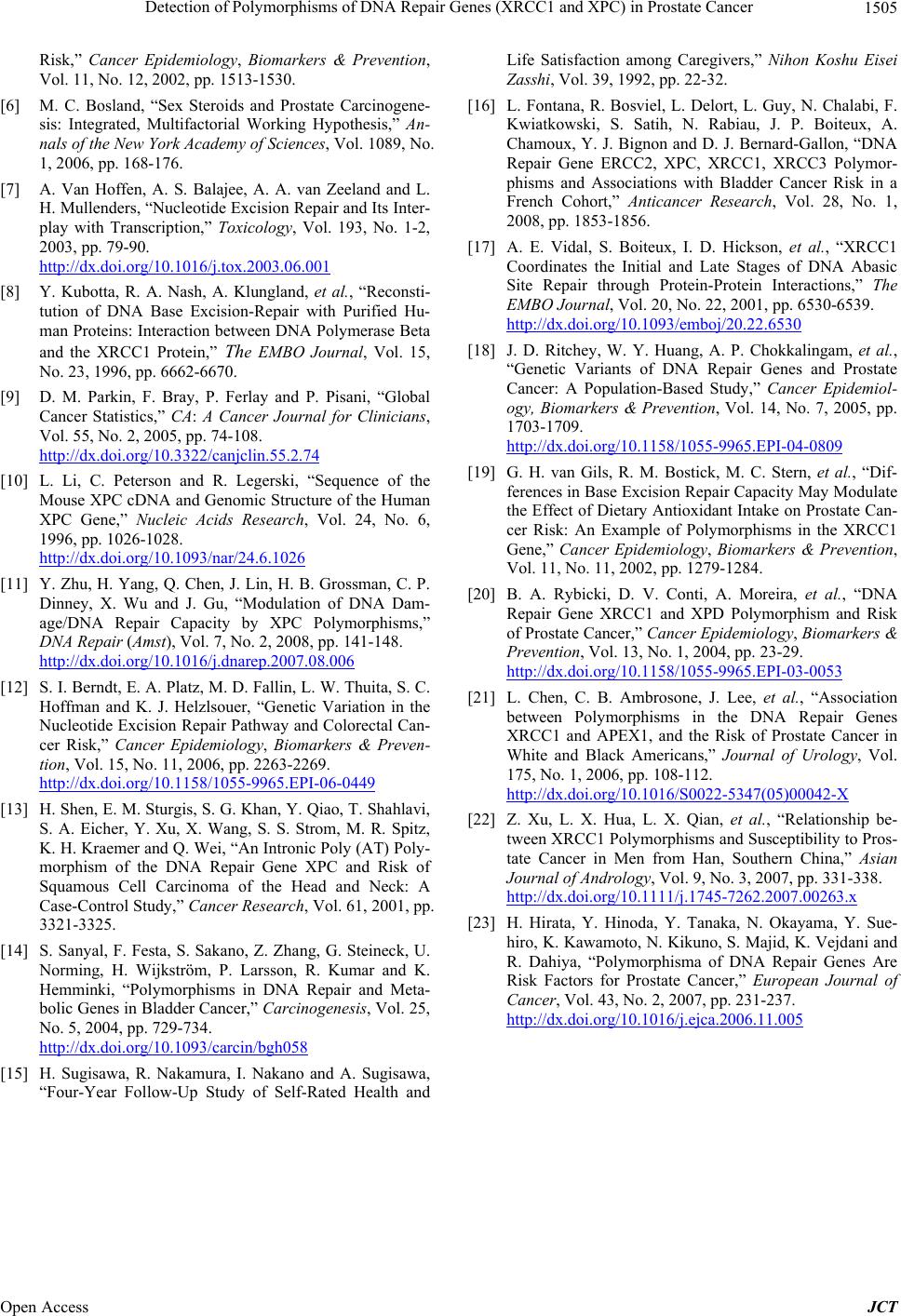
Detection of Polymorphisms of DNA Repair Genes (XRCC1 and XPC) in Prostate Cancer 1505
Risk,” Cancer Epidemiology, Biomarkers & Prevention,
Vol. 11, No. 12, 2002, pp. 1513-1530.
[6] M. C. Bosland, “Sex Steroids and Prostate Carcinogene-
sis: Integrated, Multifactorial Working Hypothesis,” An-
nals of the New York Academy of Sciences, Vol. 1089, No.
1, 2006, pp. 168-176.
[7] A. Van Hoffen, A. S. Balajee, A. A. van Zeeland and L.
H. Mullenders, “Nucleotide Excision Repair and Its Inter-
play with Transcription,” Toxicology, Vol. 193, No. 1-2,
2003, pp. 79-90.
http://dx.doi.org/10.1016/j.tox.2003.06.001
[8] Y. Kubotta, R. A. Nash, A. Klungland, et al., “Reconsti-
tution of DNA Base Excision-Repair with Purified Hu-
man Proteins: Interaction between DNA Polymerase Beta
and the XRCC1 Protein,” The EMBO Journal, Vol. 15,
No. 23, 1996, pp. 6662-6670.
[9] D. M. Parkin, F. Bray, P. Ferlay and P. Pisani, “Global
Cancer Statistics,” CA: A Cancer Journal for Clinicians,
Vol. 55, No. 2, 2005, pp. 74-108.
http://dx.doi.org/10.3322/canjclin.55.2.74
[10] L. Li, C. Peterson and R. Legerski, “Sequence of the
Mouse XPC cDNA and Genomic Structure of the Human
XPC Gene,” Nucleic Acids Research, Vol. 24, No. 6,
1996, pp. 1026-1028.
http://dx.doi.org/10.1093/nar/24.6.1026
[11] Y. Zhu, H. Yang, Q. Chen, J. Lin, H. B. Grossman, C. P.
Dinney, X. Wu and J. Gu, “Modulation of DNA Dam-
age/DNA Repair Capacity by XPC Polymorphisms,”
DNA Repair (Amst), Vol. 7, No. 2, 2008, pp. 141-148.
http://dx.doi.org/10.1016/j.dnarep.2007.08.006
[12] S. I. Berndt, E. A. Platz, M. D. Fallin, L. W. Thuita, S. C.
Hoffman and K. J. Helzlsouer, “Genetic Variation in the
Nucleotide Excision Repair Pathway and Colorectal Can-
cer Risk,” Cancer Epidemiology, Biomarkers & Preven-
tion, Vol. 15, No. 11, 2006, pp. 2263-2269.
http://dx.doi.org/10.1158/1055-9965.EPI-06-0449
[13] H. Shen, E. M. Sturgis, S. G. Khan, Y. Qiao, T. Shahlavi,
S. A. Eicher, Y. Xu, X. Wang, S. S. Strom, M. R. Spitz,
K. H. Kraemer and Q. Wei, “An Intronic Poly (AT) Poly-
morphism of the DNA Repair Gene XPC and Risk of
Squamous Cell Carcinoma of the Head and Neck: A
Case-Control Study,” Cancer Research, Vol. 61, 2001, pp.
3321-3325.
[14] S. Sanyal, F. Festa, S. Sakano, Z. Zhang, G. Steineck, U.
Norming, H. Wijkström, P. Larsson, R. Kumar and K.
Hemminki, “Polymorphisms in DNA Repair and Meta-
bolic Genes in Bladder Cancer,” Carcinogenesis, Vol. 25,
No. 5, 2004, pp. 729-734.
http://dx.doi.org/10.1093/carcin/bgh058
[15] H. Sugisawa, R. Nakamura, I. Nakano and A. Sugisawa,
“Four-Year Follow-Up Study of Self-Rated Health and
Life Satisfaction among Caregivers,” Nihon Koshu Eisei
Zasshi, Vol. 39, 1992, pp. 22-32.
[16] L. Fontana, R. Bosviel, L. Delort, L. Guy, N. Chalabi, F.
Kwiatkowski, S. Satih, N. Rabiau, J. P. Boiteux, A.
Chamoux, Y. J. Bignon and D. J. Bernard-Gallon, “DNA
Repair Gene ERCC2, XPC, XRCC1, XRCC3 Polymor-
phisms and Associations with Bladder Cancer Risk in a
French Cohort,” Anticancer Research, Vol. 28, No. 1,
2008, pp. 1853-1856.
[17] A. E. Vidal, S. Boiteux, I. D. Hickson, et al., “XRCC1
Coordinates the Initial and Late Stages of DNA Abasic
Site Repair through Protein-Protein Interactions,” The
EMBO Journal, Vol. 20, No. 22, 2001, pp. 6530-6539.
http://dx.doi.org/10.1093/emboj/20.22.6530
[18] J. D. Ritchey, W. Y. Huang, A. P. Chokkalingam, et al.,
“Genetic Variants of DNA Repair Genes and Prostate
Cancer: A Population-Based Study,” Cancer Epidemiol-
ogy, Biomarkers & Prevention, Vol. 14, No. 7, 2005, pp.
1703-1709.
http://dx.doi.org/10.1158/1055-9965.EPI-04-0809
[19] G. H. van Gils, R. M. Bostick, M. C. Stern, et al., “Dif-
ferences in Base Excision Repair Capacity May Modulate
the Effect of Dietary Antioxidant Intake on Prostate Can-
cer Risk: An Example of Polymorphisms in the XRCC1
Gene,” Cancer Epidemiology, Biomarkers & Prevention,
Vol. 11, No. 11, 2002, pp. 1279-1284.
[20] B. A. Rybicki, D. V. Conti, A. Moreira, et al., “DNA
Repair Gene XRCC1 and XPD Polymorphism and Risk
of Prostate Cancer,” Cancer Epidemiology, Biomarkers &
Prevention, Vol. 13, No. 1, 2004, pp. 23-29.
http://dx.doi.org/10.1158/1055-9965.EPI-03-0053
[21] L. Chen, C. B. Ambrosone, J. Lee, et al., “Association
between Polymorphisms in the DNA Repair Genes
XRCC1 and APEX1, and the Risk of Prostate Cancer in
White and Black Americans,” Journal of Urology, Vol.
175, No. 1, 2006, pp. 108-112.
http://dx.doi.org/10.1016/S0022-5347(05)00042-X
[22] Z. Xu, L. X. Hua, L. X. Qian, et al., “Relationship be-
tween XRCC1 Polymorphisms and Susceptibility to Pros-
tate Cancer in Men from Han, Southern China,” Asian
Journal of Andrology, Vol. 9, No. 3, 2007, pp. 331-338.
http://dx.doi.org/10.1111/j.1745-7262.2007.00263.x
[23] H. Hirata, Y. Hinoda, Y. Tanaka, N. Okayama, Y. Sue-
hiro, K. Kawamoto, N. Kikuno, S. Majid, K. Vejdani and
R. Dahiya, “Polymorphisma of DNA Repair Genes Are
Risk Factors for Prostate Cancer,” European Journal of
Cancer, Vol. 43, No. 2, 2007, pp. 231-237.
http://dx.doi.org/10.1016/j.ejca.2006.11.005
Open Access JCT